Multiplex live single-cell transcriptional analysis demarcates cellular functional heterogeneity
Figures
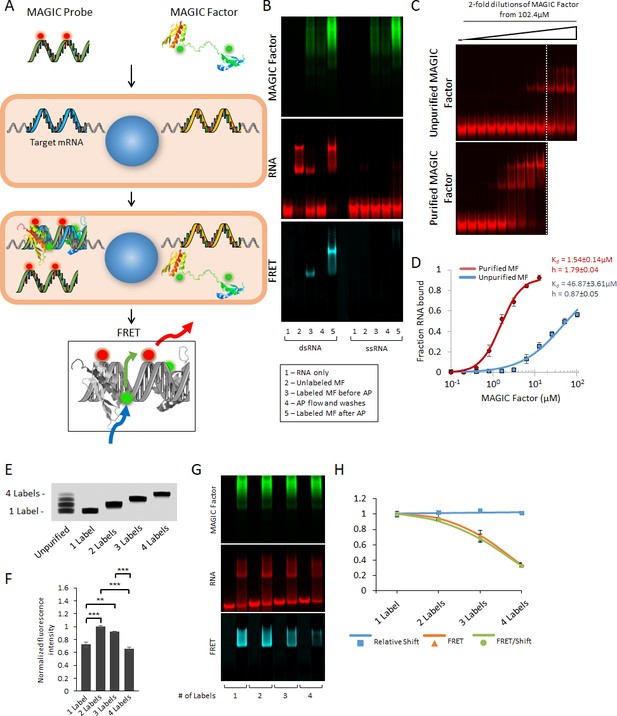
Strategy for the multiplex analysis of gene expression in individual living cells (MAGIC).
(A) MAGIC anti-sense RNA Probes are generated by in vitro transcription using T7 phage polymerase and fluorescently-labeled using aminoallyl-modified UTP nucleotides. MAGIC Factor is the recombinant dsRNA-binding domain of human protein kinase R that has been fluorescently labeled and affinity purified against dsRNA. Both the probe and the protein are delivered into living cells by transfection. Upon cellular delivery, MAGIC Probes hybridize to their target gene and generate an RNA-RNA duplex. This enables MAGIC Factor to bind to the newly formed dsRNA, thereby bringing the donor fluorophore on the protein to come into close contact with the acceptor fluorophore on the RNA probe and for FRET to occur (blue arrow: excitation of the donor; green arrow: energy transfer; red arrow: emission of the acceptor). Cells expressing the gene of interest are identified by assaying the FRET signal. (B) Affinity purification of fluorescent MAGIC Factor restores its binding affinity. MAGIC Factor was fluorescently-labeled with Alexa Fluor 488 and then reacted with dsRNA-coupled agarose beads to separate binding, functional protein from non-binding, non-functional protein. An electrophoretic mobility shift assay (EMSA) of dsRNA and ssRNA labeled with Alexa Fluor 647 and reacted with MAGIC Factor is shown in the RNA, protein and FRET channels. (C) Unpurified and purified MAGIC Factor were reacted at increasing concentrations with a fixed concentration of dsRNA (200 nM) and run on a native 12% polyacrylamide gel. The dashed lines represent the cutoff after which increasing concentrations of only unpurified MAGIC Factor were reacted with dsRNA. (D) Quantification of the binding affinity (represented as dissociation constant Kd) and Hill coefficients of unpurified and affinity purified, fluorescent MAGIC Factor (MF) from the EMSA in (C). Affinity purification of MAGIC Factor resulted in a 30-fold increase in binding affinity to dsRNA. (E) In vitro transcribed and fluorescently-labeled 20-mer RNA probe was gel purified to obtain one-, two-, three- and four-labeled RNA probes. (F) The fluorescence intensities of equimolar concentrations of purified probes with one to four labels were measured using a spectrophotometer. (G) 20-mer RNA probes with one to four labels were reacted with unlabeled sense probes to generate dsRNA. Representative EMSA with MAGIC Factor is shown in the RNA, protein and FRET channels. Note that the appearance of MAGIC Factor as multiple bands is likely due to the use of an NHS-ester dye to attach Alexa Fluor 488 to the protein. Dependent on the exact location of the fluorophore molecule, each single protein molecule likely runs differently on the native polyacrylamide gel. (H) The relative shift of fluorescent dsRNA, the FRET intensity of shifted dsRNA and the FRET/shift ratio for one to four labeled RNA probes were quantified from the EMSA in (G). Quantified data are shown as mean ± s.e.m. **p<0.01 and ***p<0.001.
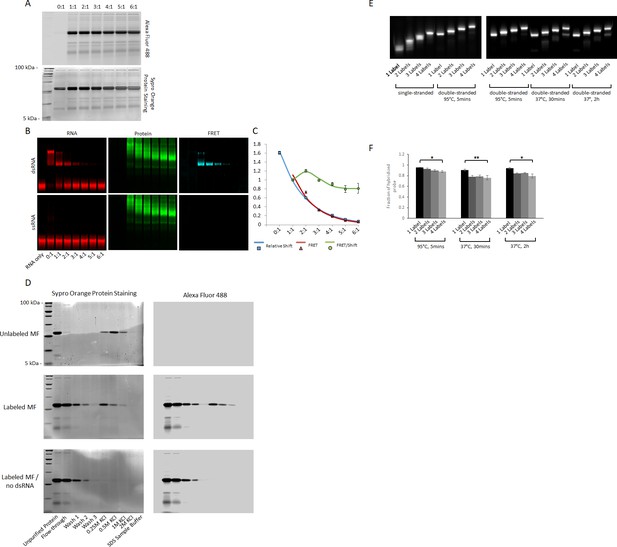
Optimization of fluorescence labeling of MAGIC Factor and MAGIC Probes.
(A) Labeling strategy of MAGIC Factor. MAGIC Factor was fluorescently labeled with increasing amounts of Alexa Fluor 488 SDP Ester and run on a 12% SDS-PAGE gel after purification by size-exclusion chromatography. The ratios refer to dye:protein molar ratios during fluorescent labeling. The control reaction was performed with DMSO instead of the dye. The same gel was imaged for native Alexa Fluor 488 fluorescence (top gel) and after staining all protein with Sypro Orange (bottom gel). (B) Electrophoretic Mobility Shift Assay (EMSA) of fluorescently labeled MAGIC Factor. Proteins from A were reacted with Alexa Fluor 647-labeled dsRNA and run on a native gel to visualize the binding affinities of the proteins. The gel was visualized in the RNA channel, protein channel and FRET channel. Note that the appearance of MAGIC Factor as multiple bands is likely due to the use of an NHS-ester dye to attach Alexa Fluor 488 to the protein. Dependent on the exact location of the fluorophore molecule, each single protein molecule likely runs differently on the native polyacrylamide gel. (C) Quantitative assessment of the EMSA with respect to the relative shift of the dsRNA, the corrected FRET (cFRET) intensity of shifted dsRNA and the cFRET/shift ratio. (D) Unlabeled and fluorescently labeled MAGIC Factor were affinity purified using dsRNA-coupled agarose beads. The proteins were reacted with the beads for 1 hr, washed three times with binding buffer and then gradually eluted with increasing concentrations of KCl. At the end, remaining proteins were eluted with 1x SDS-PAGE sample buffer and all samples run on a 12% SDS-PAGE gel. As a control experiment, fluorescently labeled MAGIC Factor was reacted with agarose beads in the absence of dsRNA. Gels were imaged for native Alexa Fluor 488 fluorescence (right gel) and after staining all protein (left gel). (E) Effect of degree of RNA fluorescent labeling on probe hybridization kinetics. Fluorescently labeled RNA probes were gel purified to obtain one to four labeled RNA probes. They were then reacted with unlabeled sense probes to generate dsRNA in TEN100 buffer (tris, EDTA, sodium chloride) at 95°C (positive control) or physiologic buffer resembling cytoplasmic ionic concentrations at 37°C for 30 min or 2 hr. ssRNA are shown as controls. Representative native 20% polyacrylamide gels are shown. (F) Quantification of RNA fluorescence intensities from (E). Quantified data are shown as mean ± s.e.m. *p<0.05 and **p<0.01.
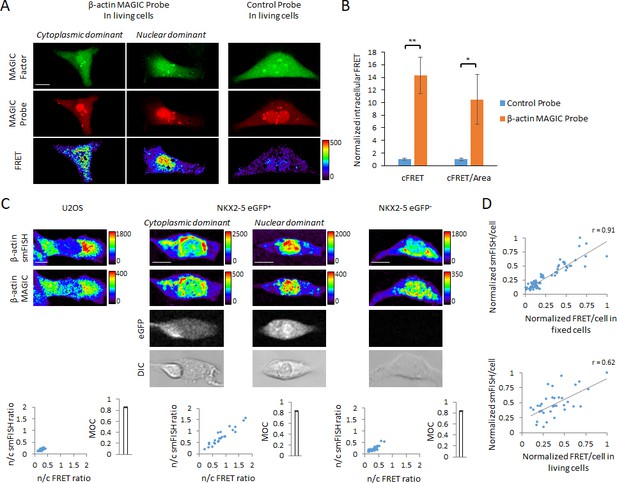
In situ visualization of transcripts in single living and fixed cells using MAGIC.
(A) A novel MAGIC Probe against the human β-actin mRNA or control probe were delivered into single living hPSC-CMs together with MAGIC Factor. Both probes were labeled with Alexa Fluor 647 and MAGIC Factor was labeled with Alexa Fluor 488. The cells were assessed by confocal microscopy and the FRET images corrected for spectral bleed-through from both donor and acceptor. The resulting FRET image is shown in pseudocolor-coding. We found two different phenotypes of the β-actin mRNA localization: cells with cytoplasmic distribution and cells with nuclear localization of the mRNA. (B) The FRET intensity of assessed single cells was quantified by removing spectral bleed-through from both donor and acceptor (corrected FRET, cFRET) and further normalizing to cell area (cFRET/Area). n ≥ 22 single cells per group. (C) U2OS cells, a pure population of cardiac myocytes (NKX2-5 eGFP+) and a non-cardiogenic cell population (NKX2-5 eGFP-) were fixed and subjected to simultaneous smFISH and MAGIC of the β-actin mRNA. The β-actin smFISH probes were labeled with Quasar 670, while MAGIC Factor was labeled with Alexa Fluor 488 and the β-actin MAGIC Probe was labeled with Alexa Fluor 546. From the smFISH and FRET images, the nuclear-to-cytoplasmic fluorescence intensity in each cell of each cell type was measured and the degree of co-localization quantified (Manders’ Overlap Coefficient, MOC). The cells were assessed by confocal microscopy and the FRET images corrected for spectral bleed-through from both donor and acceptor. The resulting FRET image is shown in pseudocolor-coding. n ≥ 23 single cells per group. (D) (Top) Normalized smFISH/cell versus normalized FRET/cell (from (C)) are plotted and show a robust positive correlation between both assays. Each point represents a single cell. (Bottom) MAGIC was performed on single living cells first and the same cells subjected to smFISH after fixation. Normalized smFISH/cell versus normalized FRET/cell are plotted and show that MAGIC can be used to infer to the level of mRNA expression in single living cells. Each point represents a single cell. Note that thresholded pixels are excluded from FRET images and thus appear as black signals. Further details on post-image processing are included in the Methods. Scale bars 25 μm. Quantified data are shown as mean ± s.e.m. *p<0.05 and **p<0.01.
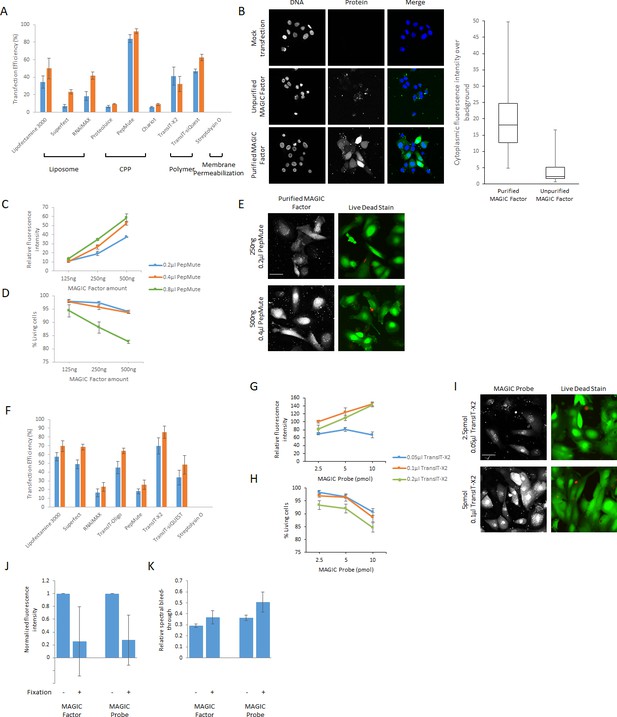
Optimization of cellular delivery of MAGIC Factor and MAGIC Probes.
(A) Cellular delivery of fluorescent MAGIC Factor. 125 ng fluorescent MAGIC Factor was delivered into hPSC-derived CMs seeded at a density of 50,000 cells/cm2 in a 96-well plate by a variety of commonly used delivery systems using liposomes, cell penetrating peptides (CPP), polymers and membrane permeabilization. Reagents were used at concentrations minimizing cell death. Lipofectamine 3000 0.2 μl and 0.4 μl, Superfect 1.25 μl and 2.5 μl, RNAiMAX 0.2 μl and 0.4 μl, ProteoJuice 0.2 μl and 0.4 μl, Pepmute 0.1 μl and 0.2 μl, Chariot 0.5 μl and 1 μl, TransIT-X2 0.05 μl and 0.1 μl, TransIT-siQUEST 0.05 μl and 0.1 μl, Streptolysin O 100 ng/μl and 1 μg/μl. Successful delivery was defined as the relative proportion of cells with a minimum two-fold increase in the cytoplasmic fluorescence signal over autofluorescence. Punctuate fluorescence signals were omitted from analysis. (B) hPSC-CMs were either mock transfected or transfected with unpurified or purified fluorescent MAGIC Factor. The cytoplasmic fluorescence intensity was then measured and is displayed relative to cytoplasmic background fluorescence based on mock transfected cells. Nuclei were counterstained with Hoechst dye. (C) 125 ng, 250 ng and 500 ng of fluorescent MAGIC Factor were delivered into hPSC-CMs using 0.2 μl, 0.4 μl or 0.8 μl of PepMute. The cytoplasmic fluorescence intensities were then measured and are displayed relative to cytoplasmic background fluorescence based on mock transfected cells. (D) The relative proportion of living cells from (C) was quantified using a live/dead assay after confocal imaging. (E) Representative images of cells from (C) and (D) transfected with 250 ng and 500 ng fluorescent MAGIC Factor with 0.2 μl and 0.4 μl PepMute, respectively, are shown. (F) Cellular Delivery of fluorescent MAGIC Probes. 2.5 pmol fluorescent MAGIC Probes were delivered into hPSC-derived CMs seeded at a density of 50,000 cells/cm2 in a 96-well plate by a variety of commonly used nucleic acid delivery systems. Reagents were used at concentrations minimizing cell death. Lipofectamine 3000 0.2 μl and 0.4 μl, Superfect 1.25 μl and 2.5 μl, RNAiMAX 0.2 μl and 0.4 μl, TransIT-Oligo 0.4 μl and 0.8 μl, Pepmute 0.1 μl and 0.2 μl, TransIT-X2 0.025 μl and 0.05 μl, TransIT-siQUEST 0.025 μl and 0.5 μl, Streptolysin O 100 ng/μl and 1 μg/μl. Successful delivery was defined as the relative proportion of cells with a minimum two-fold increase in the cytoplasmic fluorescence signal over autofluorescence. Punctuate fluorescence signals were omitted from analysis. (G) 2.5 pmol, 5 pmol and 10 pmol of fluorescent MAGIC Probe were delivered into hPSC-CMs using 0.05 μl, 0.1 μl or 0.2 μl of TransIT-X2. The cytoplasmic fluorescence intensities were then measured and are displayed relative to cytoplasmic background fluorescence based on mock transfected cells. (H) The relative proportion of living cells from (G) was quantified using a live/dead assay after confocal imaging. (I) Representative images of cells from (G) and (H) transfected with 2.5 pmol and 5 pmol fluorescent MF with 0.05 μl and 0.1 μl TransIT-X2, respectively, are shown. (J) hPSC-CMs were transfected with Alexa Fluor 488-labeled MAGIC Factor or Alexa Fluor 594-labeled MAGIC Probe. The same single cells were imaged before and after paraformaldehyde fixation and the fluorescence intensity of both constructs quantified. The fluorescence intensity in each single cell after fixation was normalized to the corresponding fluorescence intensity before fixation. (K) hPSC-CMs were transfected with Alexa Fluor 488-labeled MAGIC Factor or Alexa Fluor 594-labeled MAGIC Probe. The same single cells were imaged before and after paraformaldehyde fixation and the spectral bleed-through of both constructs into the FRET channel quantified. Scale bars 40 μm.
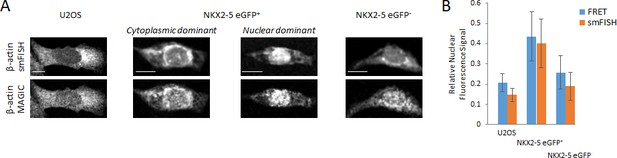
Cytoplasmic and nuclear localization of β-actin mRNA.
(A) Raw fluorescence images of images shown in Figure 2C are shown. (B) From the FRET and smFISH images in Figure 2C, the relative nuclear fluorescence signal in each cell was quantified. Scale bars 25 μm. Quantified data are shown as mean ± s.d.
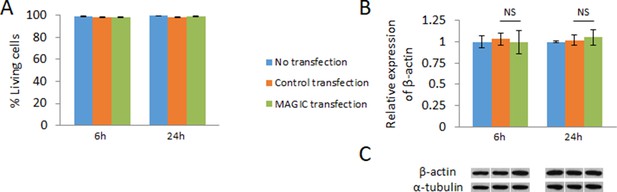
Effect of cellular delivery of MAGIC Factor and MAGIC probes on cell viability, and mRNA and protein levels.
(A) hPSC-CMs were not transfected, exposed to the transfection reagents only (control transfection) or co-delivered with MAGIC Factor and MAGIC Probe against the human β-actin mRNA. The viability of over 1200 living cells in each group was assessed after 6 hr and 24 hr of cell culture using a two-color fluorescence assay (n = 3 independent experiments). (B) hPSC-CMs were not transfected, exposed to the transfection reagents only (control transfection) or co-delivered with MAGIC Factor and MAGIC Probe against the human β-actin mRNA. Transcript levels of the β-actin gene were assessed after 6 hr and 24 hr of cell culture via quantitative PCR and normalized against the housekeeping gene GAPDH (n = 3 independent experiments). (C) hPSC-CMs were not transfected, exposed to the transfection reagents only (control transfection) or co-delivered with MAGIC Factor and MAGIC Probe against the human β-actin mRNA. Representative immunoblots of β-actin and the housekeeping protein α-tubulin of corresponding controls and experimental group are shown. Quantified data are shown as mean ± s.e.m. NS, not significant.
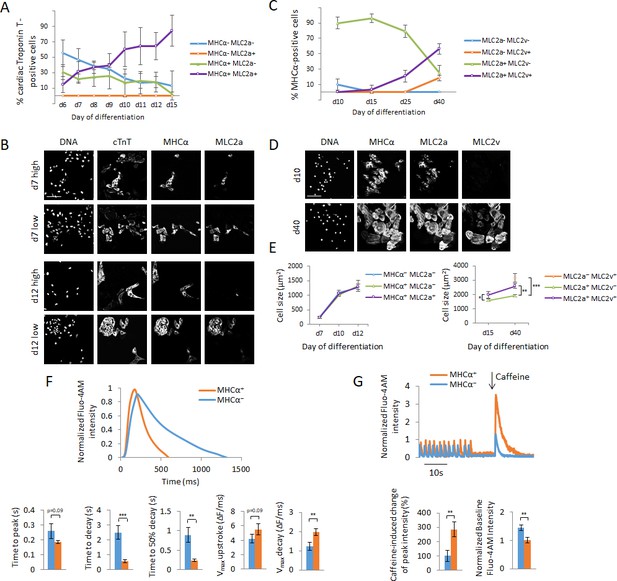
Cellular heterogeneity and functional assessment of hPSC-CMs using MAGIC.
(A) hPSC-CMs were quantified for their expression of myosin light chain 2a (MLC2a) and myosin heavy chain alpha (MHCα) between days 6 and 15 of hPSC differentiation by immunofluorescence staining. Cardiac myocytes were identified by their co-expression of cardiac troponin T (cTnT). Quantitative assessment of protein expression is shown for days 6–15 of hPSC differentiation. (B) The replicates in (A) displayed a high degree of batch-to-batch variability in the co-expression of cTnT, MHCα and MLC2a. Representative images of cells from two different batches of each day 7 and day 12 of hPSC differentiation with a low and high degree of cellular heterogeneity are shown. A low degree of heterogeneity results in more uniform cell populations with most cardiac myocytes expressing MHCα and MLC2a, whereas a high degree of heterogeneity results in more diverse cell populations with most cardiac myocytes differentially expressing MHCα and MLC2a. (C) hPSC-CMs were quantified for their expression of MLC2a and myosin light chain 2 v (MLC2v) between days 10 and 40 of hPSC differentiation by immunofluorescence staining. Cardiac myocytes were identified by their co-expression of MHCα. Quantitative assessment of protein expression is shown for days 10–40 of hPSC differentiation. (D) Representative images of days 10 and 40 of hPSC differentiation of (C). (E) Cell size differences of myocardial sublineages of (A) and (C) at various time points of cellular differentiation were quantified (n = 227 (left panel) and n = 190 (right panel)). (F) Assessment of calcium handling of hPSC-CMs differing in their expression of MHCα. Single living hPSC-CMs were analyzed for MHCα gene expression using MAGIC first (same fluorophore combination as in (F)) and then loaded with the calcium indicator Fluo-4 AM to analyze their spontaneous Ca2+ handling properties. Representative Ca2+ transients and quantitative Ca2+ kinetics of MHCα+ and MHCα- CMs are shown (n = 28 cells). (G) Assessment of calcium handling of hPSC-CMs differing in their expression of MHCα in response to caffeine. Single living hPSC-CMs were first analyzed for MHCα gene expression (same fluorophore combination as in (F)) and then loaded with the calcium indicator Fluo-4 AM. 10 mM of caffeine was added in calcium-free buffer during data acquisition. Representative Ca2+ transients and quantitative Ca2+ kinetics of MHCα+ and MHCα- CMs in response to caffeine are shown (n = 19 cells). Scale bars 100 μm. Quantified data are shown as mean ± s.e.m. *p<0.05, **p<0.01 and ***p<0.001.
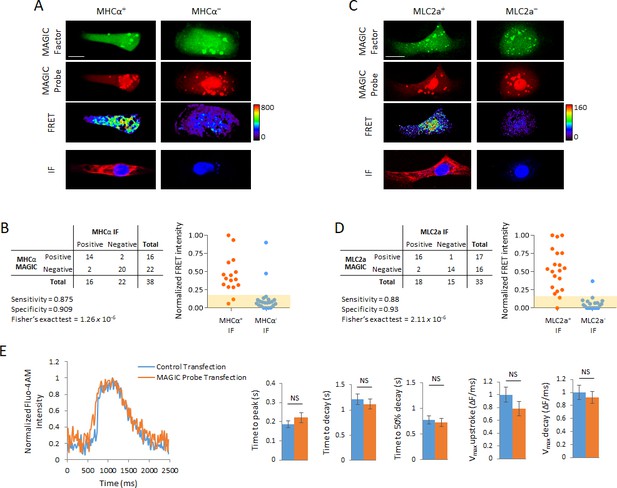
Development of MAGIC Probes against MHCα and MLC2a, and effect of MAGIC Probe transfection on calcium handling of hPSC-CMs.
(A) Single living hPSC-CMs were first analyzed for MHCα gene expression using a unique Alexa Fluor 647-labeled MAGIC Probe and Alexa Fluor 488-labeled MAGIC Factor, and then analyzed for MHCα protein expression by immunofluorescence staining (IF). FRET images were corrected for spectral bleed-through from both donor and acceptor and the resulting FRET image is shown in pseudocolor-coding. (B) Contingency table of analyzed hPSC-CMs from (A) (n = 38 cells) with distribution of normalized FRET signal intensities in cells stained for MHCα. The shaded area represents the threshold above which cells were considered positive for MHCα expression and contains 95% of FRET signal intensities in cells that received a control probe with no target in human cells. (C) Single living hPSC-CMs were first analyzed for MLC2a gene expression using a unique Alexa Fluor 594-labeled MAGIC Probe and Alexa Fluor 488-labeled MAGIC Factor, and then analyzed for MLC2a protein expression by immunofluorescence staining (IF). FRET images were corrected for spectral bleed-through from both donor and acceptor and the resulting FRET image is shown in pseudocolor-coding. (D) Contingency table of analyzed hPSC-CMs from (C) (n = 33 cells) with distribution of normalized FRET signal intensities in cells stained for MLC2a. The shaded area represents the threshold above which cells were considered positive for MLC2a expression and contains 95% of FRET signal intensities in cells that received a control probe with no target in human cells. (E) hPSC-CMs were exposed to the transfection reagents only (control transfection) or co-delivered with MAGIC Factor and MAGIC Probes against the MHCα, MLC2a and NKX2-5 mRNA, and their calcium handling assessed using the calcium indicator Fluo-4 AM. Representative Ca2+ transients and quantitative Ca2+ kinetics of single cells are shown (n = 50 cells). Note that thresholded pixels are excluded from FRET images (A,C) and thus appear as black signals. Further details on post-image processing are included in the Methods. Scale bars 20 μm. Quantified data are shown as mean ± s.e.m. NS, not significant.
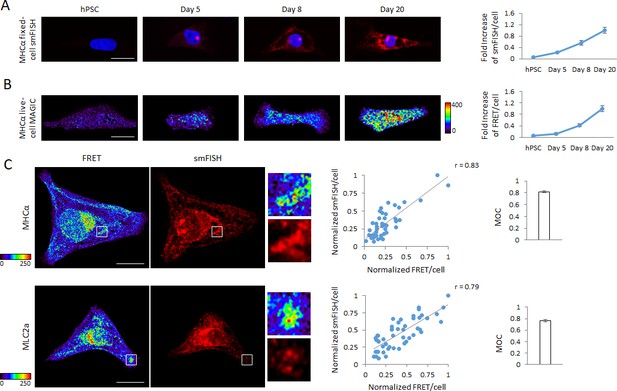
Detection of dynamic range of transcript levels using MAGIC.
(A) Undifferentiated hPSCs, differentiating hPSCs at day 5 of cardiac differentiation as well as differentiated cardiac myocytes at days 8 and 20 of differentiation were fixed and stained with smFISH probes against the MHCα mRNA. Normalized smFISH/cell is plotted and shows an increase of transcript levels as cellular differentiation of hPSCs proceeds (n = 164 cells). (B) Living undifferentiated hPSCs, differentiating hPSCs at day 5 of cardiac differentiation as well as differentiated cardiac myocytes at days 8 and 20 of differentiation were transfected with MAGIC Factor and MAGIC Probe against the MHCα mRNA. Single living cells were assessed for FRET by confocal microscopy and the FRET images were corrected for spectral bleed-through from both donor and acceptor. The resulting FRET image is shown in pseudocolor-coding. Normalized FRET/cell is plotted and shows an increase of transcript levels as cellular differentiation of hPSCs proceeds, demonstrating that MAGIC is able to detect a dynamic range of transcript levels (n = 65 cells). (C) hPSC-CMs were subjected to simultaneous smFISH and MAGIC of the MHCα (top panel) and MLC2a (bottom panel) mRNA. smFISH/cell and FRET/cell are plotted and show a robust positive correlation between both assays. Each point represents a single cell. From the smFISH and FRET images, the degree of co-localization was quantified (Manders’ Overlap Coefficient, MOC). The cells were assessed by confocal microscopy and the FRET images corrected for spectral bleed-through from both donor and acceptor. The resulting FRET image is shown in pseudocolor-coding. n ≥ 45 single cells per group. Note that thresholded pixels are excluded from FRET images and thus appear as black signals. Further details on post-image processing are included in the Materials and methods. Scale bars 25 μm (A,B) and 20 μm (C). Quantified data are shown as mean ± s.e.m.
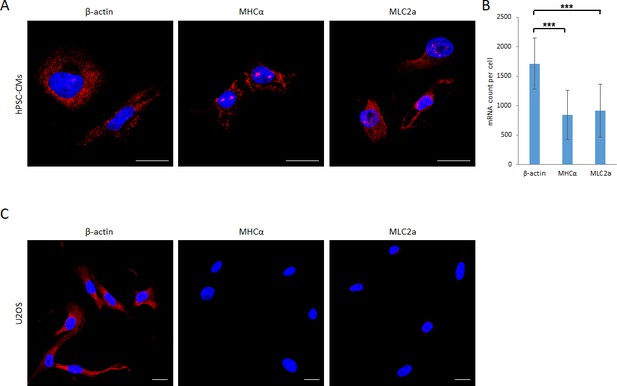
smFISH imaging of β-actin, MHCα and MLC2a mRNA in hPSC-CMs and U2OS cells.
(A) Images of hPSC-derived CMs stained with smFISH probes targeting the β-actin, MHCα and MLC2a mRNA are shown. (B) Transcript levels of β-actin, MHCα and MLC2a in single hPSC-CMs were quantified from images shown in (A) using Volocity software (n = 15 cells per group). (C) Images of U2OS cells stained with smFISH probes targeting the β-actin, MHCα and MLC2a mRNA are shown. Scale bars 25 μm. Quantified data are shown as mean ± s.d. ***p<0.001.
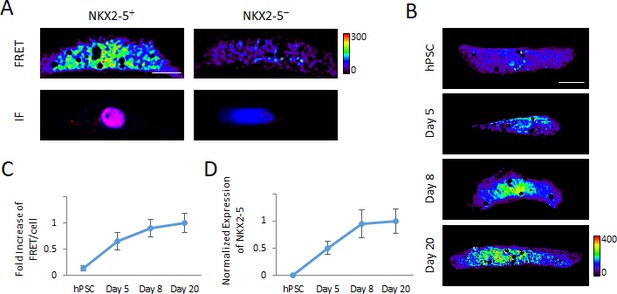
Detection of transcription factor mRNA using MAGIC.
(A) Single living hPSC-CMs were first analyzed for NKX2-5 gene expression using three unique Alexa Fluor 594-labeled MAGIC Probes and Alexa Fluor 488-labeled MAGIC Factor, and then analyzed for NKX2-5 protein expression by immunofluorescence staining (IF). FRET images were corrected for spectral bleed-through from both donor and acceptor and the resulting FRET image is shown in pseudocolor-coding. (B) Living undifferentiated hPSCs, differentiating hPSCs at day 5 of cardiac differentiation as well as differentiated cardiac myocytes at days 8 and 20 of differentiation were transfected with MAGIC Factor and MAGIC Probes against the NKX2-5 mRNA. Single living cells were assessed for FRET by confocal microscopy and the FRET images were corrected for spectral bleed-through from both donor and acceptor. The resulting FRET image is shown in pseudocolor-coding. (C) Normalized FRET/cell from the cells in (B) is plotted and shows an increase of transcript levels as cellular differentiation of hPSCs proceeds, demonstrating that MAGIC is able to detect a dynamic range of transcript levels of low-abundant transcription factors (n = 80 cells). (D) Transcript levels of the NKX2-5 gene in undifferentiated hPSCs, differentiating hPSCs at day 5 of cardiac differentiation as well as differentiated cardiac myocytes at days 8 and 20 of differentiation were assessed via quantitative PCR and normalized against the housekeeping gene GAPDH (n = 3 independent experiments). Note that thresholded pixels are excluded from FRET images and thus appear as black signals. Further details on post-image processing are included in the Methods. Scale bars 15 μm. Quantified data are shown as mean ± s.e.m.
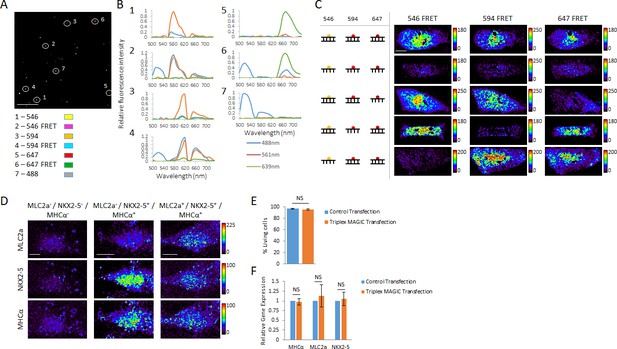
Multiplex spectral FRET imaging in single living cells.
(A) Individual lipocomplexes containing only RNA labeled with Alexa Fluor 488, 546, 594, 647, 488 and 546, 488 and 594 or 488 and 647 were visualized by spectral imaging, resolving four individually labeled RNA constructs and three RNA FRET constructs. Image shown is a pseudo-colored merge image of all seven RNA constructs after linear unmixing data processing. Circles highlight individual lipocomplexes containing one of each RNA construct. (B) The spectral profiles of the highlighted, individual lipocomplexes in (A) were plotted from spectral imaging data, validating multiplexing potential of spectral FRET imaging. Each construct was excited with 488 nm, 561 nm and 639 nm lasers and their spectral profile between 500 and 740 nm recorded. (C) 20-mer dsRNA or ssRNA probes with no target in human cells and fluorescently labeled with Alexa Fluor 546, 594 or 647 were co-delivered into living hPSC-CMs together with MAGIC Factor as indicated. Spectral imaging and linear unmixing were performed on single living cells. (D) Single living hPSC-CMs were analyzed for MLC2a, NKX2-5 and MHCα expression using Alexa Fluor 546-, Alexa Fluor 594- and Alexa Fluor 647-labeled probes, respectively. Spectral imaging and linear unmixing were performed to identify individual cell subpopulations. The resulting individual FRET images are displayed in pseudo-coloring. (E) hPSC-CMs were control transfected or co-delivered with MAGIC Factor and MAGIC Probes against the MHCα, MLC2a and NKX2-5 mRNA. The viability of living cells in each group was assessed after transfection using a two-color fluorescence assay (n = 3 independent experiments). (F) hPSC-CMs were control transfected or co-delivered with MAGIC Factor and MAGIC Probes against the MHCα, MLC2a and NKX2-5 mRNA. Transcript levels of the three genes were assessed after transfection via quantitative PCR and normalized against the housekeeping gene GAPDH (n = 3 independent experiments). Note that thresholded pixels are excluded from FRET images and thus appear as black signals. Further details on post-image processing are included in the Methods. Scale bars 4 μm (A) and 15 μm (C,D). Quantified data are shown as mean ± s.e.m. NS, not significant.
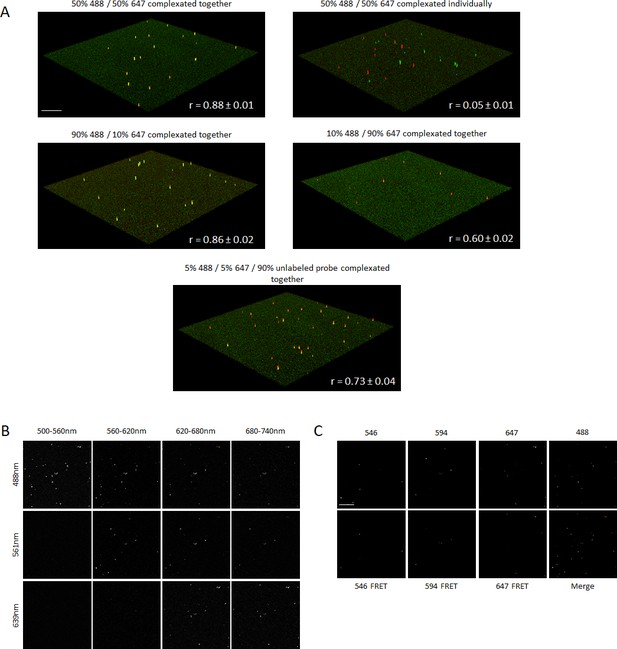
Spectral imaging of complexated fluorescent RNA probes.
(A) Co-localization of complexated fluorescent RNA probes. 20-mer RNA probes containing one Alexa Fluor 488 or 647 molecule were prepared and complexated with TransIT-Oligo transfection reagent to form fluorescent vesicles. The complexes were formed by incubation of both probes with the transfection reagent together or individually and at different ratios as described in figure. 3-D stacks of complexes were recorded by confocal microscopy and Pearson’s correlation coefficient r calculated to quantify the extent of co-localization of complexes. If complexated individually and sequentially loaded onto a plate, there was no significant co-localization. (B) Multiplex spectral FRET imaging using RNA lipocomplexes. Three RNA FRET pairs (Alexa Fluor 488–546, 488–594 and 488–647) and the corresponding single labeled constructs (Alexa Fluor 488, 546, 594 and 647) were complexated in individual lipocomplexes using TransIT-Oligo and loaded onto a fluorescence plate. Every lipocomplex contains only one type of fluorescent construct. We employed spectral imaging using laser scanning confocal microscope and imaged the spectral range 500–740 nm using 488 nm, 561 nm and 639 nm lasers in 10 nm windows. For visual clarity, we merged six windows. Images show 60 nm spectral windows excited by each laser of the same region of interest. (C) Linear unmixing and independent component analysis resulted in the acquisition of seven distinct images, showing each type of fluorescent construct individually. Scale bars 25 μm (A) and 4 μm (B,C).
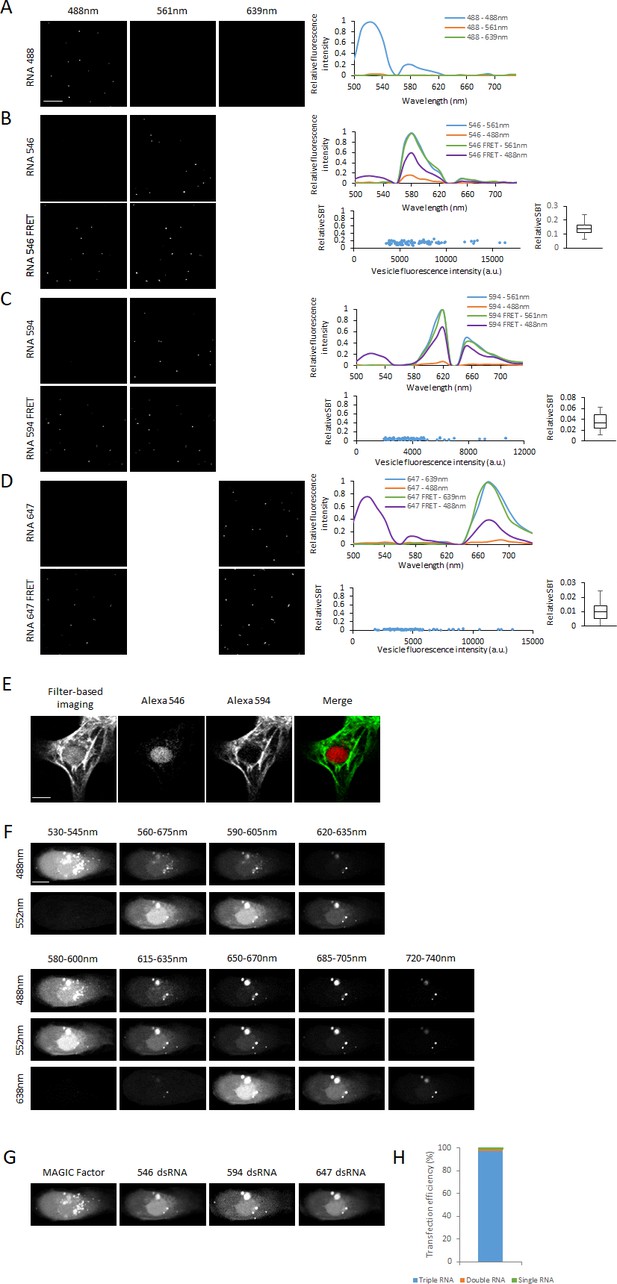
Spectral FRET imaging.
We complexated three RNA FRET pairs (Alexa Fluor 488–546, 488–594 and 488–647) and the corresponding single labeled constructs (Alexa Fluor 488, 546, 594 and 647) in lipocomplexes using TransIT-Oligo and loaded them onto a fluorescent plate. We employed spectral imaging using laser scanning confocal microscope and imaged the spectral range 500–740 nm using 488 nm, 561 nm and 639 nm lasers in 10 nm windows. Images show linearly unmixed images after spectral imaging. (A) RNA labeled with Alexa Fluor 488. From the spectral images, the corresponding spectra were acquired. (B) RNA labeled with Alexa Fluor 546 or Alexa Fluor 488 and 546. From the spectral images, the corresponding spectra were acquired and the relative spectral bleed-through (SBT) calculated. (C) RNA labeled with Alexa Fluor 594 or Alexa Fluor 488 and 594. From the spectral images, the corresponding spectra were acquired and the relative spectral bleed-through (SBT) calculated. (D) RNA labeled with Alexa Fluor 647 or Alexa Fluor 488 and 647. From the spectral images, the corresponding spectra were acquired and the relative spectral bleed-through (SBT) calculated. (E) hPSC-CMs were fixed and stained for NKX2-5 and MHCα using Alexa Fluor 546 and 594-labeled secondary antibodies, respectively. The cells were first imaged using standard filter-based microscopy, and then subjected to spectral imaging and linear unmixing to resolve the fluorescence signal from each fluorophore. (F) The cell co-delivered with MAGIC Factor and 20-mer dsRNA probes labeled with Alexa Fluor 546, 594 or 647 from Figure 4c is shown. Spectral imaging was performed using 488, 552 and 638 nm lasers from 530 to 635 nm (488 and 552 nm lasers) and from 580 to 740nm (488, 552 and 638nm lasers) in the indicated windows (G) Linear unmixing was performed to resolve MAGIC Factor and all three individual dsRNA probes. (H) Alexa Fluor 546, 594 or 647-labeled dsRNA probes were co-delivered into single living hPSC-CMs and spectral imaging and linear unmixing performed to resolve all three dsRNA probes. The fraction of cells containing all three probes, a combination of two probes or a single probe was quantified. Our co-delivery approach yielded a 97% transfection efficiency of all three probes together (n = 70 cells). Scale bar 4μm (A-D), 10μm (E) and 15μm (F,G).
Tables
Comparison of MAGIC with other live-cell mRNA imaging technologies.
https://doi.org/10.7554/eLife.49599.002Advantages | Disadvantages | |
---|---|---|
Nucleic Acid Probes | Most established approach | Complexity of probe design and cellular delivery |
Single-molecule sensitivity achievable | Need to screen many probes for specificity and sensitivity | |
Cell isolation via FACS | Probe sequestration and false-positive signals | |
Protein Probes | Single-molecule sensitivity | Genetic encoding of target RNA and reporter protein |
Study of RNA dynamics | Multiple binding sites necessary | |
Low sensitivity | ||
MAGIC | Imaging of transcription factors | Complexity of MAGIC Probe production |
Double detection with MAGIC Factor and Probes increases specificity | Efficient transfection of MAGIC Factor and Probes into the same cell required | |
Coupling with cell physiology assays |
Sequences of MAGIC probes and corresponding target sites.
https://doi.org/10.7554/eLife.49599.005MAGIC Probe Target | Sequence | Target site |
---|---|---|
Human β-actin | 5'-GGATAGCACAGCCTGGATAG-3' | 507-488 |
Human myosin heavy chain α (MHCα) | 5'-GGCACCAATGTCACGGCTCT-3' | 5864-5845 |
Human myosin light chain 2a (MLC2a) | 5’-GGCCTGCTTGGTGGCTGCCA-3’ | 66-47 |
Human NKX2-5, Probe 1 | 5’-GGCTGCGCTGCTGCTGTTCC-3’ | 308-289 |
Human NKX2-5, Probe 2 | 5’-GGACGTGAGTTTCAGCACGC-3’ | 766-747 |
Human NKX2-5, Probe 3 | 5’-GCGTTATAACCGTAGGGATT-3’ | 984-965 |
Control Probe | 5'-GGATCGTGACTAGATCGTCA-3' | |
Labeled ssRNA for EMSAs | 5'-GGATGAGTCACTGCCTAGCC-3' | |
Unlabeled ssRNA complementary to probe above | 5'-GGCTAGGCAGTGACTCATCC-3' | |
Labeled ssRNA for multiplex spectral FRET | 5'-GGAAGTAGCACAGTCCAGAC-3' | |
Unlabeled ssRNA for affinity purification, strand 1 | 5'-GAGTCCTTCCACGATAGACC-3' | |
Unlabeled ssRNA for affinity purification, strand 2 | 5'-GGTCTATCGTGGAAGGACTC-3' |
Expected expression levels of MHCα, MLC2a and MLC2v in atrial and ventricular hPSC-CMs during early vs. late differentiation (Kamakura et al., 2013; van den Berg et al., 2015).
https://doi.org/10.7554/eLife.49599.012atrial | ventricular | |||
---|---|---|---|---|
early | late | early | late | |
MHCα | + | +++ | + | +++ |
MLC2a | + | +++ | + | ++ |
MLC2v | - | - | - | +++ |
-
Table 3—source data 1
Summary of quantified data.
- https://doi.org/10.7554/eLife.49599.013
-
Table 3—source data 2
Fluorescence intensity quantification of quantified confocal microscopy data for each channel (MAGIC Factor, MAGIC Probe, FRET).
- https://doi.org/10.7554/eLife.49599.014
Reagent type (species) or resource | Designation | Source or reference | Identifiers | Additional information |
---|---|---|---|---|
Cell line (H. sapiens) | H7 | Harvard University | RRID:CVCL_9772 | |
Cell line (H. sapiens) | HUES-9 | Harvard University | RRID:CVCL_0057 | |
Cell line (H. sapiens) | NKX2-5eGFP/w | Dr. Stanley, Monash University, Australia | ||
Cell line (H. sapiens) | U2OS | This study | RRID:CVCL_0042 | Cell line can be obtained from our lab upon request |
Antibody | Anti-MHCalpha (mouse monoclonal) | R and D Systems | clone 940344 | 1:200 |
Antibody | Anti-MLC2a (mouse monoclonal) | Synaptic Systems | clone 56F5 | 1:200 |
Antibody | Anti-MLC2v (rabbit monoclonal) | Proteintech | Cat#: 10906–1-AP | 1:200 |
Antibody | Anti-Troponin T (mouse monoclonal) | R and D Systems | clone 200805 | 1:1000 |
Antibody | Anti-Nkx2.5 (goat monoclonal) | R and D Systems | Cat#: AF2444 | 1:400 |
Additional files
-
Transparent reporting form
- https://doi.org/10.7554/eLife.49599.021