Polyunsaturated fatty acid analogues differentially affect cardiac NaV, CaV, and KV channels through unique mechanisms
Figures
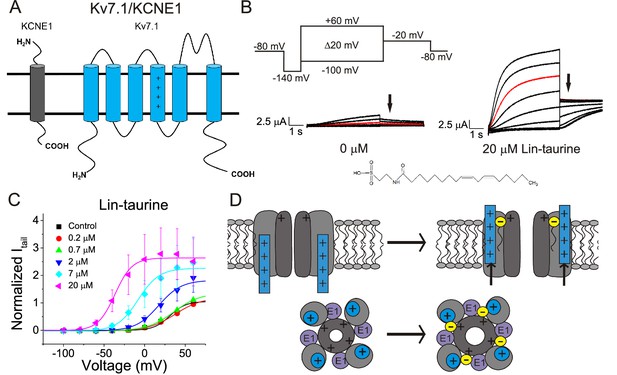
PUFA analogue, Linoleoyl-taurine, activates Kv7.1/KCNE1 channels through an electrostatic mechanism on voltage sensor and pore.
(A) Simplified membrane topology of a single Kv7.1 α-subunit (blue) and a single KCNE1 β-subunit (grey). (B) Voltage protocol used to measure voltage dependence of activation and representative Kv7.1/KCNE1 current traces in control (0 μM) and 20 μM Lin-taurine. Arrows mark tail currents. (C) Current-voltage relationship demonstrating PUFA analogue-induced left-shift in the voltage-dependence of activation (V0.5) and increase in maximal conductance (Gmax) (mean ± SEM; n = 3). (D) Model in which PUFA analogues with their negatively charged head groups insert in the cell membrane close to the positively charged arginines in the voltage sensor S4 and close to a positively charged lysine in the pore. The PUFA thereby exerts an electrostatic effect on the voltage sensor to shift the voltage dependence of activation and an electrostatic effect on the pore to increase the maximum conductance (Liin et al., 2018a).
-
Figure 1—source data 1
Effects of lin-taurine on cardiac ion channels.
- https://cdn.elifesciences.org/articles/51453/elife-51453-fig1-data1-v2.docx
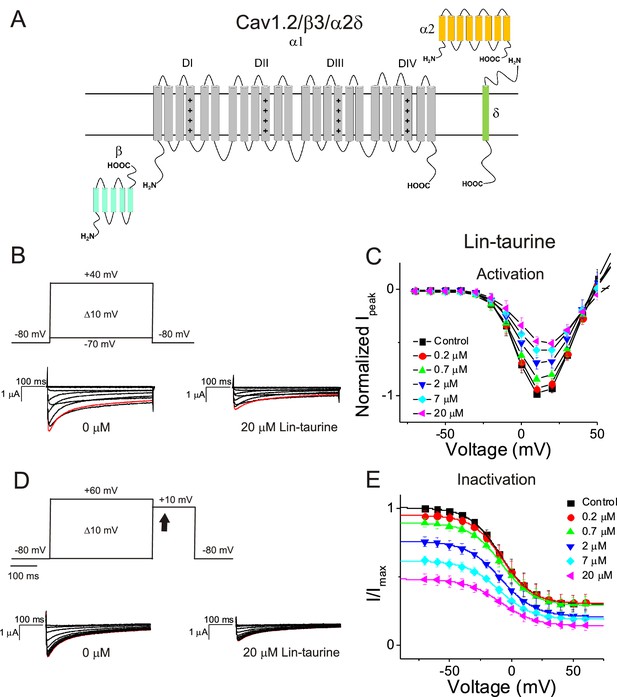
PUFA analogue, Linoleoyl-taurine, inhibits Cav1.2/β3/α2δ channels without altering channel voltage dependence.
(A) Simplified membrane topology of the Cav1.2 pore-forming α-subunit (light gray) and auxiliary β- (mint) and α2δ-subunits (yellow and green). (B) Voltage protocol used to measure voltage dependence of activation and representative Cav1.2/β3/α2δ current traces in control (0 μM) and 20 μM Lin-taurine. (C) Current-voltage relationship demonstrating dose-dependent inhibition of Cav1.2/β3/α2δ currents measured from activation protocol (mean ± SEM; n = 5). (D) Voltage protocol used to measure voltage dependence of inactivation and representative Cav1.2/β3/α2δ current traces in control (0 μM) and 20 μM Lin-taurine measured at arrow. (E) Current-voltage relationship demonstrating dose-dependent inhibition of Cav1.2/β3/α2δ currents measured from inactivation protocol (mean ± SEM; n = 5). See Figure 2—figure supplement 1 for comparison with effects on Cav1.2/β2/α2δ.
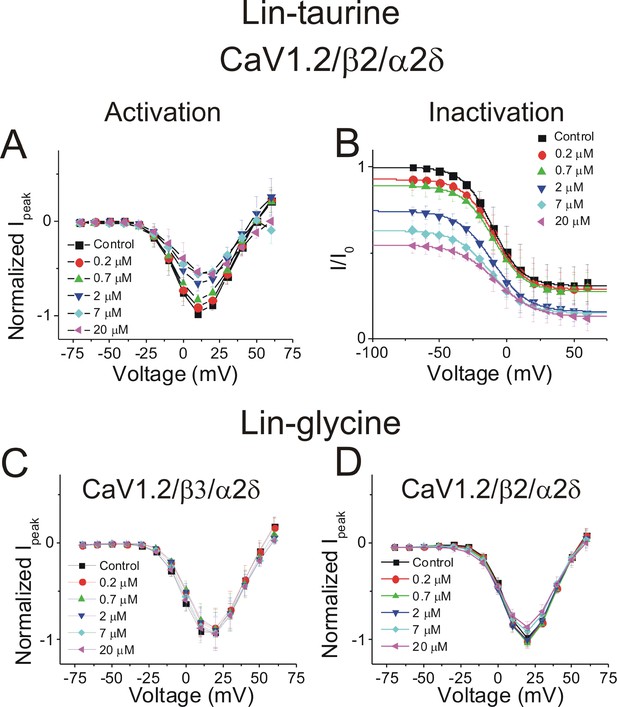
PUFA-induced effects on Cav1.2 expressed with β-subunit β2 and α2δ (Cav1.2/β2/α2δ).
(A) Voltage dependence of activation for Cav1.2/β2/α2δ in the presence of Lin-taurine. (B) Voltage dependence of inactivation for Cav1.2/β2/α2δ in the presence of Lin-taurine. (C–D) Comparison between the voltage dependence of activation for (C) Cav1.2/β3/α2δ and (D) Cav1.2/β2/α2δ in the presence of Lin-glycine.
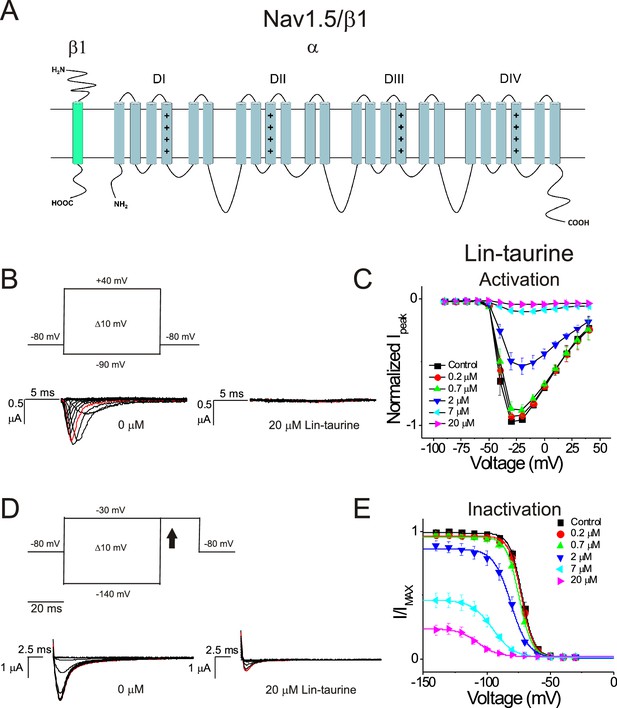
PUFA analogue, Linoleoyl-taurine, inhibits Nav1.5/β1 by shifting the voltage dependence of inactivation.
(A) Simplified membrane topology of the Nav1.5 pore-forming α-subunit (light blue) and auxiliary β-subunit (green). (B) Voltage protocol used to measure voltage dependence of activation and representative Nav1.5/β1 current traces in control (0 μM) and 20 μM Lin-taurine. (C) Current-voltage relationship demonstrating dose-dependent inhibition of Nav1.5/β1 currents measured from activation protocol (mean ± SEM; n = 5). (D) Voltage protocol used to measure voltage dependence of inactivation and representative Nav1.5/β1 current traces in control (0 μM) and 20 μM Lin-taurine measured at arrow. (E) Current-voltage relationship demonstrating dose-dependent inhibition of Nav1.5/β1 currents and leftward shift in the voltage dependence of inactivation measured from inactivation protocol (mean ± SEM; n = 5).
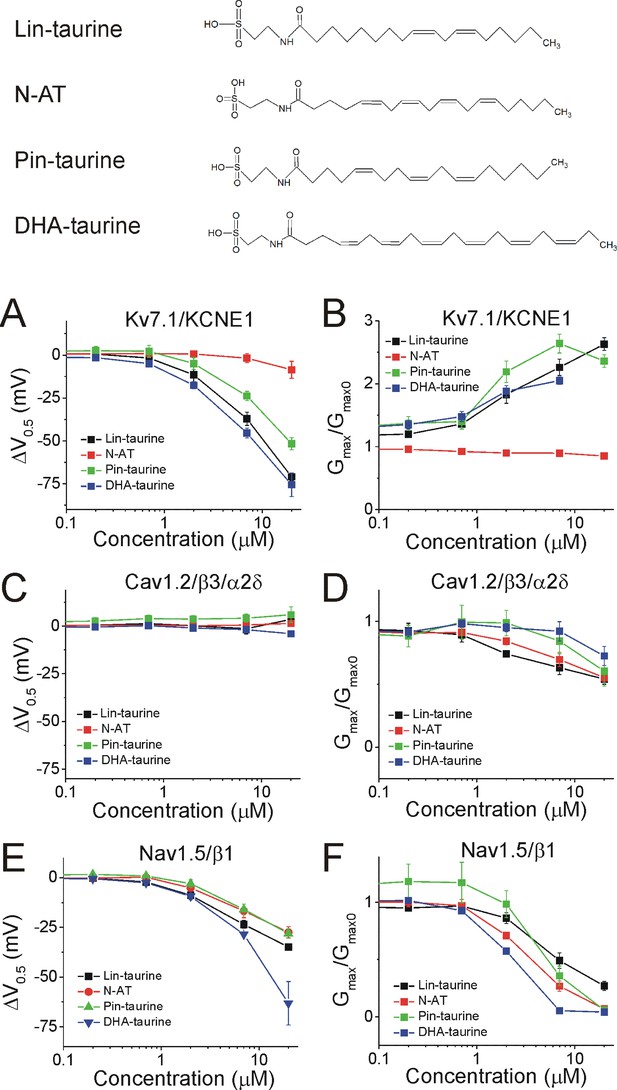
PUFA analogues with taurine head groups have the tendency to broadly modulate Kv7.1/KCNE1, Cav1.2/β3/α2δ, and Nav1.5/β1 channels.
Structures for PUFA analogues with taurine head groups: Lin-taurine, N-AT, Pin-taurine, and DHA-taurine (top). (A–B) Dose dependent shifts in the (A) voltage dependence of activation (ΔV0.5) and (B) changes in maximal conductance (Gmax) for Kv7.1/KCNE1 induced by Lin-taurine (black squares; n = 3; mean ± SEM), N-AT (red circles; n = 5; mean ± SEM), Pin-taurine (green triangles; n = 4; mean ± SEM), and DHA-taurine (blue triangles; n = 3; mean ± SEM). (C–D) Dose dependent shifts in the (C) voltage dependence of inactivation (ΔV0.5) and (D) changes in maximal conductance (Gmax) for Cav1.2/β3/α2δ induced by Lin-taurine (black squares; n = 3; mean ± SEM), N-AT (red circles; n = 3; mean ± SEM), Pin-taurine (green triangles; n = 5; mean ± SEM), and DHA-taurine (blue triangles; n = 3; mean ± SEM). (E–F) Dose dependent shifts in the (E) voltage dependence of inactivation (ΔV0.5) and (F) changes in maximal conductance (Gmax) for Nav1.5/β1 induced by Lin-taurine (black squares; n = 3; mean ± SEM), N-AT (red circles; n = 3; mean ± SEM), Pin-taurine (green triangles; n = 4; mean ± SEM), and DHA-taurine (blue triangles; n = 3; mean ± SEM). See Figure 4—figure supplements 1–6 for more details.
-
Figure 4—source data 1
Effects of N-AT on cardiac ion channels.
- https://cdn.elifesciences.org/articles/51453/elife-51453-fig4-data1-v2.docx
-
Figure 4—source data 2
Effects of pin-taurine on cardiac ion channels.
- https://cdn.elifesciences.org/articles/51453/elife-51453-fig4-data2-v2.docx
-
Figure 4—source data 3
Effects of DHA-taurine on cardiac ion channels.
- https://cdn.elifesciences.org/articles/51453/elife-51453-fig4-data3-v2.docx
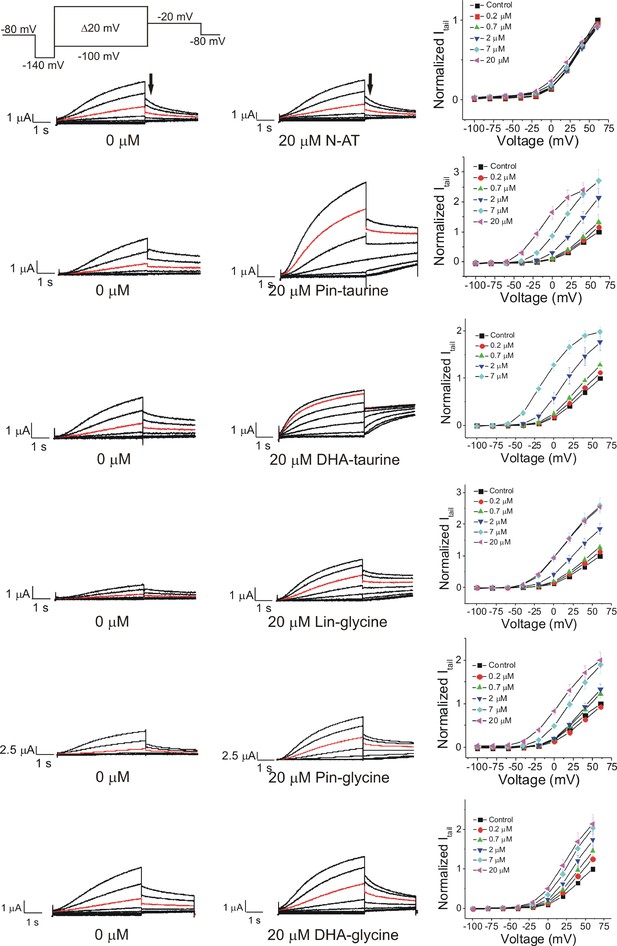
Raw current traces for PUFA analogues on Kv7.1/KCNE1.
Kv7.1/KCNE1 raw current traces in control (0 μM) (left) and 20 μM (middle) PUFA analogues, followed by current-voltage (I–V) relationship (right) for each PUFA analogue tested. Voltage protocol (top) overlaps with current traces to illustrate time points during the voltage steps. Arrows over top trace represent the point at which tail currents are measured for generation of current-voltage (I–V) relationship. Red traces represent the current that occurs at a voltage step to 20 mV.
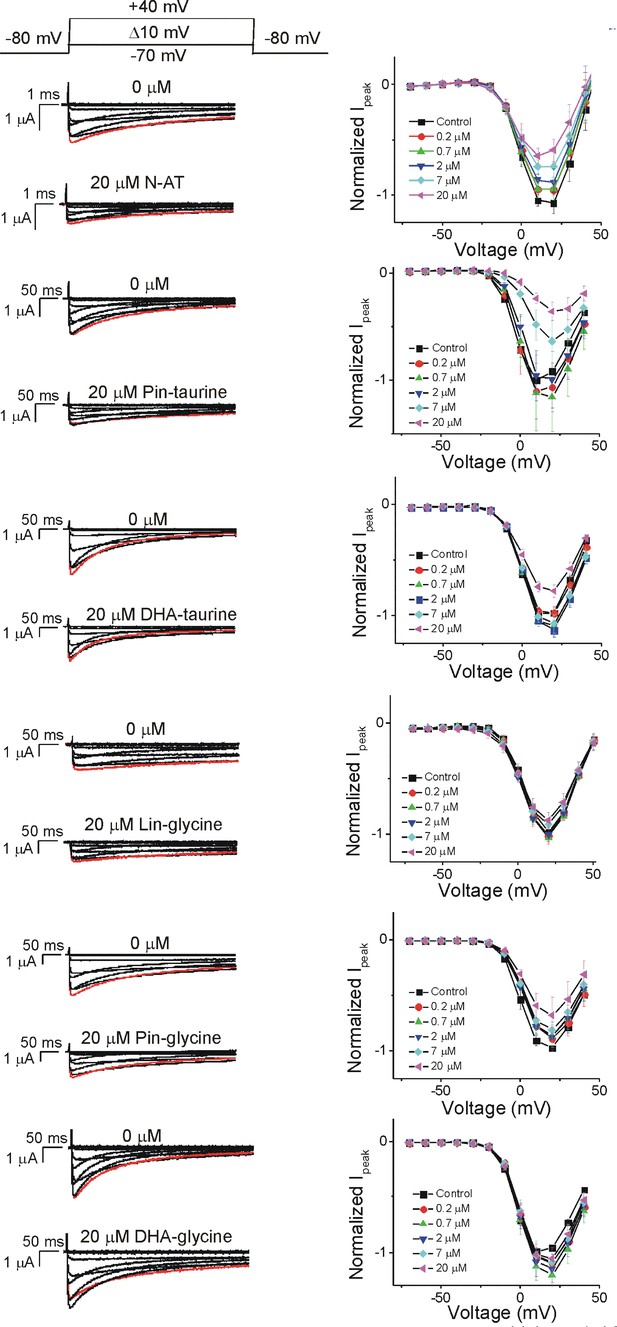
Raw current traces for PUFA analogues on Cav1.2/β3/α2δ.
Cav1.2/β3/α2δ raw current traces in control (0 μM) (top trace for each PUFA analogue) and 20 μM (bottom trace for each PUFA analogue), followed by current-voltage (I–V) relationship (right) of voltage dependent activation for each PUFA analogue tested. Voltage protocol (top) overlaps with current traces to illustrate time points during the voltage steps. Red traces represent the voltage step that elicits peak current.
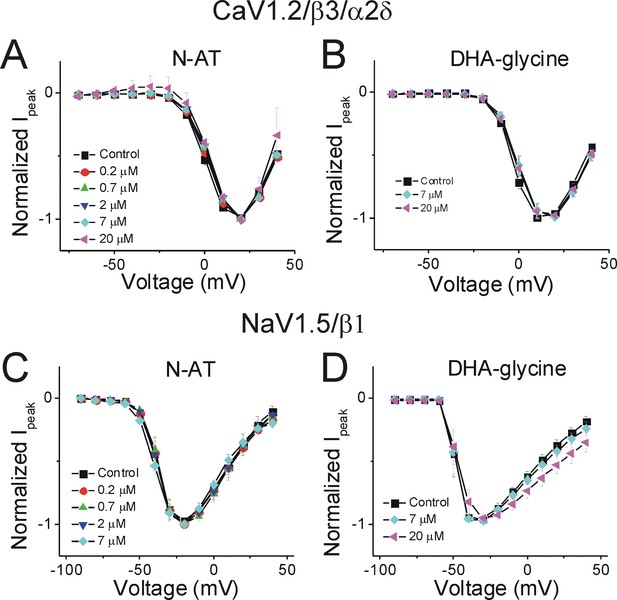
PUFA-induced changes in I/I0 normalized by concentration show no changes in voltage-dependent activation of Cav1.2/β3/α2δ and Nav1.5/β1 channels.
(A–B) Voltage-dependent activation of Cav1.2/β3/α2δ in the presence of (A) N-AT (n = 3) and (B) DHA-glycine (n = 3). Peak currents are normalized to each concentration to clearly visualize that there is no shifts in voltage-dependent activation. (C–D) Voltage-dependent activation of Nav1.5/β1 in the presence of (C) N-AT (n = 3) and (D) DHA-glycine (n = 7). Peak currents are normalized to each concentration to clearly visualize that there are no shifts in voltage-dependent activation.
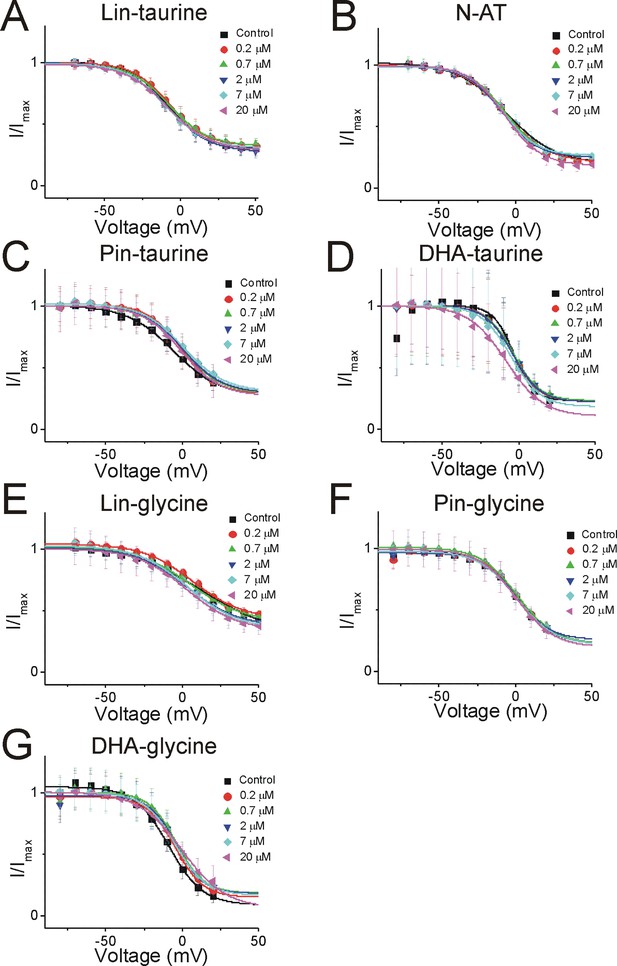
Internally normalized steady state inactivation curves for Cav1.2/β3/α2δ.
(A–G) PUFA-induced effects on Nav1.5/β1 voltage dependent inactivation normalized by each concentration for: (A) Lin-taurine, (B) N-AT, (C) Pin-taurine, (D) DHA-taurine, (E) Lin-glycine, (F) Pin-glycine, and (G) DHA-glycine.
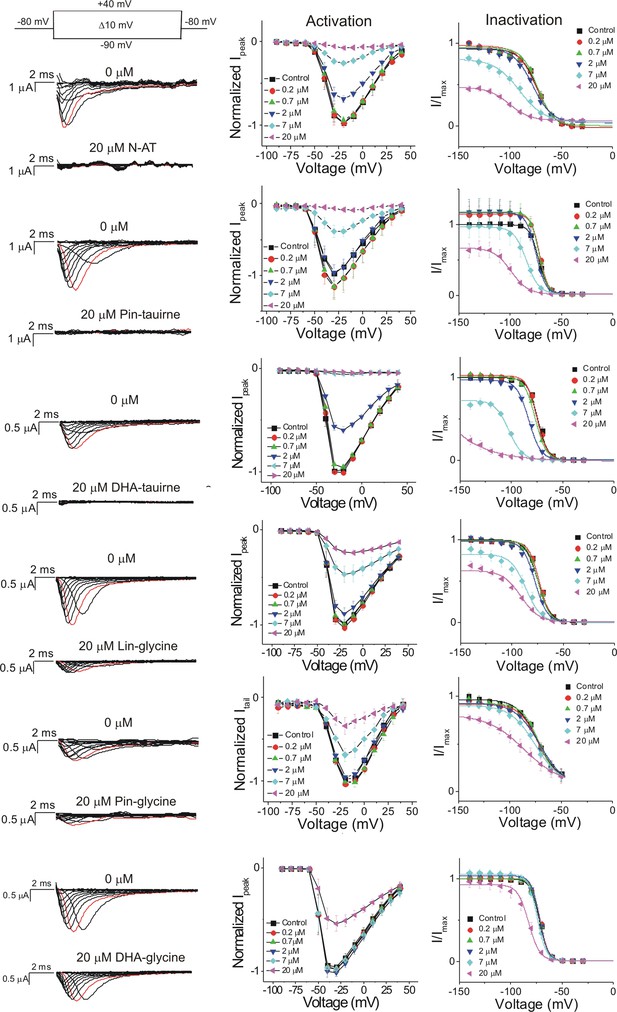
Raw current traces for PUFA analogues on Nav1.5/β1 channels.
Nav1.5/β1 raw current traces in control (0 μM) (top trace for each PUFA analogue) and 20 μM (bottom trace for each PUFA analogue) of applied PUFA analogues, followed by current-voltage (I–V) relationship for voltage dependent activation (middle) and I-V relationship for voltage dependent inactivation (right) for each PUFA analogue tested. Voltage protocol (top) overlaps with current traces to illustrate time points during the voltage steps. Red traces represent the voltage step that elicits peak current.
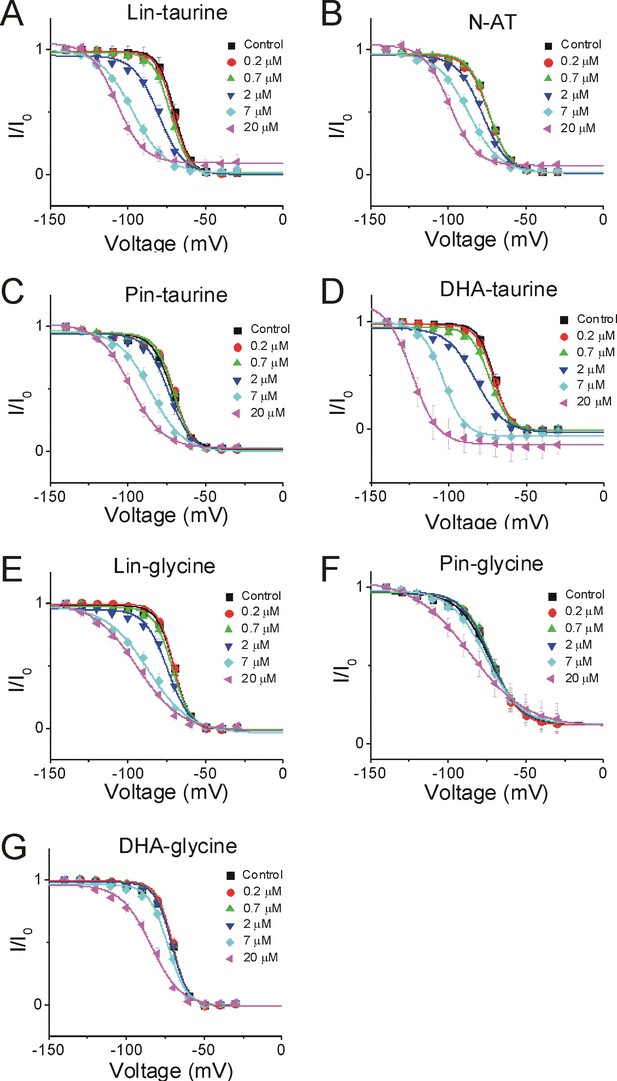
Internally normalized steady state inactivation curves for Nav1.5/β1.
(A–G) PUFA-induced effects on Nav1.5/β1 voltage dependent inactivation normalized by each concentration for: (A) Lin-taurine, (B) N-AT, (C) Pin-taurine, (D) DHA-taurine, (E) Lin-glycine, (F) Pin-glycine, and (G) DHA-glycine.
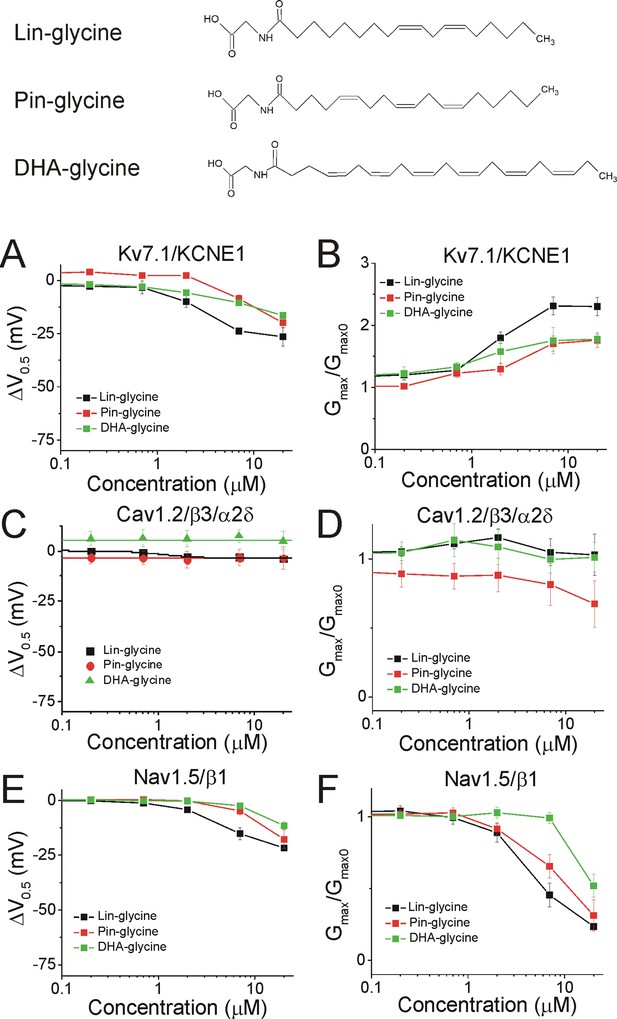
PUFA analogues with glycine head groups tend to be more selective for Kv7.1/KCNE1 than Cav1.2/β3/α2δ and Nav1.5/β1 channels.
Structures for PUFA analogues with glycine head group: Lin-glycine, Pin-glycine, and DHA-glycine (top). (A–B) Dose dependent shifts in the (A) voltage dependence of activation (ΔV0.5) and (B) changes in maximal conductance (Gmax) for Kv7.1/KCNE1 induced by Lin-glycine (black squares; n = 4; mean ± SEM), Pin-glycine (red circles; n = 3; mean ± SEM), and DHA-glycine (green triangles; n = 4; mean ± SEM). (C–D) Dose dependent shifts in the (C) voltage dependence of inactivation (ΔV0.5) and (D) changes in maximal conductance (Gmax) for Cav1.2/β3/α2δ induced by Lin-glycine (black squares; n = 4; mean ± SEM), Pin-glycine (red circles; n = 3; mean ± SEM), and DHA-glycine (green triangles; n = 3; mean ± SEM). (E–F) Dose dependent shifts in the (E) voltage dependence of inactivation (ΔV0.5) and (F) changes in maximal conductance (Gmax) for Nav1.5/β1 induced by Lin-glycine (black squares; n = 4; mean ± SEM), Pin-glycine (red circles; n = 4; mean ± SEM), and DHA-glycine (green triangles; n = 7; mean ± SEM). See Figure 4—figure supplements 1–6 for more details.
-
Figure 5—source data 1
Effects of lin-glycine on cardiac ion channels.
- https://cdn.elifesciences.org/articles/51453/elife-51453-fig5-data1-v2.docx
-
Figure 5—source data 2
Effects of pin-glycine on cardiac ion channels.
- https://cdn.elifesciences.org/articles/51453/elife-51453-fig5-data2-v2.docx
-
Figure 5—source data 3
Effects of DHA-glycine on cardiac ion channels.
- https://cdn.elifesciences.org/articles/51453/elife-51453-fig5-data3-v2.docx
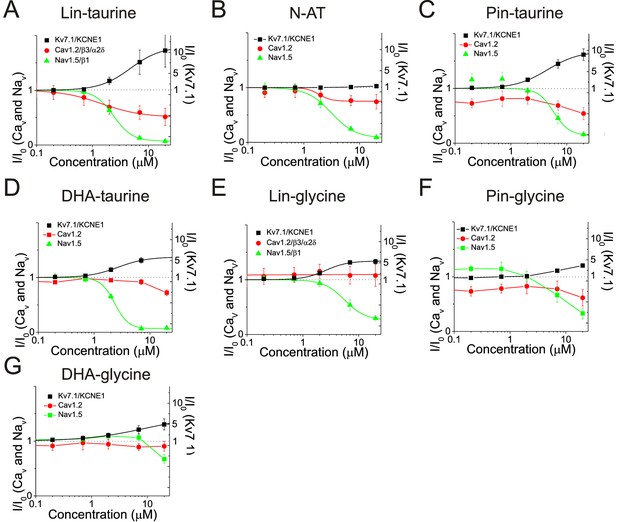
Dose response curves for PUFAs on IKs, ICaL, and INaV at 0 mV.
Dose response of IKs, ICaL, and INaV currents (I/I0) at 0 mV for (A) Lin-taurine (Km of IKs = 11.4 ± 0.4 μM; Km of ICaL = 1.4 ± 0.4 μM; Km of INaV = 2.4 ± 0.04 μM; mean ± SEM), (B) N-AT (Km of IKs = NA; Km of ICaL = 2.5 ± 1.9 μM; Km of INaV = 3.1 ± 0.3 μM; mean ± SEM), (C) Pin-taurine (Km of IKs = 4.5 ± 0.2 μM; Km of ICaL = NA; Km of INaV = 5.8 ± 1.7 μM; mean ± SEM), (D) DHA-taurine (Km of IKs = 5.9 ± 0.3 μM; Km of ICaL >20 μM; Km of INaV = 2.3 ± 0.1 μM; mean ± SEM), (E) Lin-glycine (Km of IKs = 5.4 ± 0.2 μM; Km of ICaL = NA; Km of INaV = 5.6 ± 0.5 μM; mean ± SEM), (F) Pin-glycine (Km of IKs > 20 μM; Km of ICaL = NA; Km of INaV >20 μM; mean ± SEM), and (G) DHA-glycine (Km of IKs > 20 μM; Km of ICaL = NA; Km of INaV >20 μM; mean ± SEM),.
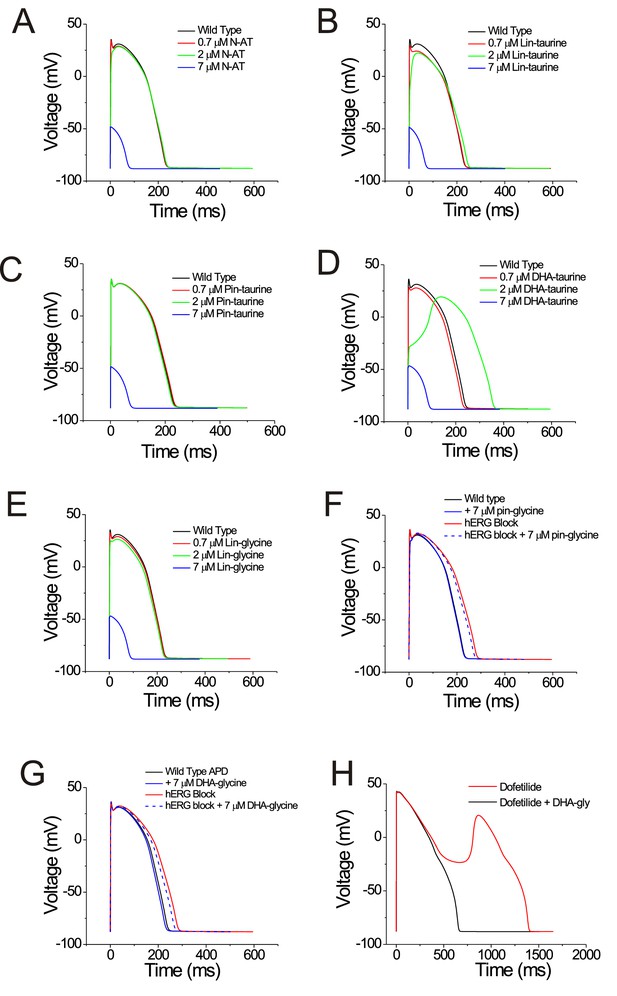
PUFAs that are selective for Kv7.1/KCNE1 channels partially restore prolonged ventricular action potential and suppress early afterdepolarizations in cardiomyocyte simulations.
(A–G) Simulated ventricular action potential in wild type cardiomyocytes (black) and in the presence of (A) 0.7 (red), 2 (green), and 7 μM N-AT (blue), (B) 0.7 (red), 2 (green), and 7 μM lin-taurine (blue), (C) 0.7 (red), 2 (green), and 7 μM pin-taurine (blue), (D) 0.7 (red), 2 (green), and 7 μM DHA-taurine (blue), (E) 0.7 (red), 2 (green), and 7 μM lin-glycine (blue), (F) 7 μM pin-glycine (blue solid), following 25% hERG block (red) and in the presence of 7 μM pin-glycine under 25% hERG block (blue dashed), and (G) 7 μM DHA-glycine (blue solid), following 25% hERG block (red) and in the presence of 7 μM DHA-glycine under 25% hERG block (blue dashed). (H) Early afterdepolarizations induced by dofetilide application (red) and suppression of early afterdepolarizations by 7 μM DHA-glycine in the presence of dofetilide (black). See Figure 7—figure supplement 1 for rate dependence of the effects.
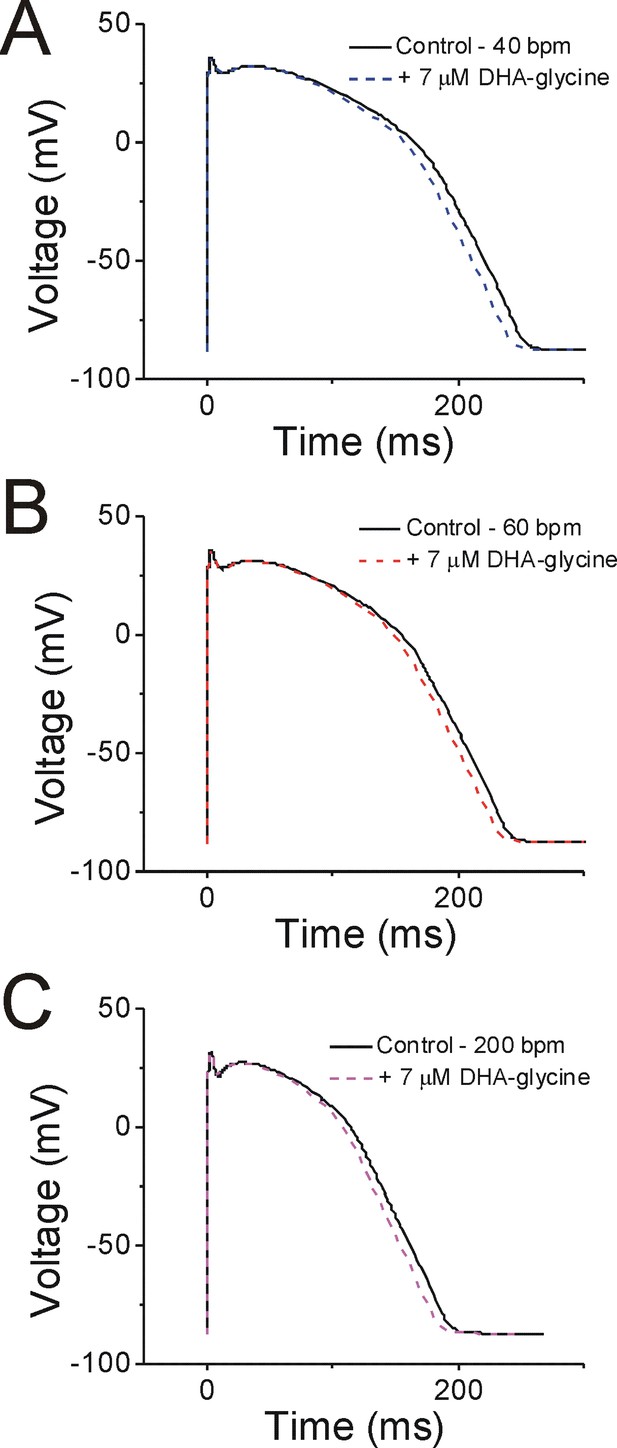
Rate dependence of the simulated ventricular action potential and effects of DHA-glycine.
(A) Simulations of the ventricular action potential at 40 bpm in control conditions (black) and simulated effects of 7 µM DHA-glycine (blue dashed line). (B) Simulations of the ventricular action potential at 60 bpm in control conditions (black) and simulated effects of 7 µM DHA-glycine (red dashed line). (C) Simulations of the ventricular action potential at 200 bpm in control conditions (black) and simulated effects of 7 µM DHA-glycine (magenta dashed line).
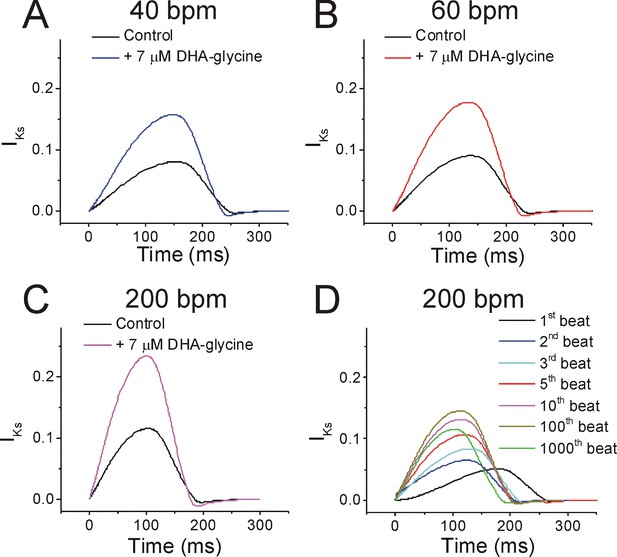
Rate dependence of the IKs current during simulated ventricular action potentials and effects of DHA-glycine.
(A) Simulations of the IKs current during the ventricular action potential at 40 bpm in control conditions (black line) and in 7 µM DHA-glycine (dark blue line). (B) Simulations of the IKs current during the ventricular action potential at 60 bpm in control conditions (black) and in 7 µM DHA-glycine (red dashed line). (C) Simulations of the IKs current during the ventricular action potential at 200 bpm in control conditions (black) and in 7 µM DHA-glycine (magenta dashed line). (D) IKs currents during simulation of the ventricular action potential at 200 bpm in control conditions after the indicated number of beats.
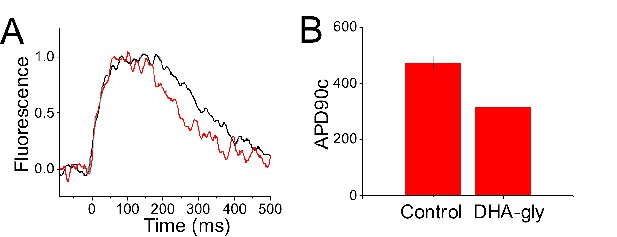
DHA-glycine decreases the APD90c of hiPSC cardiomyocytes.
(A) Normalized representative CaT optical traces before (black) and after applied (red) 30 µM DHA-glycine on a monolayer of hiPSC-CM. (B) APD90c (ms) value in control conditions (black) and after applied (red) 30 µM DHA-glycine on hiPSC-CM (mean ± SEM; n = 3). *p<0.05.
Tables
Summary of PUFA effects on current and apparent affinity.
I/I0 represents the relative current of the specified channel. The Km indicates the concentration at which half the maximal effect on I/I0 occurs and is used as a measure of the apparent affinity of the PUFA analogue. Data is represented as mean ± SEM. Comparisons were made using One-way ANOVA and Student’s t-test. Significance level is set to p=0.05.
PUFA Analogue | IKs I/I0 (7 μM) (mean ± SEM) | Km IKs (μM) (mean ± SEM) | Adj R2 of Hill Fit | ICa I/I0 (7 μM) (mean ± SEM) | Km ICa (μM) (mean ± SEM) | Adj R2 of Hill Fit | INa I/I0 (7 μM) (mean ± SEM) | Km INa (μM) (mean ± SEM) | Adj R2 of Hill Fit |
---|---|---|---|---|---|---|---|---|---|
Lin-taurine | 7.7 ± 2.9 (p=0.14) | 11.4 ± 0.4 | 0.99 | 0.6 ± 0.1 (p=0.01) | 1.4 ± 0.4 | 0.98 | 0.1 ± 0.02 (p=0.0001) | 2.4 ± 0.04 | 0.99 |
N-AT | 1.12 ± 0.1 (p=0.27) | NA | NA | 0.8 ± 0.1 (p=0.1) | 2.5 ± 1.9 | 0.70 | 0.2 ± 0.01 (p=0.001) | 3.1 ± 0.3 | 0.99 |
Pin-taurine | 6.8 ± 1.0 (p=0.01) | 4.5 ± 0.2 | 0.99 | 0.7 ± 0.1 (p=0.02) | NA | NA | 0.5 ± 0.1 (p=0.01) | 5.8 ± 1.7 | 0.89 |
DHA-taurine | 5.1 ± 0.7 (p=0.03) | 5.9 ± 0.3 | 0.99 | 0.9 ± 0.1 (p=0.23) | >20 | 0.75 | 0.07 ± 0.01 (p=0.0001) | 2.3 ± 0.1 | 0.99 |
Lin-glycine | 5.1 ± 0.4 (p=0.002) | 5.4 ± 0.2 | 0.99 | 1.1 ± 0.2 (p=0.4) | NA | NA | 0.5 ± 0.1 (p=0.02) | 5.6 ± 0.5 | 0.96 |
Pin-glycine | 2.5 ± 0.2 (p=0.02) | >20 | 0.98 | 0.8 ± 0.1 (p=0.26) | NA | NA | 0.7 ± 0.1 (p=0.09) | >20 | 0.90 |
DHA-glycine | 3.7 ± 1.0 (p=0.07) | >20 | 0.99 | 0.9 ± 0.1 (p=0.19) | NA | NA | 1.1 ± 0.05 (p=0.24) | >20 | 1 |
Fitted slope factor of conductance vs voltage relationship of Kv7.1/KCNE1 + PUFA analogues.
PUFA Analogue | Control | 0.2 mM | 0.7 mM | 2 mM | 7 mM | 20 mM |
---|---|---|---|---|---|---|
Lin-taurine | 17.5 ± 0.75 mV | 17.7 ± 0.76 mV | 17.7 ± 0.77 mV | 18.6 ± 1.0 mV | 18.1 ± 1.0 mV | 12.2 ± 1.8 mV |
N-AT | 16.7 ± 0.71 mV | 16.9 ± 0.65 mV | 17.2 ± 0.62 mV | 17.4 ± 0.60 mV | 18.2 ± 0.59 mV | 18.7 ± 0.73 mV |
Pin-taurine | 17.7 ± 0.67 mV | 17.9 ± 0.82 mV | 17.7 ± 0.82 mV | 18.3 ± 0.96 mV | 18.8 ± 1.0 mV | 16.8 ± 0.73 mV |
DHA-taurine | 18.7 ± 1.2 mV | 18.7 ± 1.1 mV | 18.6 ± 1.2 mV | 18.6 ± 1.3 mV | 18.1 ± 1.2 mV | NA |
Lin-glycine | 18.4 ± 1.2 mV | 18.2 ± 0.89 mV | 19.0 ± 1.0 mV | 19.2 ± 1.2 mV | 20.6 ± 1.5 mV | 22.1 ± 1.7 mV |
Pin-glycine | 18.4 ± 0.70 mV | 17.9 ± 0.83 mV | 17.8 ± 0.84 mV | 17.9 ± 0.91 mV | 18.7 ± 1.1 mV | 20.9 ± 1.4 mV |
DHA-glycine | 16.7 ± 0.44 mV | 16.5 ± 0.49 mV | 16.6 ± 0.45 mV | 16.6 ± 0.52 mV | 17.0 ± 0.56 mV | 17.9 ± 0.68 mV |