Ubiquitous macropinocytosis in anthozoans
Figures
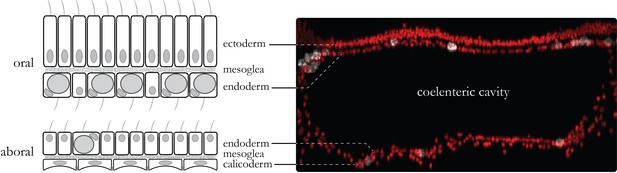
Coenosarc anatomy.
The tissue connecting individual polyps is composed of two tissues. The oral tissue is composed of the oral ectoderm (in contact with the sea water) and the oral endoderm (which contains Symbiodiniaceae in most cells) separated by the acellular mesoglea. The aboral tissue is composed of the aboral endoderm (which contains Symbiodiniaceae in some cells) and the calicoblastic ectoderm (responsible for the formation of the skeleton) separated by the mesoglea. Tissue layers have their basal side contacting the mesoglea whereas motile cilia and septate junctions mark their apical side. In between the two endodermal layers lies the coelenteric cavity which carries most of the internal fluids.
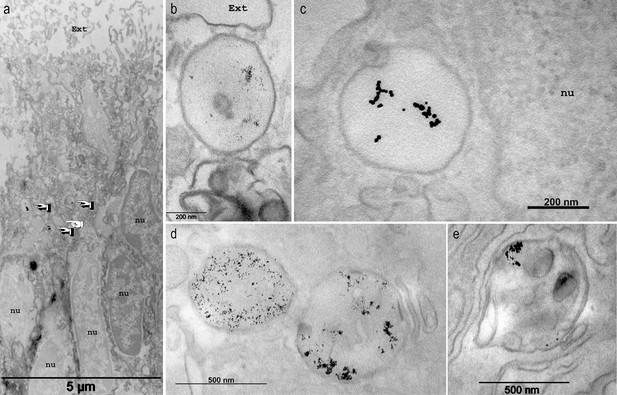
Endocytosis of gold dextran-coated nanoparticle by large vesicles.
Microcolonies were incubated in sea water complemented with Gold dextran coated nanoparticles of 3 nm (a,b,d,e) or 10 nm (c) in diameter and imaged with transmission electron microscopy in the ectoderm (a–b) or the endoderm (c–e). Samples here were not contrasted with OsO4. In (a) gold nanoparticles are detected in 3 out of the four vesicles (finger pointing) present in the tissue layer, but not in other parts of the cytoplasm. Higher magnifications (b–e) clearly delineate the large vesicles containing the nanoparticles. Of note, multivesicular body endosomes containing the nanoparticles (d, vesicle on the right, and e) were also observed. Ext, external sea water medium; nu, nucleus.
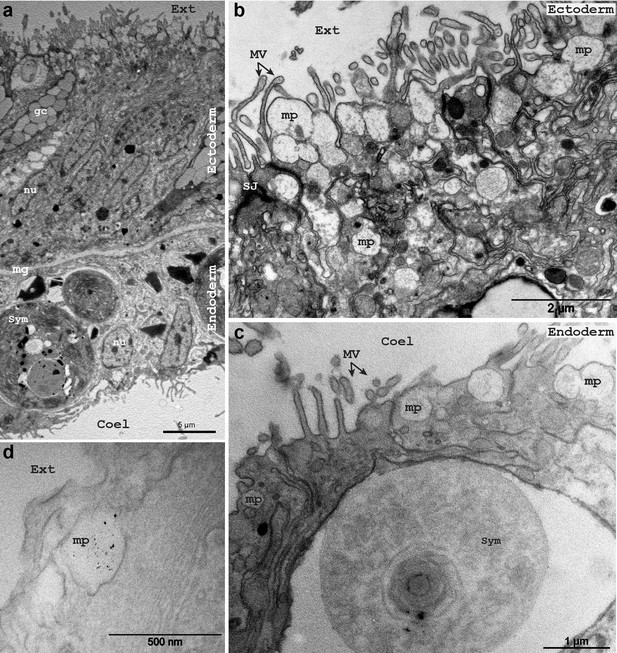
Macropinosomes formation.
Transmission electron microscopy photographs of Stylophora pistillata with (a–c) or without (d) osmium post-fixation step. In (d), the sample was incubated with 3 nm gold nanoparticles. The apical side of the ectoderm (a,b,d) and the endoderm (a,c) show large vesicle formation, typical of macropinocytic processes. Note that on the oral ectoderm apical side, the forming macropinosomes appears loaded with mucus. Ext, external sea water medium; Coel, coelenteric cavity; mp, macropinosome; Sym, symbiont; MV, microvillis; nu, nucleus; mg, mesoglea; gc, gland cell; SJ, septate junction.
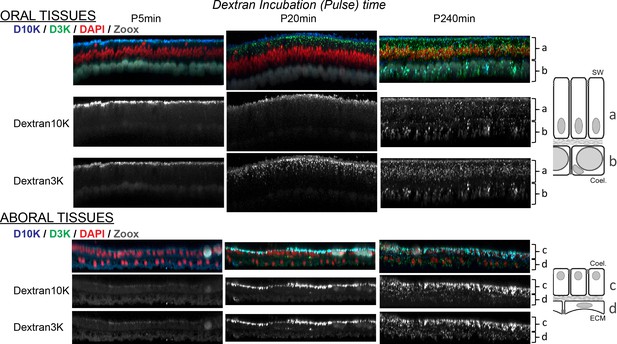
Kinetic of dextran uptake by Stylophora pistillata.
Incremental time length of Dextran 3K and 10K incubation shows gradual penetration of dextrans inside the tissues. Note that this figure only shows 5, 20, and 240 min’ incubation times, see Figure 3—figure supplement 3 for complementary time points. All images correspond to y-projections of Z stacks acquired through the oral (top panel) and the aboral tissues (bottom panel). The different tissue layers, depicted besides the photos as a,b,c,d, correspond to the oral ectoderm, the oral endoderm, the aboral endoderm, and the calicoblastic ectoderm respectively (SW: sea water; coel.: coelenteron; ECM: Extracellular Calcifying Medium). Dextran 3K and 10K individual channel acquisitions are shown in black and white, merged are shown in color: Dextran 10 KDa (D10K) in blue, Dextran 3 KDa (D3K) in green, DAPI (nuclei) in red and chlorophyll autofluorescence from the Symbiodiniaceae present in the endodermal tissue layers in gray. Each photograph represents 144 µm wide and 9 µm depth tissues.
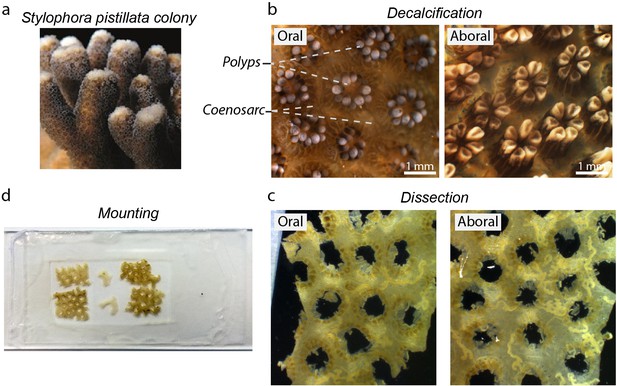
Sample processing.
A portion of a branch (microcolony) of a Stylophora pistillata colony (a) was fixed and decalcified in paraformaldehyde and EDTA based solutions, respectively, enabling visualization of both oral and aboral tissues (b). Polyps were further removed with micro scissors. The resulting coenosarc (c), which connects individual polyps, was cut into two pieces and mounted on a microscope slide with one piece having its oral tissue facing the coverslip and the other with its aboral tissue facing the coverslip (d); notice the mesenteries easily observable from the aboral side.
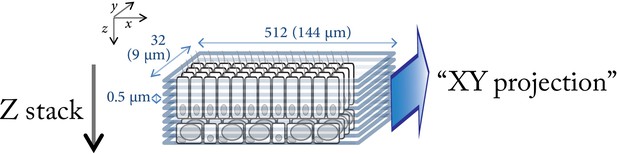
Imaging strategy.
Along the z axis, serial sections of 9 × 144 µm at 0.5 µm intervals are acquired through either the oral or the aboral tissues. Then, the z stack (60–100 µm deep) is projected along the x axis using the option ‘3D projection’ / ‘x projection = 90°’ in the ‘process’ menu of the LasAF software (Leica SP5). This projection represents the 9 µm thick cross-section of the imaged tissue layers.
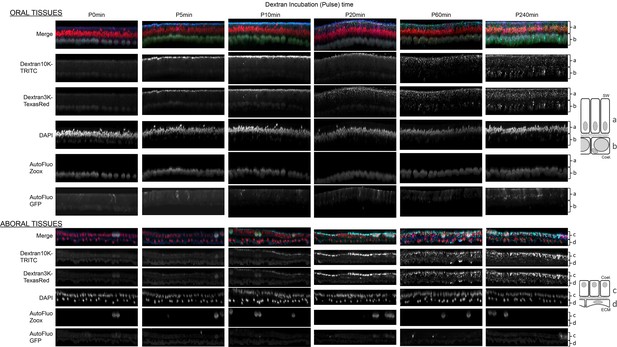
Incremental pulse (0, 5, 10, 20, 60, 240 min incubations) lengths of Dextran 3K and 10K show the Dextrans’ gradual penetration inside the tissues.
Images correspond to y-projections of Z-stacks acquired through the oral (top panel) and the aboral tissues (bottom panel). The different tissue layers, depicted beside the photos as a,b,c,d, correspond to the oral ectoderm, the oral endoderm, the aboral endoderm, and the calicoblastic ectoderm, respectively. Individual channel acquisitions (as described on the left side of the photographs) are shown in black and white, merged are shown in color: Dextran 10 KDa in blue, Dextran 3 KDa in green, DAPI (nuclei) in red, Symbiodiniaceae (endodermal tissue layers) autofluorescence in gray, and GFP potential autofluorescence in cyan.
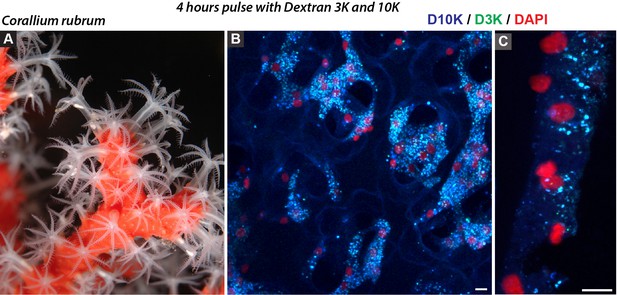
Probable macropinocytosis in Corallium rubrum.
Amongst Anthozoa, Octocorallia is the sister group to Hexacorallia. We investigated the octocorallian Corallium rubrum (or Mediterranean red coral, (A) for macropinocytosis. Red corals have a relatively different anatomy than reef-building corals in the sense that the mesoglea makes the gross of the tissues whereas the analog of the coelenteron corresponds to a ramified network of ‘vessels’ called vascular canals. After 4 hr incubation with the dextrans 3K and 10K, the large majority of the cells (centered by their nucleus in red) displayed labeled cytoplasm (B) with marked large vesicles, typical of macropinocytosis (C). Note that macropinosomes labeled with both D3K and D10K appear as merged color blue and green. Scale bar = 5 µm.
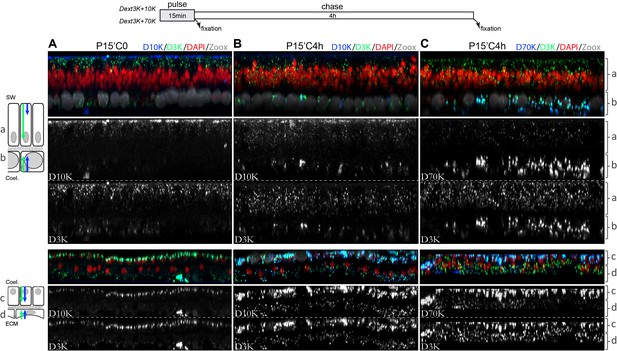
Cellular progression of the dextran uptake.
Dextran pulse of 15 min followed by 4 hr chase. Microcolonies were incubated for 15 min in sea water supplemented with D3K and D10K and fixed (A), or pulsed with D3K and D10K (B) or D3K and D70K (C) and left for an additional 4 hr in sea water before fixation. Tissues and labelling legends are as in Figure 2. Note that in all tissues, dextrans appear to follow an apical to basal progression inside the cells. Contrary to the other cell layers, the oral ectoderm have preferential endocytosis toward smaller dextran molecules (D3K >> D10K>D70K). Each photograph represents 144 µm wide and 9 µm depth tissues.
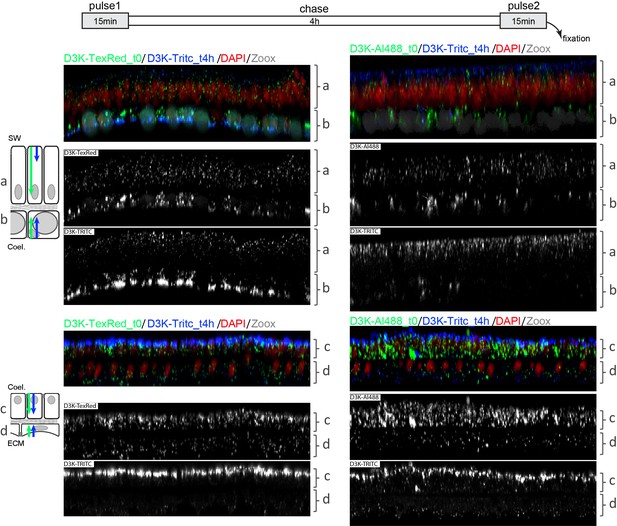
Apical to basal endocytosis: two 15 min pulses separated by a 4 hr chase.
Microcolonies were first incubated for 15 min with D3K either coupled with Texas-red (left panel) or Alexa 488 (right panel), chased in sea water for 4 hr to let the dextran progress through the epithelial cells’ cytoplasms, and then incubated for another 15 min with D3K coupled with TRITC. Regardless of the cell layer, the second pulsed dextran was always found at the apical face of the tissues whereas the first pulsed dextran was visible throughout the cells’ cytoplasms. Each photograph represents 144 µm wide and 9 µm depth tissues.
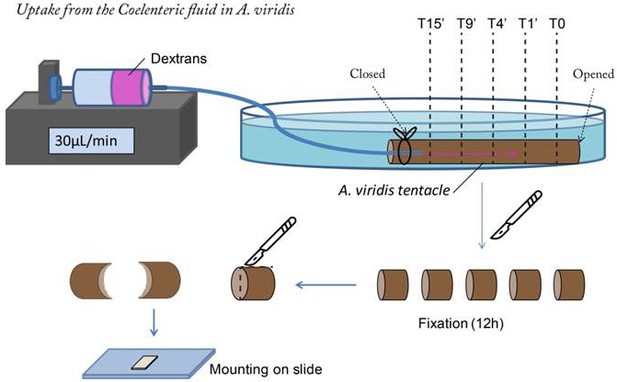
Dextran uptake from the Coelenteron of the sea anemone Anemonia viridis tentacles.
A tentacle was mounted as a semi-opened small bag similar to the experiment in Bénazet-Tambutté et al. (1996b). A 50 ml syringe containing 150 ng/ml D3K and 250 ng/ml D10K solubilized in sea water was set on a syringe pump with a constant flow of 30 µl per minute. This syringe was connected to one freshly isolated A. viridis tentacle (approx. 5 cm long) via a 1.5 mm (diameter) plastic tube and sealed at the tentacle’s proximal end with a silk yarn. The tentacle was bathing in a petri dish filled with sea water. Once the dextran solution started to leak out of the tentacle’s distal end, a portion of few millimeter long was sectioned after 1, 4, 9, and 15 min, and was immediately fixed on ice. A control section (T0) was cut before the onset of the syringe flow through. After fixation, the A. viridis tentacle pieces were opened by a longitudinal section, and mounted on a microscope slide with the endoderm facing the cover glass.
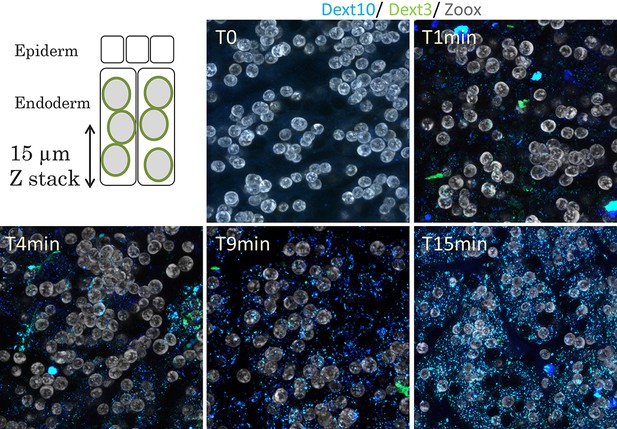
Dextran uptake from the Coelenteron of the sea anemone.
For each time points, a confocal Z stack encompassing the first 15 µm of the endoderm (from the coelenteric/apical side) was acquired. After only 1 min, the first dextran labeling was detected as dots around 500 nm diameter punctuating the cells’ cytoplasm. With longer incubations’ time, the number of dextran dots increased proportionally. Hence dextrans were macropinocytosed from the coelenteric cavity of the tentacle from the apical side of the endoderm. Dextran 10 KDa in blue, Dextran 3 KDa in green, Symbiodiniaceae in gray.
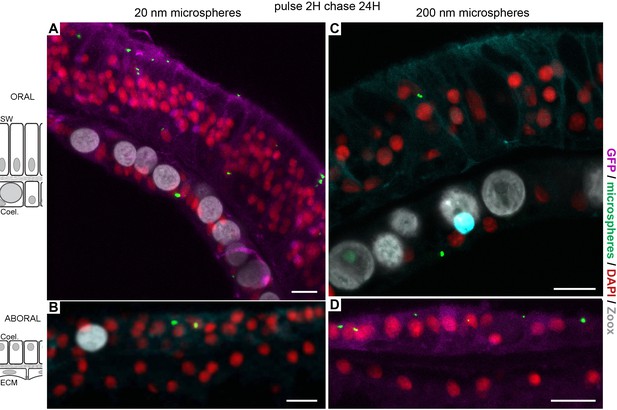
Latex beads paracellular diffusion and macropinocytosis.
microcolonies were incubated with 20 nm and 200 nm latex beads in order to challenge (i) the paracellular barrier and (ii) dextran specific endocytosis. Incubations of Fluorescent latex beads for 2 hr followed by a chase of 1 day show that the three tissue layers (A, oral ectoderm; B, oral endoderm; C, aboral endoderm; D, calicoblastic ectoderm) in contact with the medium could engulf particles of 20 nm (A,B) and 200 nm (C,D) in diameter. Thus, after passing via the polyps’ mouths (data not shown), beads enter the coelenteric cavity and are endocytosed by the endoderm tissue layers, although with less efficiency than dextran molecules. Alternatively, they are directly acquired from the sea water by the oral ectoderm. However, in the calicoblastic cells, neither 20 nm nor 200 nm beads were detected. The paracellular barrier made by septate junctions prevented the access to the extra calcifying medium, and thus the calicoblastic cells. Scale bar = 10 µm.
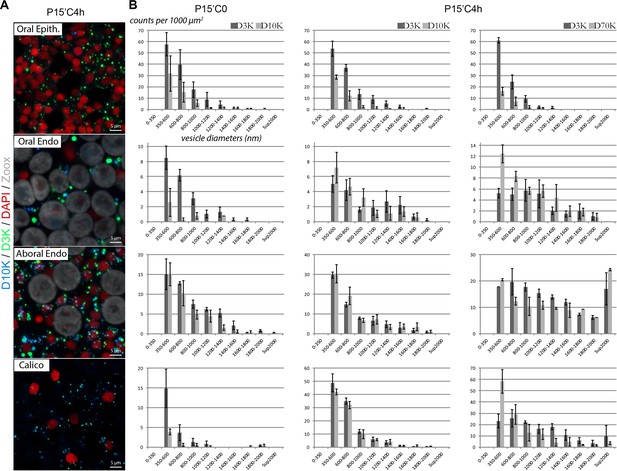
Quantitative analysis of the dextran endocytic vesicles.
High magnification Z stack sections encompassing the different individual tissue layers (from top to bottom: oral ectoderm, oral endoderm, aboral endoderm, calicoblastic ectoderm) were acquired after the same pulse-chase experiments than in Figure 3A) Representative Z projections for each tissue after D3K/D10K 15 min pulse/4 hr chase (legends as in Figure 3). Note the variable relative content of the co-localizing dextrans from one vesicle to another. (B) For each experiment, the diameters (nm) of the dextran vesicles were measured then ranked according to their size distribution and their counts were normalized per square units (detailed in Appendix 1). Note that the very large vesicles (D > 2 µm) observed in the aboral ectoderm with D3K-D70K were in most cases due to the piling of smaller vesicles that were recognized by the imageJ program as one single artefactual vesicle after Z-stack projection.
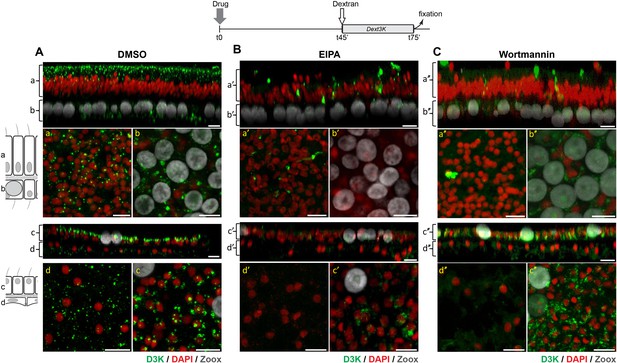
EIPA and Wortmannin inhibits macropinocytosis in corals.
Microcolonies were first incubated in the presence of DMSO (control) (A), 100 µM EIPA (B) or 1 µM Wortmannin (C) for 45 min. Then D3K was added to the incubations and pulsed for an additional 30 min before being fixed and processed for confocal analysis. Top (a,b, a’,b’) and bottom (c,d,c’,d’) panels corresponds to oral and aboral tissue analyses, respectively. In each panel, the top picture is a y projection of a Z-stack through the tissue layers as in Figure 3, and the two bottom pictures correspond to a Z projection of a stack embracing individual tissue layers at higher magnification as in Figure 7A. Although DMSO has no effect on dextran endocytosis, EIPA and Wortmannin strongly impaired dextran internalization. Note that for the Wortmannin experiment, PMTs were increased to pick up the low signals of dextran intake. Scale bars = 10 µm.
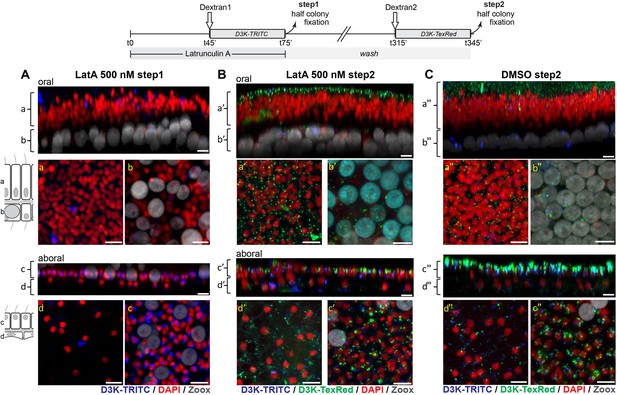
Actin is required for coral macropinocytosis: at T = 0, microcolonies were incubated in the presence of 500 (panels A and B) Latrunculin or control DMSO (panel C).
At T = 45 min., D3K-TRITC was added to the incubation. At T = 75 min. colonies were cut into two halves, one half was fixed (step1) the other half was set to normal seawater. At T = 315 min., the second halves of the colonies were incubated with D3K-TexRed for another 30 min. before fixation (step2). Panels and legends are as in Figure 7. At 500 nM, micropinocytosis (intake of D3K-TRITC) is clearly inhibited in the oral and calicoblastic cell layers whereas only slightly impaired in the aboral endoderm (step1, Panel A) (note that macropinocytosis in the aboral endoderm is completely inhibitied at 1000 nM LatA, Figure 9—figure supplement 1). However, after 4 hr washing, inhibition was removed as macropinocytic activity (intake of D3K-TexRed) was restored (PanelB). Scale bars = 10 µm.
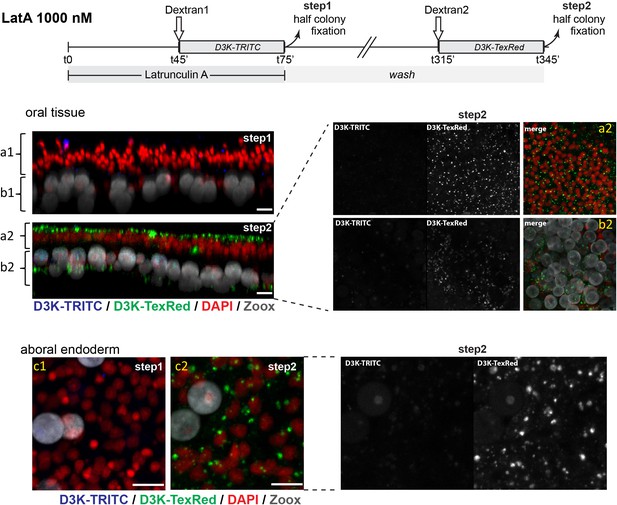
Latrunculin A (LatA) at 1000 reversibly inhibits macropinocytosis in the aboral endoderm.
In parallel to the experiment carried out with 500 nM of Latruculin A (Figure 9), we carried out a two steps inhibition experiment at 1000 nM on the same colony. The first step consisted in a 30 min. Dextran pulse (D3K-TRITC) in the presence of the inhibitor (LatA/1000 nM), the 2nd step of a second 30 min. Dextran pulse (D3K-TexRed) after 4 hr washing in regular seawater. Like for the experiment at 500 nM, in the oral tissue (oral ectoderm (a1, a2) and endoderm (b1,b2)), the first inhibitory step led to almost complete abolition of the D3K-TRITC intake, but then macropinocytosis of the D3K-TexRed was restored after 4H washing. In the aboral tissues, the same was observed for the aboral endoderm, implying that macropinocytosis was reversibly inhibited at 1000 nM LatA. However, at this concentration, the integrity of calicoblastic cell layer was compromised (not shown). Black and White images correspond the individual channel (TRITC or TexRed) acquired at same PMTs.
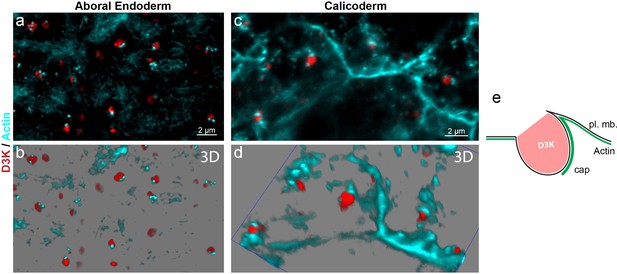
F-actin is linked to macropinosome formation.
A microcolony grown on coverslip was incubated for 15 min. with D3K, fixed and labeled with phalloidin. Representative confocal Z-stacks (a, c) and 3D reconstruction snapshots (b, d) of the aboral endodermal (a, b) and calicoblastic (c, d) cell layers are shown; Phalloidin (F-actin) in cyan and D3K in red (see also Videos 3–5). For the vast majority of the forming macropinosomes, as visualized by D3K labeling, an intracellular ‘cap’ of actin is associated (e), illustrating the participation of F-Actin to macropinosome formation.
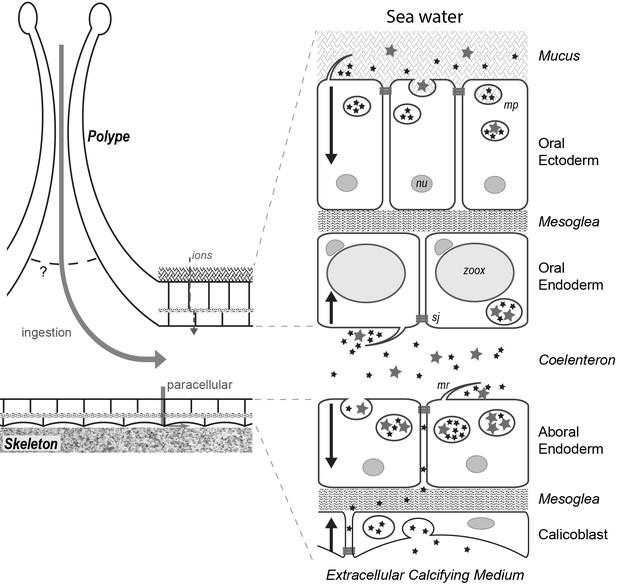
Fluids/particles flow from the sea water to the cell cytoplasm.
Schematic representation of the movement of particles in the different tissue layers of a coral (see text). Stars represent particles of two different sizes (inferior and superior to 20 nm); mp, macropinosome; mr, membrane ruffling; nu; nucleus; sj, septate junction (apical side); zoox, Symbiodiniaceae.
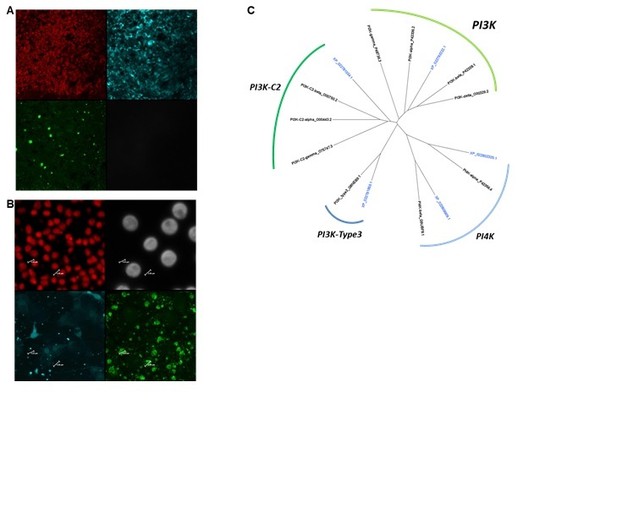
LY294002 treatment triggers unexpected secondary effect and phylogenetic comparison of the PI3K family in Stylophora pistillata vs human.
(A and B) Confocal Z-stack of Stylophora incubated in LY294002. Red=Dapi; Green=dextra; gray=Zoox, and Cyan is the GFP chanel. A and B correspond to the oral ectoderm and the aboral endoderm, respectively. Note that in the oral ectoderm, the cell layer is filled with GFP-like signal that is never seen under other conditions. In B, the GFP-like signal is less strong (although different than controls), and the vesicles of dextran are abnormally large (4-5 μm in diameter). However, based on the side effect of LY294002, this assay was not validated. (C) PhyML phylogeny of the PI3K families in human (names in black) and in Stylophora (names in blue). In Stylophora, there is only one cognate PI3K homolog (4 in human). The other sequences are PI3K-related members (i.e. PI3K-C2, PI3K-type3 and PI4K).
Videos
Macropinosomes.
A branch of Stylophora pistillata Coral was incubated for 30 min with D3K and D10K, then fixed. The video shows a 3D reconstruction (LAS-AF) of a Z-stack encompassing the aboral endoderm. Symbiodiniaceae (10 µm diameter) are in gray, nuclei (3 µm diameter) in red, texasRed-D3K in green and TRITC-D10K in blue. Note the macropinosomes labeled with D3K and D10K which appear as merged color.
Paracellular diffusion.
The video correspond to screencasts of the LasAM program graphical interface (Leica) displaying the time-laps acquisition (xzyt) in the video mode. The laterally grown microcolony was set in an incubation chamber and analyzed by inverted confocal microscopy from beneath, at the edge of the microcolony where there are gaps in-between the growing crystals. Time laps imaging was recorded (single Z section, 5sec/image). After 3 min 30 s of recording, texasRed-D3K was added to the medium. D3K is in green (TexasRed detected 615–625 nm); Symbiodiniaceae (10 µm diameter) are in gray. Note the timer display at the bottom right corner of the screen. Within tens of seconds, the paracellular labeling is apparent.
Actin and macropinosomes, large view.
A laterally grown microcolony was incubated in Dextran for 15 min, fixed and shortly decalcified before F-actin labeling with phalloidin. The video corresponds to a 3D reconstruction (LAS-X) of a Z-stack encompassing the aboral endoderm. The video describes a large view of the cellular F-actin network and the process of macropinocytosis (D3K uptake) in the endoderm. TexasRed-D3K in red (macropinosomes), F-actin in cyan and nuclei in blue.
Actin and macropinosomes, zoom into the endoderm.
Same as in Video 3 (endoderm), showing a closer view, without nuclei. Note the cap of actin (cyan) always associated with the forming macropinosome (red).
Actin and macropinosomes, zoom into the calicoblastic cells.
The video corresponds to a 3D reconstruction (LAS-X) of a Z-stack encompassing 3 cells of the calicoblastic ectoderm. Like for the endoderm, note the cap of actin (cyan) always associated with the forming macropinosome (red).
Tables
Tracers’ endocytosis.
Tracer | Size (nm) | Charge* | Oral ecto. | Oral endo. | Aboral endo. | Calico | Para-cellular |
---|---|---|---|---|---|---|---|
dextran 3 KDa Alexa488 | 1.3 | (-) | ++ | ++ | ++ | ++ | + |
dextran 3 KDa TexRed | 1.3 | (+/-) | ++ | ++ | ++ | ++ | + |
dextran 10 KDa TRITC | 2.3 | (-) | + | ++ | ++ | ++ | + |
dextran 70 KDa TRITC | 6 | (-) | - | ++ | ++ | ++ | + |
gold-dextan-coated-3nm | 3 | (?) | + | + | + | na | na |
gold-dextan-coated-10nm | 10 | (?) | - | + | + | na | na |
microsphere-20nm | 20 | (--) | + | + | + | - | - |
microsphere-200nm | 200 | (--) | + | + | + | -- | - |
-
*charge: (?) unknown, (+/-) zwitterionic; (-) anionic; (--) highly negatively charged.
Dye detection.
Fluo | Laser ex (nm) | Em max (nm) | Detection range (nm) |
---|---|---|---|
DAPI | 405 | 461 | 430–470 |
Symbiodiniaceae* | 405 | 680–740 | |
GFP* | 488 | 500–530 | |
Alexa 488 | 488 | 495 | 500–530 |
TRITC | 543 | 577 | 557–585 |
TexRed | 543 | 613 | 607–630 |
FluoSphere | 543 | 605 | 600–640 |
-
*autofluorescence.