Disruption of entire Cables2 locus leads to embryonic lethality by diminished Rps21 gene expression and enhanced p53 pathway
Abstract
In vivo function of CDK5 and Abl enzyme substrate 2 (Cables2), belonging to the Cables protein family, is unknown. Here, we found that targeted disruption of the entire Cables2 locus (Cables2d) caused growth retardation and enhanced apoptosis at the gastrulation stage and then induced embryonic lethality in mice. Comparative transcriptome analysis revealed disruption of Cables2, 50% down-regulation of Rps21 abutting on the Cables2 locus, and up-regulation of p53-target genes in Cables2d gastrulas. We further revealed the lethality phenotype in Rps21-deleted mice and unexpectedly, the exon 1-deleted Cables2 mice survived. Interestingly, chimeric mice derived from Cables2d ESCs carrying exogenous Cables2 and tetraploid wild-type embryo overcame gastrulation. These results suggest that the diminished expression of Rps21 and the completed lack of Cables2 expression are intricately involved in the embryonic lethality via the p53 pathway. This study sheds light on the importance of Cables2 locus in mouse embryonic development.
Introduction
The mouse embryo at the blastocyst stage, consists of two layers: the outer trophectoderm and the inner cell mass (ICM). The ICM is characterized as pluripotent stem cells, from which the epiblast and primitive endoderm are derived (Chazaud et al., 2006; Evans and Kaufman, 1981; Rossant et al., 2003). Epiblast cells give rise to all cell types of the fetal tissues. The primitive endoderm produces the visceral endoderm (VE) extraembryonic yolk sac lining. Following implantation, Wnt, Nodal, and bone morphogenetic protein (BMP) signaling pathways are essential and coordinately control formation of the proximal–distal (P–D) axis during the egg cylinder stage and the subsequent conversion of this axis into the anterior–posterior (A–P) axis early in gastrulation (reviewed in Arkell and Tam, 2012; Robertson, 2014; Shen, 2007; Ten Berge et al., 2008; Wang et al., 2012; Winnier et al., 1995). Nodal and Wnt activity levels are dependent upon the BMP pathway interactions (Robertson, 2014; Tam and Loebel, 2007). The epiblast undergoes rapid cell proliferation and is sensitive to DNA damage, which may lead to p53-dependent checkpoint activation and result in apoptosis (Heyer et al., 2000; Kojima et al., 2014; O'Farrell et al., 2004). The primitive streak is formed by regional regulated expression of lineage-specific markers including Brachyury (T) and Wnt3 via the Wnt/β-catenin pathway, to initiate the gastrulation stage. (Rivera-Pérez and Magnuson, 2005; Tam et al., 2006). While some murine axis and gastrulation signaling events are known, many other processes remain undiscovered.
Cdk5 and Abl enzyme substrate 1 (Cables1, also known as ik3-1) founded the Cables protein family, each member of which has a C-terminal cyclin box-like domain. Cables1 physically interacts with cyclin-dependent kinase 2 (Cdk2), Cdk3, Cdk5, and c-Abl molecules, and is phosphorylated by Cdk3, Cdk5, and c-Abl (Matsuoka et al., 2000; Yamochi et al., 2001; Zukerberg et al., 2000). Furthermore, in primary cortical neurons, c-abl phosphorylation of Cables1 augments tyrosine phosphorylation of Cdk5 to promote neurite outgrowth (Zukerberg et al., 2000). Cables1 also functions as a bridging factor linking Robo-associated Abl and the N-cadherin-associated β-catenin complex in chick neural retina cells (Rhee et al., 2007). Notably, Cables1-null mice show increased cellular proliferation resulting in endometrial hyperplasia, colon cancer, and oocyte development (Kirley et al., 2005a; Lee et al., 2007; Zukerberg et al., 2004). Additionally, the corpus callosum development in mice may rely on a dominantly acting, truncated version of Cables1 (Mizuno et al., 2014). During zebrafish development, Cables1 is required for early neural differentiation and its loss subsequently causes apoptosis of brain tissue and behavioral abnormalities (Groeneweg et al., 2011). Zebrafish have only one Cables gene (Cables1), whereas the mouse and human genomes contain the paralogue, Cables2 (also known as ik3-2). The C-terminal cyclin-box-like region of Cables1 and Cables2 share a high degree of similarity. Cables2 has been shown to physically associate with Cdk3, Cdk5, and c-Abl (Sato et al., 2002). Moreover, forced expression of Cables2 induced apoptotic cell death in both a p53-dependent manner and a p53-independent manner in vitro (Matsuoka et al., 2003). In human, CABLES2 was recently found as a new susceptibility gene or tumor suppressor in colorectal cancer (Guo et al., 2021). Adult mouse tissues including the brain, testis, and ovary express Cables2 (Hasan et al., 2021), however, the role of this protein in vivo is unknown.
Therefore, in this study, we generated Cables2d mice with completely deleted entire locus (Cables2d) to elucidate Cables2 function in vivo. The data reveal the necessity of the Cables2 locus for early embryonic development in mice and introduce the novel relationship of Cables2 to Rps21, the p53 and Wnt/β-catenin pathways.
Results
Expression of Cables2 during early mouse development
Cables2 is widely expressed at equivalent levels in mouse tissues, including the brain, heart, muscle, thymus, spleen, kidney, liver, stomach, testis, skin, and lung (Sato et al., 2002). We first investigated the expression of Cables2 in mouse embryonic stem cells (ESCs), blastocysts, and embryos at E7.5 by reverse transcription polymerase chain reaction (RT-PCR). The results indicated that Cables2 was expressed in all three stages of early development (Figure 1A). To confirm Cables2 gene expression in mouse embryogenesis, localization of Cables2 mRNA expression was examined in embryos by WISH (Figure 1B-F). The data for the whole embryo and transverse sections showed that Cables2 was expressed at E6.5 (Figure 1B,C). Cables2 was detected in both extra- and embryonic parts at E7.5 (Figure 1D) and strongly expressed in the allantois and in regions caudal to the heart at E8.5 (Figure 1E). At E9.5, widespread expression of Cables2 was observed in embryo and extraembryonic tissues, including the yolk sac (Figure 1F). Overall, these data indicate that Cables2 is widely expressed during early development, including throughout gastrulation in mouse embryos.
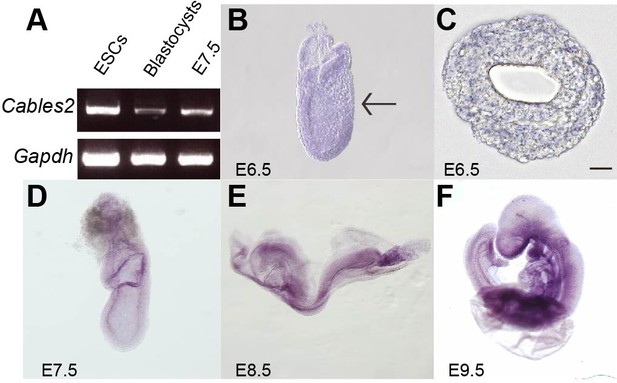
Cables2 expression during early mouse embryo development.
(A) Cables2 gene expression was examined by RT-PCR with ESCs, blastocyst, and E7.5 embryo samples. Gapdh was used as an internal positive control. (B–F) Wild-type embryos from E6.5 to E9.5 were examined by in situ hybridization with a Cables2 probe. The whole embryo expressed Cables2 at E6.5 (B). The black arrow indicates the position of the transverse section shown in (C). Scale bars, 20 μm.
Early embryonic lethality in Cables2d model
Cables2d mice were generated to investigate the physiological role of Cables2 in vivo. Cables2 heterozygous mice were produced using conventional aggregation with Cables2-targeted ESC clones purchased from KOMP (Knockout Mouse Programme). The entire Cables2 allele was deleted with VelociGene KOMP design, a targeting strategy of IMPC (International Mouse Phenotyping Consortium) (Figure 2A). Interestingly, no homozygous Cables2d mice were observed following intercrossing heterozygous mice; however, the heterozygotes were viable and fertile (Table 1, Figure 2—figure supplement 1A). Embryos were collected and genotyped at various times during embryonic development to identify the critical point at which Cables2 is essential for survival (Table 1). Homozygous Cables2 mutant mice were detected in Mendelian ratios at E6.5–E9.5 but no homozygous embryos were observed at or beyond E12.5, indicating that Cables2d mice die and are resorbed between E9.5 and 12.5 (Table 1, Figure 2—figure supplement 1B). All the Cables2d embryos collected at E7.5–9.5 were considerably smaller than their wild-type littermates and did not progress beyond the wild-type early-mid-gastrula cylindrical morphology (Table 1, Figure 2B–E). Considerably small Cables2d/d embryos had barely progressed beyond E8.5 (Figure 2C). Notably, at E7.5 homozygous mutant embryos resembled E6.5 wild-type embryos, in both morphology and size, when the primitive streak is just beginning to form (Figure 2F,G). Histological analyses confirmed that pre-streak stage (E6.0) Cables2d/d embryos were structurally normal, exhibiting a normal-sized epiblast, extraembryonic ectoderm, and primitive endoderm (Figure 2H,I). These results suggested that Cables2 full deletion causes growth and patterning arrest in gastrulation accompanied by post-gastrulation embryonic lethality.
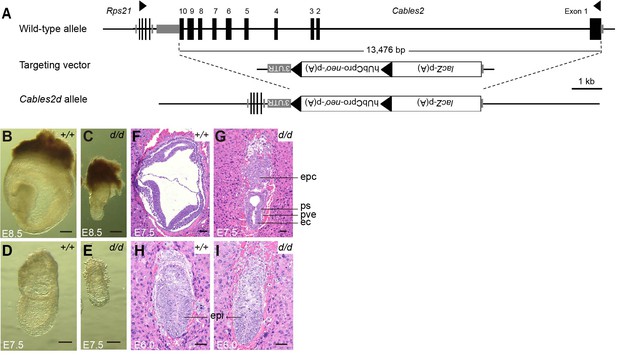
Morphological and histological analyses of Cables2d embryos at early stages of development.
(A) Following VelociGene’s KOMP design, the entire protein coding sequence of the target gene was deleted by homologous recombination in C57BL/6N ESCs, therefore, the full Cables2 allele was null (Cables2d allele). Embryos were collected and genotyped at E8.5 (B, C) and E7.5 (D, E). Histological analysis was on HE-stained sections. Wild-type and Cables2d mutant embryos were embedded in paraffin and stained at E7.5 (F, G) and E6.0 (H, I). Epc: ectoplacental cone, ps: primitive streak; pve: posterior visceral endoderm; ec: ectoderm; epi: epiblast. Scale bars, 100 μm (B–E), 50 μm (F–I).
Survival rate and Mendelian ratio of Cables2-mutant embryos.
Embryonic days (E) | Total number of embryos | Genotypes | ||
---|---|---|---|---|
+/+ | +/- | -/- | ||
E6.5 | 437 | 132 (30.2)* | 221 (50.6) | 80 (18.3) |
E7.5 | 70 | 18 (25.7) | 32 (45.7) | 20† (28.6) |
E8.5 | 21 | 9 (42.9) | 9 (42.9) | 3† (14.3) |
E9.5 | 18 | 7 (38.9) | 7 (38.9) | 4† (22.2) |
E12.5 | 6 | 2 (33.3) | 4 (66.7) | 0 (0) |
Adult | 90 | 24 (26.7) | 66 (73.3) | 0 (0) |
-
* Number of embryos (percentage), † Abnormal phenotype.
Developmental defect in Cables2d embryos at the onset of gastrulation
We further analyzed the expression of gastrulation markers at E6.5. Prior to gastrulation, T transcripts are first detected as a ring in extra-embryonic ectoderm and then in the posterior epiblast before the appearance of the primitive streak. (Perea-Gomez et al., 2004; Rivera-Pérez and Magnuson, 2005). T transcripts therefore serve as a marker of the transition from the P-D to A-P axis. At E6.5, Cables2d/d embryos exhibited T expression as a band in the extra-embryonic ectoderm, indicating that the PS marker presented in the posterior epiblast even before morphological appearance of the primitive streak (Figure 3A,B). To confirm the PS formation in Cables2d/d embryos, we further investigated the expression of Fgf8, a member of the fibroblast growth factor family expressed in the PS (Crossley and Martin, 1995), and found that Fgf8 was also appeared in mutant embryos at E6.5 (Figure 3E,F). Notably, T and Fgf8 were decreased expression relative to wild-type embryos, suggesting the PS formation marker was initiated, however, with the low intensity in Cables2d/d embryos.
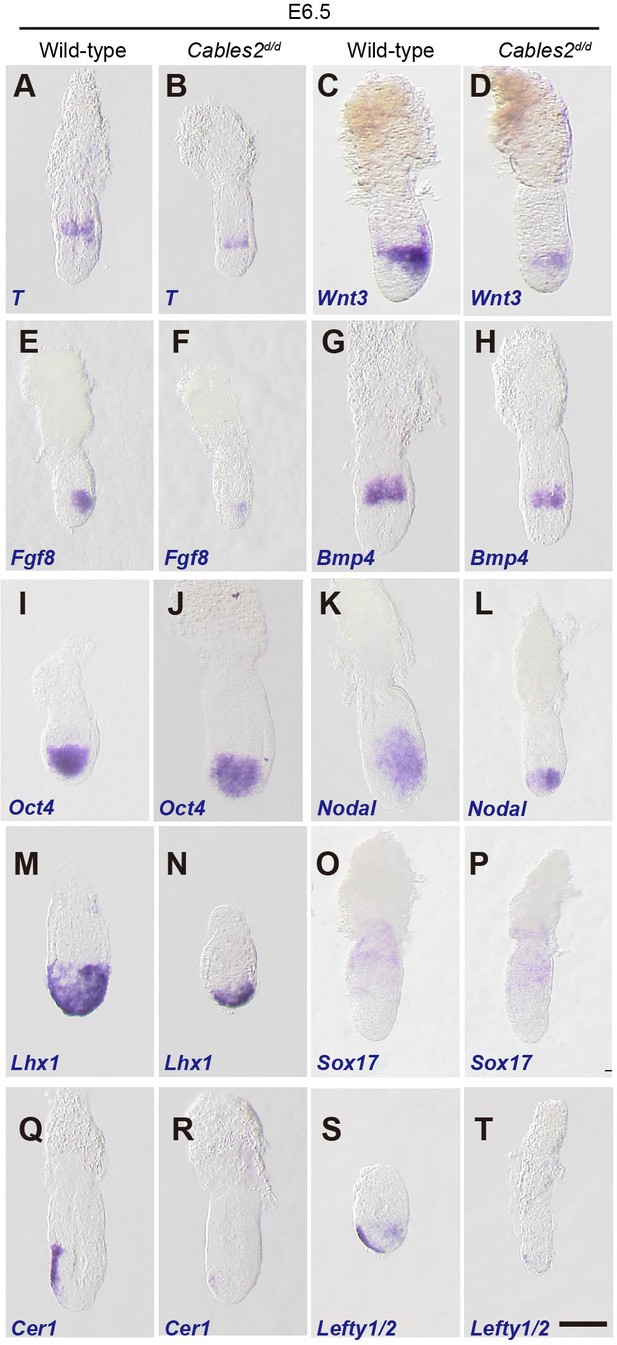
Expression of gastrulation markers in Cables2d embryos.
(A–T) All embryos were collected, genotyped, and used for WISH at E6.5. Several key gastrulation markers were examined using both wild-type and Cables2d embryos: T (n = 5), Wnt3 (n = 5), Fgf8 (n = 3), Bmp4 (n = 3), Oct4 (n = 4), Nodal (n = 4), Lhx1 (n = 3), Sox17 (n = 3), Cer1 (n = 3), and Lefty1/2 (n = 3). Scale bars, 100 μm.
PS formation and progression depends upon canonical Wnt signaling driven by the expression of Wnt3 in the proximal-posterior epiblast and PVE (Liu et al., 1999; Mohamed et al., 2004; Rivera-Pérez and Magnuson, 2005; Yoon et al., 2015). T is a direct target of this Wnt activity (Arnold et al., 2000). WISH showed that expression of Wnt3 appeared in the proximal and posterior part of E6.5 Cables2d/d mutants with the decreased expression compared with wild-type (Figure 3C,D). In the extraembryonic part, Bmp4 was similarly expressed in Cables2d/d embryos compared with wild-type embryos at E6.5 (Figure 3G,H), suggesting that the extraembryonic ectoderm is develops normally in mutant embryos at least until E6.5. On the other hand, the pluripotency marker, Oct4 was expressed normally in Cables2d/d mutants at E6.5 (Figure 3I,J).
We next examined markers of the distal/anterior components of the axis in Cables2d/d embryos. Proper activation of the Nodal signaling in VE is required for the AVE formation (Takaoka and Hamada, 2012). Nodal was normally expressed in Cables2d/d embryos at E6.0 (data not shown). Subsequently, Nodal expression normally localizes to the nascent PS and the posterior epiblast at E6.5; however, in E6.5 Cables2-deficient embryos Nodal expression remains throughout the epiblast (Figure 3K,L). Lhx1, which is normally expressed in the AVE and nascent mesoderm of wild-type embryos, was accumulated in the distal part of E6.5 Cables2d embryos (Figure 3M,N). The normal formation of definitive endoderm and extraembryonic endoderm in mutant embryo was confirmed by the expression of Sox17 (Figure 3O,P). Our data also showed that Cerberus 1 (Cer1) and Lefty1, antagonists of Nodal signaling, were expressed at lower levels in Cables2d/d embryos compared to the wild-type at E6.5 (Figure 3Q–T). Furthermore, WISH analyses demonstrated absent or decreased expression of Lefty2 in the posterior epiblast of Cables2d embryos at E6.5 (Figure 3S,T). The combined results of WISH analyses suggested that PS formation is retarded in the Cables2d model but A-P axis is established.
Activation and interaction of Cables2 with Wnt/β-catenin signaling
The Cables2 paralog (Cables1) binds to β-catenin (Rhee et al., 2007) and, in fact, the Wnt/β-catenin targets are downregulated in the Cables2 mutant embryo (Figure 3A–D). We therefore examined whether Cables2 facilitates β-catenin activity at Wnt target sites and physically interacts with β-catenin. Cables2-activated β-catenin/TCF-mediated transcription in vitro with an almost twofold increase in relative TOP/FOP luciferase activity (Figure 4A). Moreover, co-IP using N-terminal FLAG-tagged Cables2 (FLAG-Cables2)-transfected 293T cell lysates with or without exogenous β-catenin indicated that β-catenin was present in the precipitated complexes with Cables2 (Figure 4B and Figure 4—figure supplement 1). These data suggested that Cables2 physically associates with β-catenin and increases its transcriptional activity at Wnt-responsive genes in vitro.
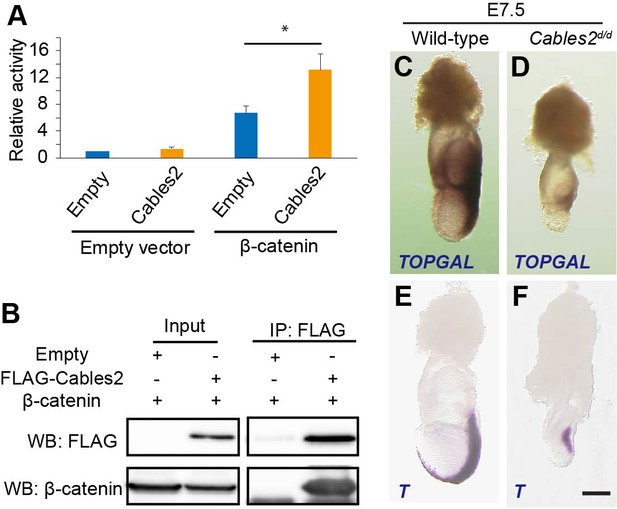
Enhancement of β-catenin activity by Cables2.
(A) Relative luciferase activities in 293T cells transfected with an empty control or Cables2 expression vectors together with an empty control or β-catenin expression vectors. Relative luciferase activity is expressed as the ratio of TOP/FOPflash reporter activity relative to the activity in cells transfected with an empty vector alone. Columns: Averages of at least three independent experiments performed in triplicate. Error bars, Standard deviation (SD). Statistical significance was determined using Student’s t test (*, p<0.05). (B) Co-IP was performed with FLAG-Cables2 and β-catenin expression vectors. The results obtained using anti-FLAG and anti-β-catenin antibodies showed the appearance of β-catenin in the precipitated complexes with Cables2. (C, D) β-Galactosidase staining demonstrating the restricted activation of Wnt/β-catenin signaling in Cables2d homozygous embryo carrying the TOPGAL reporter (n = 6). (E, F) WISH analysis showing the expression of T in wild-type and Cables2d embryos at E7.5 (n = 5). Scale bars, 100 μm.
Accumulating evidence suggests that Wnt/β-catenin signaling is implicated in the formation of AVE and further PS (Engert et al., 2013; Huelsken et al., 2000; Lickert et al., 2002). To assess the functional significance of altered Wnt3 ligand expression, Cables2d mice were crossed with the TOPGAL transgenic mice, which express the β-galactosidase under the control of three copies of the Wnt-specific LEF/TCF binding sites (Moriyama et al., 2007). Beta-galactosidase was detected in the fully elongated primitive streak and in the adjacent posterior tissues as expected in wild-type E7.5 embryos carrying TOPGAL (Figure 4C). In contrast, E7.5 Cables2d/d embryos carrying TOPGAL showed diminished β-galactosidase only in the proximal-posterior PS (Figure 4D). Moreover, T transcripts were observed in the PS of the mutant embryos, but not extending to the distal point of the embryo, and there was no signal in the axial mesendoderm (Figure 4E,F). These results suggested altered transcription activation of Wnt/β-catenin signaling in Cables2d/d embryos.
Increased apoptotic cells in Cables2d embryo at E7.5
Cell proliferation and apoptotic cell death are key events during embryonic development. To clarify the cell growth status, we performed EdU assay and measured the percentage of EdU-positive cells. There was no significant difference in the percentage of proliferation cells between wild-type and Cables2d/d embryos at E6.5 (Figure 5A,B,M). Furthermore, a simultaneous TUNEL assay was performed to determine whether the reduced size of Cables2d/d embryos could be attributed to increased programed cell death. Although apoptotic cells were detected, the average percentage of dead cells in Cables2d/d embryos was not significantly different from that in wild-type embryos (Figure 5C–F,N). These results suggest that cell proliferation and apoptotic cell death are normal in Cables2d embryos until E6.5. Interestingly, the percentage of proliferative cells of the wild-type and mutant embryos were comparable at E7.5 (Figure 5G,H,M), however, the TUNEL-positive apoptotic cells increased significantly in Cables2d/d E7.5 embryos while wild-type embryos exhibited few (Figure 5I–L,N). These results suggested that increased programed cell death occured in Cables2d/d model after E6.5.
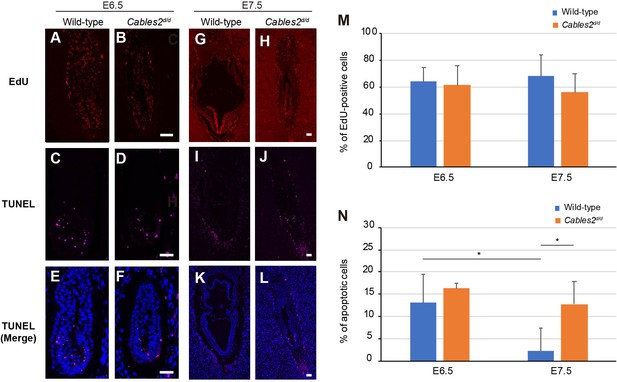
Proliferating and apoptotic cells in Cables2d embryos.
(A–B) The EdU-incorporating cells represented the proliferation of cells in both wild-type and Cables2d/d embryos at E6.5 (n = 6). (C–F) Apoptotic cells were detected in both embryonic and extraembryonic parts of wild-type and Cables2d/d embryos (n = 6). (G–N) The proliferative and apoptotic cells at E7.5 were examined and showed the percentage in both wild-type and Cables2d/d embryos (M, N). The average percentage was calculated by number of counted cells normalized to total number of cells within the embryo. Statistical significance was determined using two-way ANOVA (*, p<0.05). Error bars, Standard deviation (SD). Scale bars, 50 μm.
Decreased Rps21 gene expression and elevated p53 pathway in Cables2d embryo
We performed RNA-seq with embryo samples at E6.5 and E7.5 to examine global gene changes. Kyoto Encyclopedia of Genes and Genomes (KEGG) pathways and Gene ontology (GO) terms were analyzed to find the maximum enrichment using the Enrichr program (Chen et al., 2013; Kuleshov et al., 2016). A heatmap resulted different expression genes in four groups with a cutoff of fold change > 2, FDR < 0.05 (Figure 6A, Supplementary file 1). At E6.5, the mutant embryos showed few differences from wild-type (fold change > 2, FDR < 0.05) with three genes downregulated and eight genes upregulated (Figure 6B–D, Supplementary file 2). Interestingly, aside from Cables2, Rps12 and Rps21 are significantly down-regulated in E6.5 mutant embryos (Figure 6B). Rps12 was highly decreased at E6.5 in Cables2d embryos, however, not significantly different from wild-type embryos at E7.5. Importantly, Rps21 is a gene located next to the exon 10 of the Cables2 locus in opposite orientation. We re-confirmed the quantitative decrease in Rps21 mRNA in Cables2d/d embryos at E7.5 (Figure 6H). Eight upregulated genes in Cables2d embryos including inducers and effectors of p53 indicated the ‘p53 signaling pathway’ is particularly enhanced without Cables2 (Figure 6C). Enhanced p53 processes include cyclin-dependent protein kinase regulation and cell cycle arrest response to DNA damage (Figure 6D). The expression of significantly upregulated genes Ccng1, Trp53inp1, and Cdkn1a (p21) was quantitatively measured using E7.5 embryos. Notably, comparable Trp53 expression suggests no impairment of Trp53 transcription.
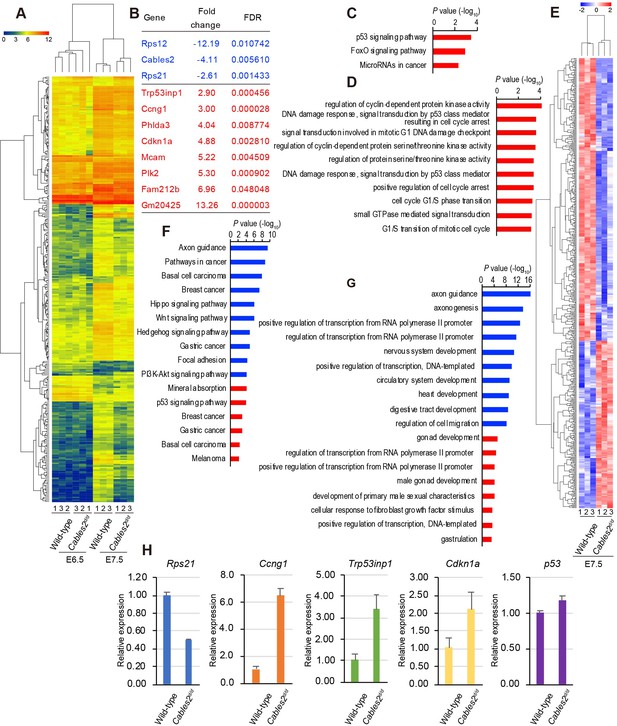
Transcriptome profiling analysis of Cables2d/d embryos.
(A) Heatmap representation of 288 genes significantly different between wild-type and Cables2d/d embryo samples (fold change >= 2, FDR < 0.05). (B) List of all downregulated (blue) and upregulated (red) genes expressing in Cables2d embryos at E6.5. (C) KEGG pathway (p<0.01) and (D) GO Biological process (p<0.001) were identified among up-regulated genes at E6.5. (E) Heatmap (Z score) for the expression of 350 downregulated and 207 upregulated genes in Cables2d/d embryos at E7.5. Different expression genes at E7.5 enriched in KEGG pathway (F) and GO Biological process (G) (p<0.001) with downregulation in blue bars and upregulation in red bars. (H) RT-qPCR validated the expression levels of representative upregulation genes at E6.5 using Cables2d/d embryos E7.5 (n = 5). Averages of three independent experiments performed in duplicated and normalized against the expression levels of Gapdh. Error bars, Standard deviation (SD).
At E7.5, the differentially expressed genes of mutant Cables2 and control embryos (fold change >2, FDR < 0.05) include 350 downregulated genes and 207 upregulated genes, indicating that the transcriptome signature was significantly disturbed at E7.5 (Figure 6E, Supplementary file 3). The enriched pathway of downregulated genes included ‘axon guidance’, ‘Wnt signaling pathway’, ‘Hippo signaling pathway’, ‘PI3K-Akt signaling pathway’ and the GO terms related to ‘axonogenesis’, ‘nervous system development’, ‘circulatory system development’ and ‘heart development’. The upregulated KEGG pathways were ‘mineral absorption’, ‘p53 signaling pathway’ and the GO terms related to ‘gonad development’, ‘male gonad development’ and ‘development of primary male sexual characteristics’ (Figure 6F,G). These data revealed programmed cell death and the p53 pathway may impair gastrulation and contribute to Cables2 mutant embryo lethality. Transcriptome profiling comparison suggested that disruption of entire Cables2 locus affects the expression of not only Cables2, but also Rps21, gene abutting the Cables2, and induces the enhanced expression of p53-target genes dramatically.
The discrepant phenotypes in Cables2 deletion models
The results of RNA-seq raise the question of which gene, Cables2 or Rps21, is the main cause of the elevated expression of p53-target genes and embryonic lethality in Cables2d mice. To explore the specific function of Cables2 in embryonic development, the Cables2 conditional KO exon one mice was obtained using CRISPR/Cas9 system. During the modified-gene mouse production, the Cables2 exon one deletion mice (Cables2e1) also were generated (Figure 7A). To re-confirm the lethal phenotype, Cables2e1 mice were exclusively intercrossed and propagated. Unexpectedly, viable and fertile homozygous Cables2e1/e1 mice were obtained, which is contrary to entire locus Cables2d phenotype. This surprising result indicates an inconsistent function of Cables2 in embryogenesis. RT-PCR analysis of adult mouse brain showed that expression of Cables2 mRNA was deleted in Cables2e1/e1 tissues (data not shown). Moreover, the quantitative RT-PCR confirmed lack of Cables2 expression in Cables2e1/e1 compared with Cables2d heterozygote and wild-type (Figure 7B), suggesting that Cables2 is not transcribed in the Cables2e1/e1 mouse.
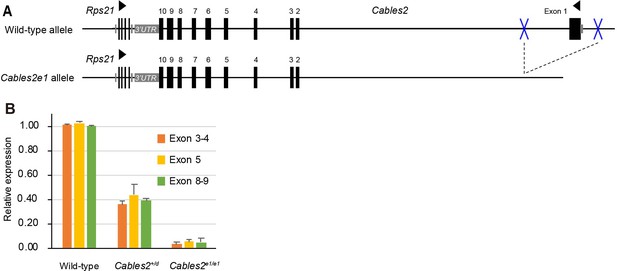
Gene construction and expression in Cables2e1 mutant mouse.
(A) The Cables2e1 mice was generated using CRISPR/Cas9 system. Blue marks indicate the target sites on the left and right of exon1 of Cables2. (B) RT-qPCR using adult brain samples showed the quantitation of Cables2 expression in wild-type, heterozygous Cables2+/d and homozygous Cables2e1/e1 mice. The expression levels were validated in different exons of Cables2. Averages of three independent experiments performed in duplicated and normalized against the expression levels of Gapdh. Error bars, Standard deviation (SD).
Investigating the phenotype of Rps21 deletion and Rps21-indel mice
We considered that Rps21 may contribute to Cables2d lethality due to its significant downregulation. Rps21 is a factor for protein translation initiation and is a potential regulator for early differentiation of mammary epithelial stem cells using the HC11 mouse cell line (Perotti et al., 2009; Török et al., 1999). To date, to our knowledge, in vivo Rps21 function has not been reported in the mouse model. To identify Rps21 function, we generated Rps21 deletion mice (Rps21d), in which exon 2 to exon 6 is deleted, and Rps21-indel mice with an identified frameshift occurs (Figure 8, Supplementary file 4). We could obtain 11 Rps21d heterozygous founders, but homozygous mice were not available (Table 2). Surprisingly, adult Rps21+/d mice exhibited white abdomen spots, white hind feet, and a kinked tail similar to Bst heterozygous mice, in which the Rpl24 gene is mutated and homozygous mutant dies before E9.5 (Oliver et al., 2004). Notably, seven of eleven founders developed smaller body size compared with that littermates and died before 8 weeks. One remaining male founder was sterile, thus unable to produce progeny. The Bst phenotype was confirmed in Rps21+/d heterozygous F1 mice with extremely low fertility. On the other hand, no signs of pregnancy were observed in all eight Rps21-indel pseudopregnant mice unexpectedly. We performed Caesarean sections and no embryos were found, with only eight implantation sites detected in all the uteruses by 2% sodium hydroxide (data not shown) indicating prenatal lethality. These results suggested the semidominant phenotype of Rps21 mutant models; however, the critical developmental stage regulated by Rps21 remains unclear. Compared with Cables2d model, the heterozygote Rps21d showed more severe phenotype including small body size, infertility, and postnatal lethality or prenatal lethality in the case of Rps21-indel. These data indicated that Rps21 could be essential for mouse development and the embryonic lethality in Cables2d mice resulted from diminished Rps21 expression. However, it remains unclear why heterozygous Rps21d mice survived during the embryonic development, whereas Cables2d/d mice with 50% Rps21 expression showed embryonic lethality.
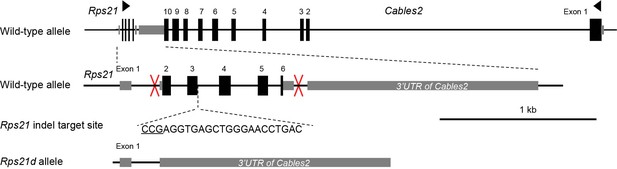
Gene construction of Rps21d and Rps21-indel mutant mice.
Rps21d and Rps21-indel mutant mice were generated using CRISPR/Cas9 system. One-cut target site was designed to induce the mutation in Rps21-indel. Left and right target sites were introduce (red marks) to delete exon 2 to exon 6 of Rps21 in Rps21d mouse. Schematic showed the full-length of Rps21 including six exons and abutting to 3’UTR of Cables2 gene.
Generation of Rps21 mutant mice.
Strain | Electroporated embryos | Transfer embryos | Total number of mice | ||
---|---|---|---|---|---|
Newborn | Founder | ||||
Male | Female | ||||
Rps21d | 207 | 199 | 38 | 7 | 4 |
Rps21-indel | 274 | 200 | 0* | - | - |
-
* At E16.5, there were eight implanted sites.
Overexpression of Cables2 in the epiblast rescues the proper gastrulation stage in Cables2d tetraploid embryo
To determine function of Cables2 in embryos with diminished Rps21 expression, chimera analysis was performed using tetraploid wild-type embryos and Cables2d/d ESCs derived from the entire locus Cables2d embryos. Tetraploid complementation chimera have the advantage that the host tetraploid embryos can only contribute to primitive endoderm derivatives and trophoblast compartment of the placenta, whereas epiblast components are completely derived from ESCs (Tanaka et al., 2009). Tetraploid wild-type morula was aggregated with Cables2d/d ESCs to produce chimera in which Cables2 was exclusively deleted in the epiblast (Cables2d Epi KO chimera) (Figure 9A). YFP reporter gene was inserted into ROSA26 locus of Cables2d/d ESCs to construct Cables2d/d; ROSA26YFP/+ ESCs which allows for embryo visualisation and imaging. We collected the Cables2 chimeric embryos at the indicated embryonic stage and analyzed the phenotype (Table 3). Like Cables2d/d embryos, the epiblast of Cables2d Epi KO embryos were smaller in size than that of control wild-type chimera littermates at E7.5 and E8.5 (Figure 9B–E). In similar to Cables2d/d gastrulas, growth retardation was observed in the Cables2d Epi KO chimera gastrulas. Next, tetraploid wild-type morula was aggregated with Cables2d/d; ROSA26YFP/+; CAG-tdTomato-2A-Cables2 ESCs to produce Cables2d Epi rescue chimera (Figure 9F). CAG-tdTomato fused 2A-Cables2 was randomly integrated into the genome of Cables2d/d;ROSA26YFP/+ ESCs to ubiquitously overexpress Cables2 in the epiblast and its derivatives. Red fluorescence was detected in Cables2d/d; ROSA26YFP/+; CAG-tdTomato-2A-Cables2 (Cables2d/d; ROSA26YFP/+; tdT-C2) ESCs, suggesting that tdTomato-2A-Cables2 was correctly translated in the cells (Figure 9G–N). Interestingly, Cables2d Epi rescue chimeras were indistinguishable from wild-type chimera littermates at all stages (Figure 9G–N). Altogether, the lethal phenotype of Cables2d/d embryos was rescued by Cables2 exogenous overexpression until at least E9.5 during gastrulation.
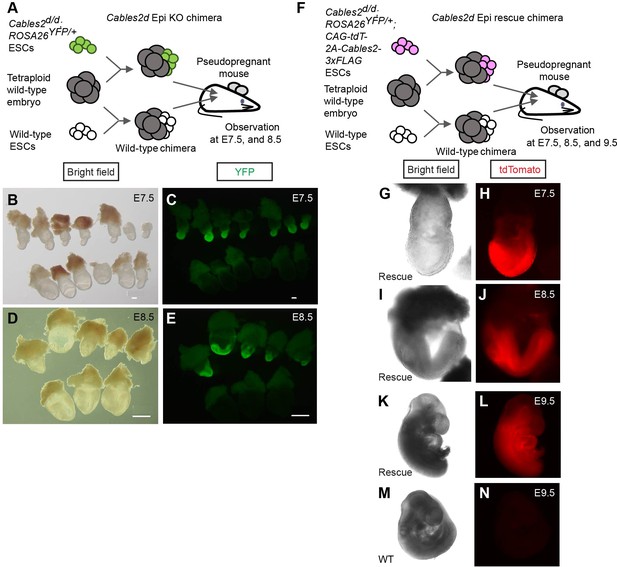
Defective and normal gastrulation development in Cables2d Epi KO and Cables2d Epi rescue chimeras, respectively.
(A) Schematic diagram of tetraploid complementation experiment for Cables2d Epi KO chimera. (B–E) Bright field (B, D) and YFP fluorescent (C, E) images of wild-type and Cables2d Epi KO chimeric embryos at E7.5 or E8.5. (F) Schematic diagram of tetraploid complementation experiment for Cables2d Epi rescue chimeras. Bright field (G, I, K, M) and tdTomato fluorescent (H, J, L, N) images of wild-type and Cables2d Epi rescue chimeric embryos at E7.5, E8.5, or E9.5. The Cables2d Epi rescue chimeric embryos developed normally until E9.5. Scale bars, 100 μm (B, C); 500 μm (D, E).
Phenotypes in Cables2d Epi KO and Cables2d Epi rescue chimeras.
Tetraploid embryo + Cables2d/d; ROSA26YFP/+ ESC (Epi KO chimera) | Wild-type chimera | |||||
---|---|---|---|---|---|---|
Embryonic days (E) | Total number of embryos | Phenotype | Total number of embryos | Phenotype | ||
Normal | Abnormal | Normal | Abnormal | |||
E6.5 | 2 | 1 | 1 | 4 | 3 | 1 |
E7.5 | 15 | 4 | 11 | 14 | 11 | 3 |
E8.5 | 13 | 0 | 13* | 7 | 6 | 1 |
Tetraploid embryo + Cables2d/d; ROSA26YFP/+; CAG-tdTomato-2A-Cables2-3xFLAG ESC (Epi rescue chimera) | Wild-type chimera | |||||
Embryonic days (E) | Total number of embryos | Phenotype | Total number of embryos | Phenotype | ||
Normal | Abnormal | Normal | Abnormal | |||
E7.5 | 5 | 4 | 1 | 2 | 1 | 1 |
E8.5 | 4 | 3 | 1 | 5 | 4 | 1 |
E9.5 | 2 | 2 | 0 | 3 | 2 | 1 |
-
* All embryos had A-P axis specification.
Discussion
In this study, we provided the first evidence regarding the physiological roles of Cables2 in mice. We demonstrated that Cables2 is expressed widely during early embryonic development and full Cables2 locus deletion caused defective PS formation, increased apoptotic cells, growth retardation, and post-gastrulation embryonic lethality. Many other mouse mutants with impaired A–P axis or PS formation either become highly dysmorphic or complete gastrulation without growth retardation but exhibit patterning defects. The divergent phenotype resulting from entire Cables2 deletion suggests multiple faulty processes during early mouse development. Further, transcriptome profiling comparison showed Cables2 and Rps21 impairments enhanced Wnt and p53 signaling pathways, possibly contributing to peri-gastrulation arrest in entire locus Cables2d embryos. Notably, the heterozygous Rps21+/d mice showed the semidominant Bst phenotype and almost died before two months old and no homozygous mutants were obtained, indicating embryonic lethality. The deletion of exclusive Cables2 by removing critical exon one in Cables2e1 model resulted in live and fertile mice versus the lethal phenotype of entire locus Cables2d mice. However, the tetraploid complementation experiments demonstrated that the A–P axis was formed normally with the wild-type VE and trophoblast compartment, and the growth retardation in the epiblast can be rescued by overexpressing Cables2. Thus, the Cables2/Rps21-impaired genotype is lethal during gastrulation, and novel interaction of Cables2 with Wnt/β-catenin and p53 pathways can rescue mouse development.
Importantly, lack of Cables2 transcription itself does not disrupt gastrulation in mice, as Cables2e1/e1 mice survive. Conventional full gene locus deletion may affect Rps21 located in the proximity. Diminished expression of Rps21 was observed when the entire Cables2 locus was deleted. Rps21 belongs to the ribosome family. In mammals, the ribosome family includes 79–80 ribosomal proteins and four ribosomal RNAs that play a vital role in ribosome biogenesis, a fundamental process for cellular proliferation, apoptosis, and maintenance (Kressler et al., 2010; Maguire and Zimmermann, 2001). Ribosomal proteins function not only in protein synthesis but also in genetic diseases and tumorigenesis (Lai and Xu, 2007; Zhou et al., 2015). Some ribosomal proteins are demonstrated in vivo as necessary factors for mouse development such as Rplp1, Rpl24, Rpl38, Rps3, and Rps6 (Kondrashov et al., 2011; Oliver et al., 2004; Panić et al., 2006; Peng et al., 2019; Perucho et al., 2014). Recently, Rps21 was described as a human oncogene, especially in prostate cancer and osteosarcoma (Arthurs et al., 2017; Liang et al., 2019; Wang et al., 2020). However, Rps21 in vivo function remains unknown. Our results revealed that the Rps21 mutant model showed the semidominant phenotype of pleiotropic abnormality including postnatal lethality. This study is the first report of Rps21d mouse and describe the essential function of Rps21 for pre- and post-natal development in mice.
We found upregulation of p53 signaling pathway-related genes, such as Ccng1, Trp53inp1 and Cdkn1a, in full locus Cables2d gastrulas that accompanied with decreased expression of Rps21. The transcription factor p53 is well-known to function in DNA damage responses and tumor suppression in cancer (Vogelstein et al., 2000; Zilfou and Lowe, 2009). P53 activates checkpoint regulation in ribosomal protein deficiency, rather than ribosome dysfunction. Interestingly, genetic deletion of p53 can rescue the lethal Rps6 phenotype. The Rps6 haploinsufficiency embryo exhibited peri-gastrulation lethality after E5.5 but can be rescued until E12.5 by genetic inactivation of p53 (Panic, et al., 2006). As mentioned before, heterozygous mutation in the Rpl24 gene causes the Bst phenotype. Homozygous Rpl24 deficiency shows early embryonic lethality. The abnormal postnatal phenotypes are largely caused by the aberrant up-regulation of p53 protein expression during embryonic development including the gastrulation stage (Barkić et al., 2009). These reports support that diminished expression of Rps21 attenuates embryonic growth by reinforced induction of p53-dependent checkpoint response in entire locus Cables2d mice.
Heterozygous deficiency of Rps21 showed the Bst phenotype similar to that of Rpl24, but not that of Rps6. Interestingly, although expression of Rps21 was maintained at a half level in Cables2d gastrulas compared with wild-type gastrulas, they caused early embryonic lethality that was different abnormality from heterozygous Rps21d mice. Further, we generated Cable2d/e1 mutant embryos by intercrossing between Cables2+/d and Cables2+/e1. The compound Cable2d/e1 embryos showed normal development by E9.5 with Mendelian ratio (Supplementary file 6) and maintained at approximately 75% on expression levels of Rps21, suggesting that a threshold level of Rps21 expression is required for early mouse embryonic development (Figure 7—figure supplement 1). However, it is thought that phenotype discrepancy between Cables2d/d and Rps21+/d would be attributed to the complete loss of Cables2 function, in addition to the hypofunction of Rps21. Actually, the impaired Rps21/Cables2 epiblast can be rescued and developed to the early organogenesis period through the gastrulation stage by inducting ubiquitous overexpression of exogenous Cables2 throughout embryonic development. Therefore, our results imply that Cables2 itself could play the role of fetal growth by resisting activation of p53 signaling pathway which results from a decrease in Rps21.
Genetic experiments that ablate Wnt3 activity in either the VE or epiblast alone (and therefore reduce overall posterior Wnt signal) have shown that this activity regulates the timing of PS formation. Embryos in which VE Wnt3 function is ablated have delayed primitive streak formation but by E9.5 are indistinguishable from wild-type littermates (Yoon et al., 2015). When Wnt3 function is removed from the epiblast, PS formation is delayed and by mid-gastrulation the embryos are highly dysmorphic with the PS bulging toward the amniotic cavity (Tortelote et al., 2013). In entire locus Cables2d embryos, the WISH analysis with gastrulation markers showed that PS formation is retarded or delayed at gastrulation initiation with the reducing of Wnt3 expression and activity. However, the cellular apoptosis was increased afterward and caused the growth failure. In Figure 5O, TUNEL-positive cells were abundantly observed in the epiblast of Cables2d embryos at E7.5, but not in the visceral endodermal cells. The result suggests that diminished Wnt/β-catenin signaling with Cables2 deficiency might be involved in retardation of AVE and/or PS formation rather than diminished level of Rps21. In addition, Rps21 expression is not reported in embryo endoderm and mesoderm by MGI database (http://www.informatics.jax.org), further supporting our hypothesis.
Cables1 is widely studied in cancer research. It is generally thought that Cables1 is a tumor suppressor gene. Loss of Cables1 expression is found with high frequency in human cancer such as lung, colon, ovarian, and endometrial cancers (Arnason et al., 2013; Kirley et al., 2005a; Kirley et al., 2005b; Zukerberg et al., 2004). In mouse model, genetic inactivation of Cables1 leads to an increased incident of endometrial cancer (Zukerberg et al., 2004) and colon cancer (Arnason et al., 2013; Kirley et al., 2005a). Cables1-deficient mouse embryonic fibroblast cells exhibit an increased growth rate (Kirley et al., 2005b). Further, Cables2 shows involvement of in both p53-dependent and p53-independent apoptotic pathway by using in vitro analyses (Matsuoka et al., 2003). Given that Cables family share the identity of amino acid more than 70% at C-ter (Sato et al., 2002), although we expected that Cables2 may have similar function with Cables1 in mouse and human, the present study provided us controversial observation on the physiological role of Cables2. Recently, Shi et al., 2015 reported that Akt (Ser/Thr kinase)-phosphorylation-mediated 14-3-3 binding prevents the apoptosis-inducing function of Cables1 for cell growth. Emerging evidence reveals that Cables1 can interact with a variety of proteins (Arnason et al., 2013; Kirley et al., 2005a; Kirley et al., 2005b; Zukerberg et al., 2004). Cables1 changes physiological phase dependent on counter partner protein. This study also demonstrated that Cables2 physically interacted with β-catenin. Moreover, we are understanding that Cables2 has the ability to physically interact with one kind of self renewal/pluripotent factor in vitro (data not shown). Therefore, it seems that Cables family protein is a signaling hub for the regulation of the cell cycle, cell growth, cell death and differentiation. However, how Cables2 controls temporal, spatial and physical interaction in vivo for resisting p53 signaling pathway during gastrulation remains unknown and needs further investigation.
Lastly, this study highlights the need to validate target knock-out genes as well as nearby genes in lethal phenotypes. In conclusion, our results suggest that Rps21 expression is essential for gastrulation and Cables2 assists it in case of decreased ribosomal biogenesis, via an unknown mechanism. Furthermore, Cables2 functions together with Wnt/β-catenin and p53 pathways in early embryonic development. These novel interactions should be expanded in future studies to give insight into the function of the Cables protein family and uncover additional roles for this protein.
Materials and methods
Reagent type (species) or resource | Designation | Source or reference | Identifiers | Additional information |
---|---|---|---|---|
Gene (M. musculus) | Cables2 | PMID:11955625 | MGI:2182335 | |
Gene (M. musculus) | Rps21 | PMID:10022917 | MGI:1913731 | |
Strain, strain background (M. musculus) | B6.Cg-Tg(TOPGAL) | Riken BRC | RBRC05918 | |
Cell line (M. musculus) | Cables2tm1(KOMP)Vlcg | KOMP | ID: VG16085, clone: 16085A-D3 RRID:MMRRC_052978-UCD | ESC line |
Cell line (Homo-sapiens) | 293T | ATCC | CRL-3216 RRID:CVCL_0063 | embryonic kidney |
Antibody | Anti-FLAG (M2) (mouse monoclonal) | Sigma-Aldrich | Cat# F1804, RRID:AB_262044 | WB(1:1000), IP (1:650) |
Antibody | Anti-β-catenin (D10A8) (rabbit monoclonal) | Cell Signaling Technology | Cat# 8480, RRID:AB_11127855 | WB(1:1000) |
Antibody | Anti-GAPDH (rabbit polyclonal) | Santa Cruz Biotechnology | Cat# sc-25778, RRID:AB_10167668 | WB(1:1000) |
Antibody | Normal mouse IgG (mouse isotype control) | Santa Cruz Biotechnology | Cat# sc-2025, RRID:AB_737182 | IP(1:250) |
Antibody | Anti-Mouse IgG-HRP (secondary antibody) | GE healthcare | Cat# NA931, RRID:AB_772210 | WB(5000) |
Antibody | Anti-Rabbit IgG-HRP (secondary antibody) | GE healthcare | Cat# NA934, RRID:AB_772206 | WB(5000) |
Peptide, recombinant protein | TrueCut Cas9 Protein | Thermo Fisher Scientific | Cat# A36498 | |
Peptide, recombinant protein | Dynabeads Protein G | Thermo Fisher Scientific | Cat# 10003D | |
Commercial assay or kit | GeneArt Precision gRNA Systhesis Kit | Thermo Fisher Scientific | Cat# A29377 | |
Commercial assay or kit | Lipofectamine 3000 Reagent | Thermo Fisher Scientific | Cat# L3000015 | |
Commercial assay or kit | AmpliTag Gold 360 Master Mix | Thermo Fisher Scientific | Cat# 4398886 | |
Commercial assay or kit | RNeasy Mini Kit | Qiagen | Cat# 74104 | |
Commercial assay or kit | TB Green Premix Ex Taq II | Takara | Cat# RR820B | |
Commercial assay or kit | Dual-Glo Luciferase assay system | Promega | Cat# E2920 | |
Commercial assay or kit | Click-iT Plus EdU Imaging Kit | Thermo Fisher Scientific | Cat# C10638 | |
Commercial assay or kit | Click-iT Plus TUNEL Assay for In situ Apoptosis Detection kit | Thermo Fisher Scientific | Cat# C10619 | |
Software | CLC Genomics Workbench | Qiagen | RRID:SCR_011853 |
Animals and husbandry
Request a detailed protocolICR mice were purchased from CLEA Japan Co. Ltd. (Tokyo, Japan); C57BL/6N mice were purchased from Charles River Laboratory Japan Co. Ltd (Yokohama, Japan). For production of staged embryos, the day of fertilization as defined by the appearance of a vaginal plug was considered to be embryonic day 0.5 (E0.5). Animals were kept in plastic cages (4–5 mice per cage) under specific pathogen-free conditions in a room maintained at 23.5 ± 2.5°C and 52.5 ± 12.5% relative humidity under a 14 hr light:10 hr dark cycle. Mice had free access to commercial chow (MF; Oriental Yeast Co. Ltd., Tokyo, Japan) and filtered water throughout the study. Animal experiments were carried out in a humane manner with approval from the Institutional Animal Experiment Committee of the University of Tsukuba (#19–057 and #20–065) in accordance with the Regulations for Animal Experiments of the University of Tsukuba and Fundamental Guidelines for Proper Conduct of Animal Experiment and Related Activities in Academic Research Institutions under the jurisdiction of the Ministry of Education, Culture, Sports, Science, and Technology of Japan.
Generation and genotyping of target gene-deficient mice
Request a detailed protocolThe targeted ESC clone Cables2tm1(KOMP)Vlcg was purchased from KOMP (project ID: VG16085, clone number: 16085A-D3, RRID:MMRRC_052978-UCD). To generate Cables2d mice, ESCs were aggregated with the wild-type morula and transferred to pseudopregnant female mice. Male chimeras that transmitted the mutant allele to the germ line were mated with wild-type females to produce Cables2d mice with the C57BL/6N background. To produce Cables2 conditional KO exon 1, Cables2 exon 1 CRISPR left and right target sites were designed (Supplementary file 4), oligo DNAs were annealed, purified, inserted into the pX330 vector (Addgene plasmid 42230, a gift from Dr. Feng Zhang [Cong et al., 2013]) and checked the in vitro activity by EGxxFP system (Fujihara and Ikawa, 2014). The ssDNA donors were co-injected with CRISPR vector to insert loxP and EcoRI to upstream, loxP and EcoRV to downstream of exon 1, respectively. Then Cables2 exon one deletion mice exclusively were propagated and analyzed.
Rps21d and Rps21-indel mutant mice were generated using CRISPR/Cas9 system. Firstly, gRNAs were synthesised using GeneArt Precision gRNA Systhesis Kit (Thermo Fisher Scientific). Then the sgRNAs were co-electroporated with Cas9 protein using TrueCut Cas9 Protein v2 (Thermo Fisher Scientific) into mouse zygote (Supplementary file 4).
In all strains, adult mice were genotyped using genomic DNA extracted from the tail. For whole-mount in situ hybridization, embryos were genotyped using a fragment of yolk sac and Reichert membrane. Samples were dispensed into lysis solution (50 mM Tris-HCl, pH 8.5, 1 mM EDTA, 0.5% Tween 20) and digested with proteinase K (1 mg/mL) at 55°C for 2 hr, inactivated at 95°C for 5 min, and then subjected to PCR. For paraffin slides, embryos were genotyped using tissue picked from sections and digested directly with proteinase K (2 mg/mL) in PBS. For others experiments, after collecting data, the whole embryos were used for genotyping. Genotyping PCR was performed with AmpliTag Gold 360 Master Mix (Thermo Fisher Scientific, Tokyo, Japan) and primer listed in Supplementary file 6.
TOPGAL reporter mice
Request a detailed protocolB6.Cg-Tg(TOPGAL) transgenic mice carrying LEF/TCF reporter of Wnt/β-catenin signaling were used for visualizing Wnt signaling pathway in vivo. TOPGAL mice were obtained from Riken BRC (RBRC02228). Animals were kept and maintained under the same conditions as described above. To produce the TOPGAL reporter in the homozygous Cables2 background, TOPGAL heterozygotes were crossed with Cables2 heterozygotes subsequently and finally, homozygous Cables2 carrying TOPGAL transgene were collected at E7.5 together with littermates. All embryos were stained S-gal (Sundararajan et al., 2012) and then genotyped using both Cables2 genotyping primers and TOPGAL primers (Supplementary file 6).
Cell culture
Request a detailed protocol293T cells were obtained from The American Type Culture Collection (Manassas, Virginia). Cells were cultured in Dulbecco’s modified Eagle’s medium (DMEM) supplemented with 10% heat-inactivated fetal bovine serum. Mouse embryonic stem cells (ESCs) were maintained on 0.1% gelatine-coated dishes in mouse ESC medium consisting of DMEM containing 20% knockout serum replacement (KSR; Thermo Fisher Scientific), 1% non-essential amino acids (Thermo Fisher Scientific), 1% GlutaMAX (Thermo Fisher Scientific), 0.1 mM 2-mercaptoethanol (Thermo Fisher Scientific), and leukemia inhibitory factor (LIF)-containing conditioned medium, supplemented with two chemical inhibitors (2i), that is 3 μM CHIR99021 (Stemgent inc, Cambridge, Massachusetts) and 1 μM PD0325901 (Stemgent).
RT-PCR, RT-qPCR, and RNA-seq
Request a detailed protocolCultured ESCs, about 130 blastocysts, and 21 embryos at E7.5 were collected. Total RNAs from blastocysts and embryos were extracted using Isogen (Nippon Gene Co., Ltd., Tokyo, Japan). RNA from ESCs was collected using an RNeasy Mini Kit (Qiagen K.K., Tokyo, Japan). The cDNA was synthesized using Oligo-dT primer (Thermo Fisher Scientific) and SuperScript III Reverse Transcriptase (Thermo Fisher Scientific) in a 20 μL reaction mixture. RT-qPCR was performed using TB Green Premix Ex Taq II (Takara) and the Thermal Cycler Dice Real Time System (Takara) according to the manufacturer’s instructions and target gene expression level was normalized to the endogenous Gapdh expression level (Supplementary file 6).
RNA sequencing analysis was performed by Tsukuba i-Laboratory LLP as previously described (Ohkuro et al., 2018). Briefly, total RNAs were extracted from wild-type and Cables2d embryos, two embryos/sample (n = 3), using Trizol reagent (Thermo Fisher Scientific). RNA quality was evaluated using Agilent Bioanalyzer with RNA 6000 Pico kit (Agilent Technologies Japan, Ltd., Tokyo, Japan). Total low-input RNA was used for rRNA-depletion and library synthesis by Takara SMARTer kit (Takara). RNA-seq library was prepared with Agilent Bioanalyzer, DNA High-sensitivity kit (Agilent Technologies Japan, Ltd., Tokyo, Japan) and performed with Illumina NextSeq500 (Illumina K.K., Tokyo, Japan) by Tsukuba i-Laboratory LLP (Tsukuba, Japan). RNA-seq data was analyzed by CLC Genomics Workbench (Qiagen). Normalization is performed by quantile method and Log2-convert normalized value after adding one for drawing heatmap. For pairwise analysis, edgeR analysis (Empirical Analysis of DGE in CLC) was performed. ANOVA analysis (Gaussian Statistical Analysis in CLC) was performed for four groups comparison. Genes were filtrated by FDR p-value, fold change and exported in Excel format. The Enrichr program was used for GO terms and KEGG pathway enrichment analyses of differentially expressed genes with at least two-fold change and FDR < 0.05.
Vector construction
Request a detailed protocolPart of Cables2 cDNA containing exons 1 and 2 was cloned in-frame into pBlueScript KS +at the BamHI site, and the fragment containing exons 3–10 was cloned into the pcDNA3 vector at the BamHI site. These fragments were obtained and amplified from a mouse embryo E7.5 cDNA library and sequenced. The part covering Cables2 exons 1 and 2 was cut at the AfeI site and ligated into the pcDNA3 vector containing exons 3–10 to synthesize the full-length Cables2. A 1.5 kb Cables2 riboprobe was prepared by amplification from the full-length cDNA template with the pcDNA3 backbone, synthesized with Sp6 polymerase, and labeled with digoxigenin as a riboprobe.
A ROSA26 knock-in vector was constructed by insertion of CAG-Venus-IRES Pac gene expression cassette (Khoa et al., 2016) into the entry site of pROSA26-1 vector (kindly gifted from Philippe Soriano, Addgene plasmid # 21714) (Soriano, 1999). The Cables2d/d; ROSAYFP/+ was generated by electroporation of the ROSA26 knock-in vector (pROSA26-CAG-Venus-IRES Pac) into Cables2d/d ESCs. The CAG-tdTomato-2A and 3xFLAG sequences were inserted in the upstream and downstream of Cables2 cDNA, respectively, to make CAG-tdTomato-2A-Cables2-3xFLAG vector. The expression of tdTomato and FLAG-tagged Cables2 in the CAG-tdTomato-2A-Cables2 vector-transfected 293T cells were evaluated by fluorescent microscopy and western blot analysis with anti-FLAG antibody, respectively (data not shown).
Production of Cables2 rescue chimeras by tetraploid complementation assay
Request a detailed protocolTetraploid (4n) wild-type embryos were made by electrofusing diploid (2n) embryos at two-cell-stage and cultured up to morula stage. The 4 n wild-type morula were aggregated with Cables2d/d; ROSAYFP/+ or Cables2d/d; ROSAYFP/+; CAG-tdTomato-2A-Cables2-3xFLAG ESCs to form blastocyst chimeras. B6N wild-type ESCs was used as a control for tetraploid complementation assay. To obtain comparable control embryos at each stage of development, an equal number of control blastocyst chimeras were transferred together with Cables2d/d blastocyst chimeras to a pseudopregnant recipient mouse at E2.5. Embryos were recovered at from E6.5 to E9.5 and the contribution of ESCs was evaluated by YFP or tdTomato fluorescence signals.
Whole-mount in situ hybridization (WISH)
Request a detailed protocolAll embryos were dissected from the decidua in PBS with 10% fetal bovine serum and staged using morphological criteria (Downs and Davies, 1993) or described as the number of days of development. WISH was carried out following standard procedures, as described previously (Rosen and Beddington, 1994). Briefly, embryos were fixed overnight at 4°C in 4% paraformaldehyde in PBS, dehydrated, and rehydrated through a graded series of 25–50% – 75% methanol/PBS. After proteinase K (10 μg/mL) treatment for 15 min, embryos were fixed again in 0.1% glutaraldehyde/4% paraformaldehyde in PBS. Pre-hybridization at 70°C for at least 1 hr was conducted before hybridization with 1–2 μg/mL digoxigenin-labeled riboprobes at 70°C overnight. Pre-hybridization solution included 50% formamide, 4 × SSC, 1% Tween-20, heparin (50 μg/mL) (Sigma-Aldrich Japan K.K, Tokyo, Japan) and hybridization was added more yeast RNA (100 μg/mL) and Salmon Sperm DNA (100 μg/mL) (Thermo Fisher Scientific). For post-hybridization, embryos were washed with hot solutions at 70°C including 50% formamide, 4 × SSC, 1% SDS, and treated with 100 μg/mL RNase A at 37°C for 1 hr. After additional stringent hot washes at 65°C including 50% formamide, 4 × SSC, samples were washed with TBST, pre-absorbed with embryo powder, and blocked in blocking solution (10% sheep serum in TBST) for 2–5 hr at room temperature. The embryo samples were subsequently incubated with anti-digoxigenin antibody conjugated with alkaline phosphatase anti-digoxigenin-AP, Fab fragments (Roche Diagnostics K.K., Tokyo, Japan) overnight at 4°C. Extensive washing in TBST was followed by washing in NTMT and incubation in NBT/BCIP (Roche) at room temperature (RT) until color development. After completion of in situ hybridization (ISH), embryos were de-stained in PBST for 24–48 hr and post-fixed in 4% paraformaldehyde in PBS. Embryos were processed for photography through a 50%, 80%, and 100% glycerol series. Before embedding for cryosectioning, embryos were returned to PBS and again post-fixed in 4% paraformaldehyde in PBS. The specimens were placed into OCT cryoembedding solution, flash-frozen in liquid nitrogen, and cut into sections 14 μm thick using a cryostat (HM525 NX; Thermo Fisher Scientific). The following probes were used for WISH: Bmp4 (Jones et al., 1991), Brachyury (T) (Herrmann, 1991), Cer1 (Belo et al., 1997), Foxa2 (Sasaki and Hogan, 1993), Fgf8 (Bachler and Neubüser, 2001), Lefty1/2 (Meno et al., 1996), Lhx1 (Shawlot and Behringer, 1995), Nanog (Chambers et al., 2003), Nodal (Conlon et al., 1994), Oct4 (Schöler et al., 1990), Otx2 (Simeone et al., 1993), Sox2 (Avilion et al., 2003), Sox17 (Kanai et al., 1996), and Wnt3 (Roelink et al., 1990).
Co-immunoprecipitation (Co-IP)
Request a detailed protocolAt 1 day before transfection, aliquots of 5 × 104 293T cells were seeded onto poly-l-lysine (PLL)-coated 6 cm dishes and co-transfected with 2 μg of each pCAG-based expression vector using Lipofectamine 3000 (Thermo Fisher Scientific). After 48 hr, the cells were washed once with PBS, resuspended in RIPA buffer (50 mM Tris-HCl, pH 7.4, 150 mM NaCl, 1 mM EDTA, 1% deoxycholic acid and 1% Nonidet P-40 [NP-40]) containing protease inhibitor cocktail (Roche Diagnostics) and placed on ice for 30 min. The supernatant was collected after centrifugation and incubated with Dynabeads Protein G (Veritas Co., Tokyo, Japan) and mouse anti-FLAG antibody (F1804; Sigma-Aldrich) overnight at 4°C. The beads were washed four times with PBS, resuspended in Laemmli sample buffer, and boiled. The precipitated proteins were analysed by SDS-polyacrylamide gel electrophoresis (SDS-PAGE) and western blotting using the ECL Select Western Blotting Detection System (GE Healthcare Japan Co., Ltd., Tokyo, Japan) and a LAS-3000 imaging system (GE Healthcare). The FLAG antibody was then washed out and the membrane was re-stained with anti-β-catenin antibody (#8480, Cell Signalling Technology), anti-FLAG antibody (F1804; Sigma-Aldrich), and anti-GAPDH antibody (sc-25778, Santa Cruz).
Luciferase reporter assay
Request a detailed protocolA total of 50,000 cells were plated in PLL-coated 96-well tissue culture plates. After overnight culture, the cells were transfected with a specific promoter-driven firefly reporter plasmid and Renilla luciferase control plasmid, pRL-TK, using Lipofectamine 3000 Reagent (Thermo Fisher Scientific) and opti-MEM (Thermo Fisher Scientific). Luciferase activity was analysed using a luminometer and a Dual-Glo Luciferase assay kit according to the manufacturer’s instructions (Promega K.K., Tokyo, Japan). The firefly luciferase values were normalized to those of Renilla luciferase. To evaluate β-catenin activity, cells were transiently transfected with TOPflash (TOP) or FOPflash (FOP) reporter plasmids carrying multiple copies of a wild-type or mutated TCF-binding site, respectively. Relative activity was calculated as normalized relative light units of TOPflash divided by normalized relative light units of FOPflash. Two-tailed p-values at less than 0.05 were considered as statistically significant.
Histology, EdU, and TUNEL assay
Request a detailed protocolMouse uteri including the decidua were collected and fixed in 4% paraformaldehyde in PBS. Subsequently, paraffin blocks were made by dehydration in ethanol, clearing in xylene, and embedding in paraffin. Embryo sections 5 μm thick were cut (Microm HM 335E; Thermo Fisher Scientific) and placed on glass slides (Matsunami Glass Ind., Ltd., Osaka, Japan). For haematoxylin-eosin (HE) staining, slides were deparaffinized and rehydrated through an ethanol series, and then stained with HE.
To label the proliferating embryonic cells, pregnant mice were injected intraperitoneally with 5-ethynyl-2'-deoxyuridine (EdU) at 200 μL/mouse and sacrificed 4–6 hr later. Embryos were embedded in paraffin blocks, and sections were refixed in 4% paraformaldehyde and permeabilized in 0.5% Triton X-100/PBS. EdU assay was performed with a Click-iT Plus EdU Imaging Kit (Thermo Fisher Scientific) and TUNEL assay was performed with a Click-iT Plus TUNEL Assay for In situ Apoptosis Detection kit (Thermo Fisher Scientific) according to the manufacturer’s protocol. As the final step, embryo sections were co-stained with Hoechst 33342 or DAPI, observed under a microscope (BZ-X700; Keyence). At least two sections were counted per embryo. The total stained nuclear count was assumed as total cell number, and cell number was counted using ImageJ software. The images were processed to create grayscale type, make binary and counted by the ‘Analyze Particles’ function in ImageJ to count the positive cells.
Data availability
The RNA-seq data have been deposited in the NCBI GEO database under accession codes GSE161338.
-
NCBI Gene Expression OmnibusID GSE161338. Comparative transcriptomic analysis between wild-type (WT) and Cables2-null embryos.
References
-
Cables1 is a tumor suppressor gene that regulates intestinal tumor progression in apc(Min) miceCancer Biology & Therapy 14:672–678.https://doi.org/10.4161/cbt.25089
-
Brachyury is a target gene of the wnt/beta-catenin signaling pathwayMechanisms of Development 91:249–258.https://doi.org/10.1016/S0925-4773(99)00309-3
-
Multipotent cell lineages in early mouse development depend on SOX2 functionGenes & Development 17:126–140.https://doi.org/10.1101/gad.224503
-
Expression of members of the fgf family and their receptors during midfacial developmentMechanisms of Development 100:313–316.https://doi.org/10.1016/S0925-4773(00)00518-9
-
The p53 tumor suppressor causes congenital malformations in Rpl24-deficient mice and promotes their survivalMolecular and Cellular Biology 29:2489–2504.https://doi.org/10.1128/MCB.01588-08
-
A primary requirement for nodal in the formation and maintenance of the primitive streak in the mouseDevelopment 120:1919–1928.
-
The mouse Fgf8 gene encodes a family of polypeptides and is expressed in regions that direct outgrowth and patterning in the developing embryoDevelopment 121:439–451.
-
Staging of gastrulating mouse embryos by morphological landmarks in the dissecting microscopeDevelopment 118:1255–1266.
-
cables1 is required for embryonic neural development: molecular, cellular, and behavioral evidence from the zebrafishMolecular Reproduction and Development 78:22–32.https://doi.org/10.1002/mrd.21263
-
Expression pattern of the brachyury gene in whole-mount TWis/TWis mutant embryosDevelopment 113:913–917.
-
Hypersensitivity to DNA damage leads to increased apoptosis during early mouse developmentGenes & Development 14:2072–2084.
-
Requirement for beta-catenin in anterior-posterior Axis formation in miceJournal of Cell Biology 148:567–578.https://doi.org/10.1083/jcb.148.3.567
-
Involvement of bone morphogenetic Protein-4 (BMP-4) and Vgr-1 in morphogenesis and neurogenesis in the mouseDevelopment 111:531–542.
-
The cables gene on chromosome 18Q regulates Colon cancer progression in vivoCancer Biology & Therapy 4:861–863.https://doi.org/10.4161/cbt.4.8.1894
-
Timing of developmental events in the early mouse embryoSeminars in Cell & Developmental Biology 34:65–75.https://doi.org/10.1016/j.semcdb.2014.06.010
-
Driving ribosome assemblyBiochimica Et Biophysica Acta (BBA) - Molecular Cell Research 1803:673–683.https://doi.org/10.1016/j.bbamcr.2009.10.009
-
Enrichr: a comprehensive gene set enrichment analysis web server 2016 updateNucleic Acids Research 44:W90–W97.https://doi.org/10.1093/nar/gkw377
-
Ribosomal proteins and colorectal CancerCurrent Genomics 8:43–49.https://doi.org/10.2174/138920207780076938
-
Requirement for Wnt3 in vertebrate Axis formationNature Genetics 22:361–365.https://doi.org/10.1038/11932
-
Molecular cloning of a cyclin-like protein associated with cyclin-dependent kinase 3 (cdk 3) in vivoBiochemical and Biophysical Research Communications 273:442–447.https://doi.org/10.1006/bbrc.2000.2965
-
ik3-2, a relative to ik3-1/Cables, is involved in both p53-mediated and p53-independent apoptotic pathwaysBiochemical and Biophysical Research Communications 312:520–529.https://doi.org/10.1016/j.bbrc.2003.10.142
-
Truncated Cables1 causes agenesis of the corpus callosum in miceLaboratory Investigation 94:321–330.https://doi.org/10.1038/labinvest.2013.146
-
Beta-catenin signaling marks the prospective site of primitive streak formation in the mouse embryoDevelopmental Dynamics 231:416–424.https://doi.org/10.1002/dvdy.20135
-
Embryonic cleavage cycles: how is a mouse like a fly?Current Biology 14:R35–R45.https://doi.org/10.1016/j.cub.2003.12.022
-
Ribosomal protein S6 gene haploinsufficiency is associated with activation of a p53-dependent checkpoint during gastrulationMolecular and Cellular Biology 26:8880–8891.https://doi.org/10.1128/MCB.00751-06
-
Dose-dependent nodal/Smad signals pattern the early mouse embryoSeminars in Cell & Developmental Biology 32:73–79.https://doi.org/10.1016/j.semcdb.2014.03.028
-
Detection of mRNA in whole mounts of mouse embryos using digoxigenin riboprobesMethods in Molecular Biology 28:201–208.https://doi.org/10.1385/0-89603-254-x:201
-
Lineage allocation and asymmetries in the early mouse embryoPhilosophical Transactions of the Royal Society of London. Series B: Biological Sciences 358:1341–1349.https://doi.org/10.1098/rstb.2003.1329
-
Differential expression of multiple fork head related genes during gastrulation and axial pattern formation in the mouse embryoDevelopment 118:47–59.
-
ik3-2, a relative to ik3-1/cables, is associated with cdk3, cdk5, and c-ablBiochimica Et Biophysica Acta (BBA) - Gene Structure and Expression 1574:157–163.https://doi.org/10.1016/S0167-4781(01)00367-0
-
Nodal signaling: developmental roles and regulationDevelopment 134:1023–1034.https://doi.org/10.1242/dev.000166
-
Generalized lacZ expression with the ROSA26 cre reporter strainNature Genetics 21:70–71.https://doi.org/10.1038/5007
-
Building the mouse gastrula: signals, asymmetry and lineagesCurrent Opinion in Genetics & Development 16:419–425.https://doi.org/10.1016/j.gde.2006.06.008
-
Gene function in mouse embryogenesis: get set for gastrulationNature Reviews. Genetics 8:368–381.https://doi.org/10.1038/nrg2084
-
Aggregation chimeras: combining ES cells, diploid, and tetraploid embryosMethods in Molecular Biology 530:287–309.https://doi.org/10.1007/978-1-59745-471-1_15
-
Wnt signaling in mammalian development: lessons from mouse geneticsCold Spring Harbor Perspectives in Biology 4:a007963.https://doi.org/10.1101/cshperspect.a007963
-
Down-Regulation of ribosomal protein RPS21 inhibits invasive behavior of osteosarcoma cells through the inactivation of MAPK pathwayCancer Management and Research 12:4949–4955.https://doi.org/10.2147/CMAR.S246928
-
ik3-1/Cables is a substrate for cyclin-dependent kinase 3 (cdk 3)European Journal of Biochemistry 268:6076–6082.https://doi.org/10.1046/j.0014-2956.2001.02555.x
-
Extra-embryonic Wnt3 regulates the establishment of the primitive streak in miceDevelopmental Biology 403:80–88.https://doi.org/10.1016/j.ydbio.2015.04.008
-
Ribosomal proteins: functions beyond the ribosomeJournal of Molecular Cell Biology 7:92–104.https://doi.org/10.1093/jmcb/mjv014
-
Tumor suppressive functions of p53Cold Spring Harbor Perspectives in Biology 1:a001883.https://doi.org/10.1101/cshperspect.a001883
Article and author information
Author details
Funding
Grant-in-Aid for Scientific Research(B), Japan Society for the Promotion of Science (JSPS KAKENHI 17H03568)
- Fumihiro Sugiyama
Grant-in-Aid for Scientific Research(S), Japan Society for the Promotion of Science (JSPS KAKENHI 26221004)
- Satoru Takahashi
Grant-in-Aid for Scientific Research(C), Japan Society for the Promotion of Science (JSPS KAKENHI 17K07130)
- Hiroyoshi Iseki
Grant-in-Aid for Young Scientists (B), Japan Society for the Promotion of Science (JSPS KAKENHI 19K16020)
- Tra Thi Huong Dinh
Grant-in-Aid for Scientific Research(A), Japan Society for the Promotion of Science (JSPS KAKENHI 20H00444)
- Fumihiro Sugiyama
The Cooperative Research Project Program of Life Science Center for Survival Dynamics, Tsukuba Advanced Research Alliance (TARA Center), University of Tsukuba, Japan (182107)
- Fumihiro Sugiyama
The funders had no role in study design, data collection and interpretation, or the decision to submit the work for publication.
Acknowledgements
We thank all members of the Sugiyama Laboratory and Laboratory Animal Resource Center for helpful discussions and encouragement. Furthermore, we are indebted to T Chiba, K Kako, H Katayama, and Y Yuda for discussion and comments on this manuscript.
Ethics
Animal experimentation: Animal experiments were carried out in a humane manner with approval from the Institutional Animal Experiment Committee of the University of Tsukuba (#19-057 and #20-065) in accordance with the Regulations for Animal Experiments of the University of Tsukuba and Fundamental Guidelines for Proper Conduct of Animal Experiment and Related Activities in Academic Research Institutions under the jurisdiction of the Ministry of Education, Culture, Sports, Science, and Technology of Japan.
Version history
- Received: July 19, 2019
- Accepted: April 19, 2021
- Version of Record published: May 5, 2021 (version 1)
Copyright
© 2021, Dinh et al.
This article is distributed under the terms of the Creative Commons Attribution License, which permits unrestricted use and redistribution provided that the original author and source are credited.
Metrics
-
- 1,104
- views
-
- 103
- downloads
-
- 3
- citations
Views, downloads and citations are aggregated across all versions of this paper published by eLife.
Download links
Downloads (link to download the article as PDF)
Open citations (links to open the citations from this article in various online reference manager services)
Cite this article (links to download the citations from this article in formats compatible with various reference manager tools)
Further reading
-
- Developmental Biology
- Stem Cells and Regenerative Medicine
Amniogenesis, a process critical for continuation of healthy pregnancy, is triggered in a collection of pluripotent epiblast cells as the human embryo implants. Previous studies have established that bone morphogenetic protein (BMP) signaling is a major driver of this lineage specifying process, but the downstream BMP-dependent transcriptional networks that lead to successful amniogenesis remain to be identified. This is, in part, due to the current lack of a robust and reproducible model system that enables mechanistic investigations exclusively into amniogenesis. Here, we developed an improved model of early amnion specification, using a human pluripotent stem cell-based platform in which the activation of BMP signaling is controlled and synchronous. Uniform amniogenesis is seen within 48 hr after BMP activation, and the resulting cells share transcriptomic characteristics with amnion cells of a gastrulating human embryo. Using detailed time-course transcriptomic analyses, we established a previously uncharacterized BMP-dependent amniotic transcriptional cascade, and identified markers that represent five distinct stages of amnion fate specification; the expression of selected markers was validated in early post-implantation macaque embryos. Moreover, a cohort of factors that could potentially control specific stages of amniogenesis was identified, including the transcription factor TFAP2A. Functionally, we determined that, once amniogenesis is triggered by the BMP pathway, TFAP2A controls the progression of amniogenesis. This work presents a temporally resolved transcriptomic resource for several previously uncharacterized amniogenesis states and demonstrates a critical intermediate role for TFAP2A during amnion fate specification.
-
- Developmental Biology
- Neuroscience
Axon projection is a spatial- and temporal-specific process in which the growth cone receives environmental signals guiding axons to their final destination. However, the mechanisms underlying changes in axonal projection direction without well-defined landmarks remain elusive. Here, we present evidence showcasing the dynamic nature of axonal projections in Drosophila’s small ventral lateral clock neurons (s-LNvs). Our findings reveal that these axons undergo an initial vertical projection in the early larval stage, followed by a subsequent transition to a horizontal projection in the early-to-mid third instar larvae. The vertical projection of s-LNv axons correlates with mushroom body calyx expansion, while the s-LNv-expressed Down syndrome cell adhesion molecule (Dscam1) interacts with Netrins to regulate the horizontal projection. During a specific temporal window, locally newborn dorsal clock neurons secrete Netrins, facilitating the transition of axonal projection direction in s-LNvs. Our study establishes a compelling in vivo model to probe the mechanisms of axonal projection direction switching in the absence of clear landmarks. These findings underscore the significance of dynamic local microenvironments in the complementary regulation of axonal projection direction transitions.