Functionally heterogeneous human satellite cells identified by single cell RNA sequencing
Figures
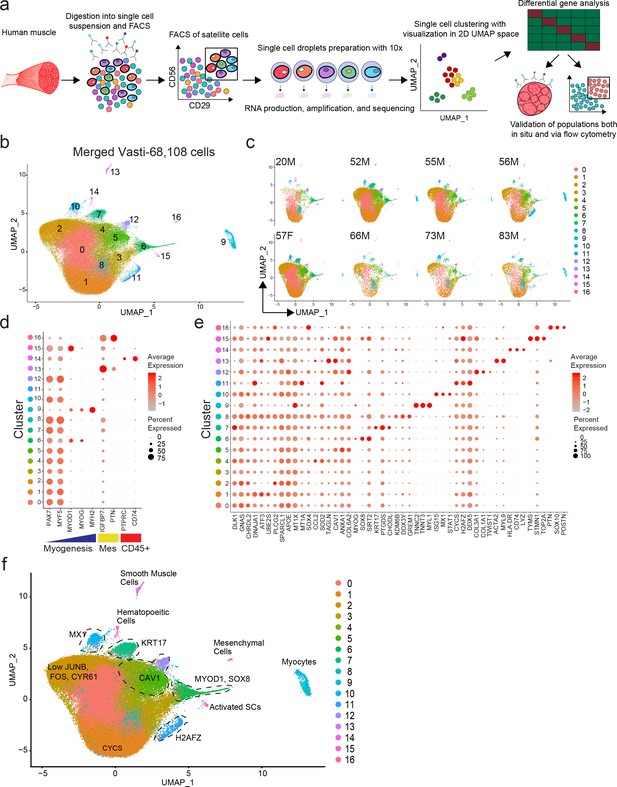
Single cell RNA sequencing reveals heterogeneity of the human satellite cell transcriptome.
(a) Schematic diagram of the process of isolating human satellite cells from muscle biopsies, and then performing single cell RNA-seq to develop single cell transcriptomes leading to discovery of cell clusters, followed by in vivo validation. (b) UMAP plot of 68,108 cells isolated from eight vasti lateralis of male and female healthy subjects. Cells are clustered according to transcriptome similarity in 2D space. Each dot represents one cell, which are colored by cluster as identified by clustering analysis. (c) UMAP of each individual sample showing the distribution of cells in clusters for each sample. (d–f) Dot plots displaying expression of individual genes within each cluster. Each cluster is depicted on the y-axis and genes are labeled on the x-axis. Larger dot size represents more cells of that cluster expressing each gene; while color indicates the level of expression within those cells. (d) Dot plot displaying expression of genes associated with myogenesis, mesenchymal and hematopoietic markers in clusters 0–16. (e) Dot plot displaying the expression of top differentially expressed cluster markers in clusters 0–16. (f) ‘Road-UMAP’ with labeled cell types and key significantly regulated markers.
-
Figure 1—source data 1
Single cell RNA sequencing reveals heterogeneity of the human satellite cell transcriptome.
- https://cdn.elifesciences.org/articles/51576/elife-51576-fig1-data1-v2.pdf
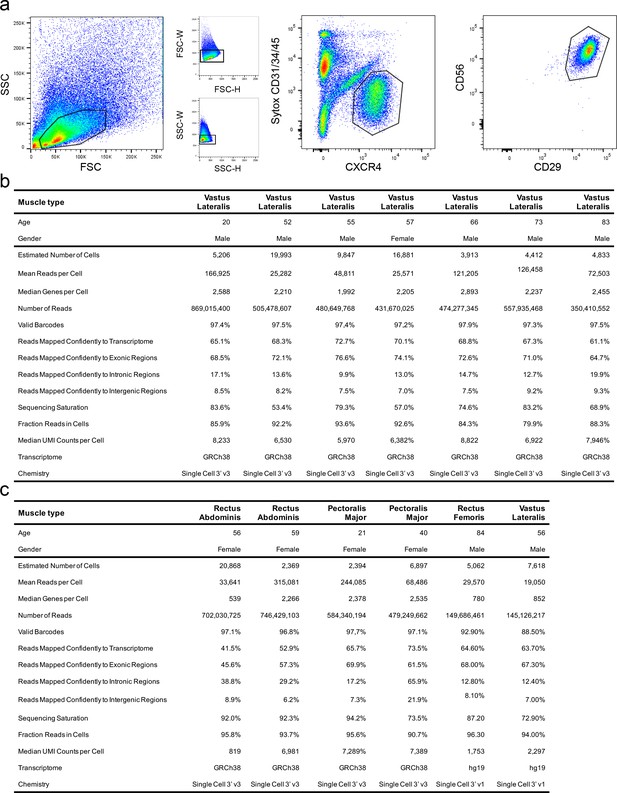
Flow cytometric sorting of CXCR4+/CD29+/CD56+ human satellite cells and quality control data from single cell RNA-sequencing experiments.
(a) Flow cytometry profiles from the isolation of CXCR4/CD29/CD56 human satellite cells from vastus lateralis muscle of a 55 year old male. (b–c) Quality control metrics for each of the single cell RNA-sequencing experiments of cells isolated from an 84 year old male (left) and a 56 year old male (right).
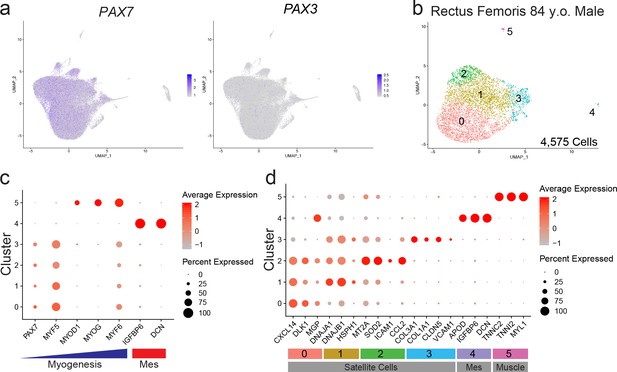
PAX7/3 expression in vasti lateralis and transcriptome from a rectus femoris muscle of human satellite cells.
(a) Feature map displaying the expression of PAX7 and PAX3 of satellite cells in eight pooled vasti lateralis human muscle. Of Note while the rectus femoris sample was prepared with the Chromium Single Cell 3' Reagent v1 kit, we found that the newer 3' Reagent v3 detected some genes including PAX7 with greater sensitivity. (b) UMAP of 5,062 cells isolated from the quadriceps muscle of a 84-year-old male. Cells are clustered according to transcriptome similarity in 2D space. Each dot represents one cell which are colored by cluster as identified by clustering analysis. (c) Dot plots displaying expression of individual genes within each cluster. Each cluster is depicted on the y-axis and genes are labeled on the x-axis. Larger dot size represents more cells of that cluster expressing each gene; while color indicates the over level of expression within those cells. (d) Dot plot displaying expression of genes associated with myogenesis and mesenchymal markers in clusters 0–5. (e) Dot plot displaying the expression of top differentially expressed cluster markers in clusters 0–5 similar to the eight vasti lateralis.
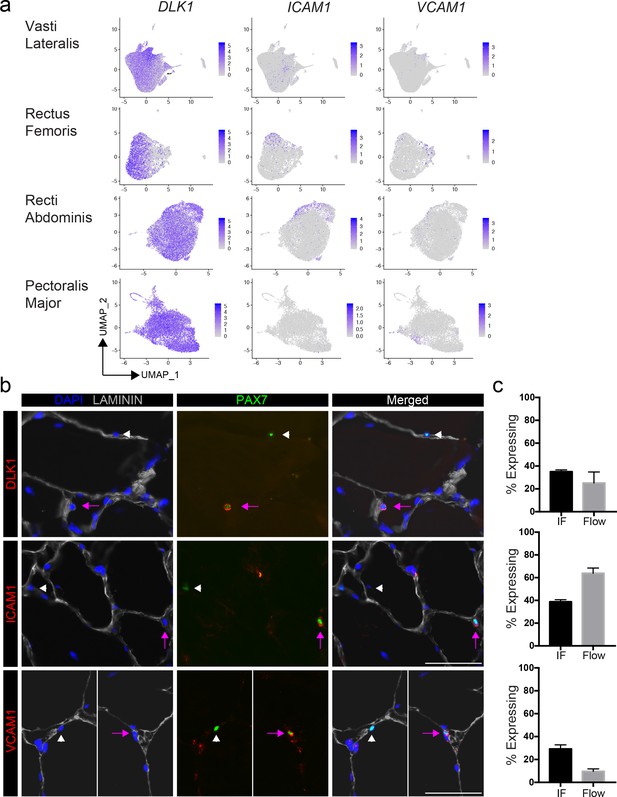
Validation of satellite cell clusters.
(a) Feature plots displaying localized gene expression for the genes DLK1, ICAM1, and VCAM1 within the 2D UMAP space of the eight combined vasti, the rectus femoris, the two combined rectus abdominis and the two combined pectoralis majors as shown in Figure 1b and Figure 1—figure supplement 2b, Figure 2—figure supplement 1a,b. Each dot represents a single cell. Deeper purple coloration represents increased expression. (b) Immunofluorescence staining of human satellite cells for validation markers within sections of human muscle. Pink arrows denote human satellite cells that are positive for the marker (scale, 50 µm). White arrowheads mark satellite cells that are negative for expression of the marker. (n = 3, biological replicates). (c) Bar plot displaying the quantification of DLK1, ICAM1, and VCAM1 expression of human satellite cells with both immunofluorescence staining and flow cytometry. (n = 3, biological replicates). Data presented as mean ± SEM.
-
Figure 2—source data 1
Validation of satellite cell clusters.
- https://cdn.elifesciences.org/articles/51576/elife-51576-fig2-data1-v2.pdf

Validation of satellite cell cluster marker CYCS across multiple human muscle type.
(a-b) UMAP plot of cells isolated from two recti abdominis and two pectoralis major. (c) Feature plot depicting localized gene expression of cluster marker CYCS in the UMAP 2D space in the eight vasti lateralis, rectus femoris, two recti abdominis and two pectoralis major. (d) Immunofluorescence staining of human satellite cells for CYCS within sections of human muscle (scale, 50 µm). Lavender arrows denote human satellite cells that are positive for CYCS. White arrowheads mark satellite cells that are negative for CYCS (n = 3, biological replicates). (e) Bar plot displaying the quantification CYCS expression of PAX7+ human satellite cells by immunofluorescence staining (n = 3, biological replicates). Data presented as mean ± SEM.
-
Figure 2—figure supplement 1—source data 1
Validation of satellite cell cluster marker CYCS across multiple human muscle type.
- https://cdn.elifesciences.org/articles/51576/elife-51576-fig2-figsupp1-data1-v2.ai
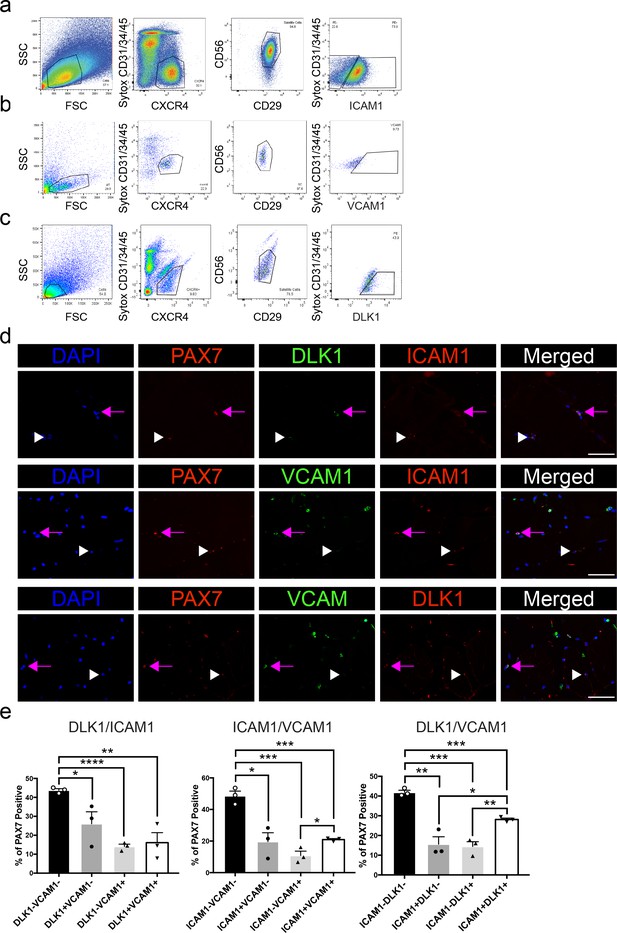
Heterogeneous expression of ICAM1, VCAM1 and DLK1 in human satellite cells.
(a-c) Representative flow cytometry profiles for CXCR4+/CD29+/CD56+ human satellite cells expressing (a) ICAM1, (b) VCAM1, and (c) DLK1. (n = 3, biological replicates). (d) Representative immunofluorescence co-staining of PAX7+ human satellite cells for DLK1/ICAM, VCAM1/ICAM1 and VCAM1/DLK1 (scale, 50 µm). Lavender arrows mark double positive PAX7+ cells, white arrowheads denote single positive PAX7+ cells. (n = 3, biological replicates). (e) Bar graph showing the proportion of double and single positive satellite cells for DLK1, VCAM1 and ICAM1 in human muscle as quantified by immunofluorescence staining (n = 3, biological replicates). *p<0.05, **p<0.01, ***p<0.001, ****p<0.0001. Data presented as mean ± SEM.
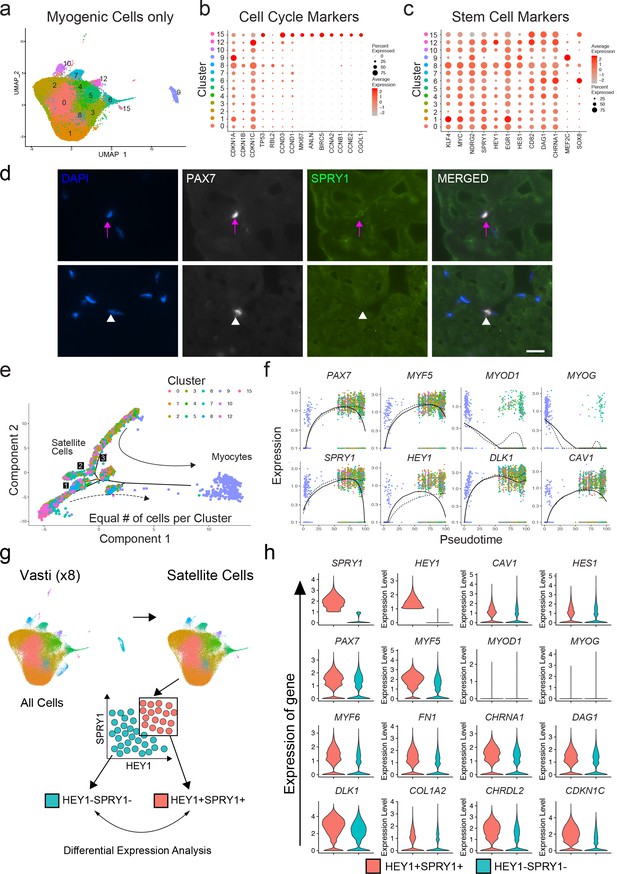
Quiescence molecular signature of human sorted satellite cells.
(a) UMAP representing all myogenic cells used for downstream analyses. (b) Dot plot displaying the expression of cell cycle genes associated with satellite cell stemness, quiescence, and activation in the satellite cells for all myogenic clusters. (c) Dot plot displaying the expression of genes associated with satellite cell stemness, quiescence, and activation in the satellite cells. (d) Immunofluorescence staining of human satellite cells for SPRY1 within sections of human muscle. Pink arrows denote human satellite cells that are positive for the marker (scale, 20 µm). White arrowheads mark satellite cells that are negative for expression of SPRY1. (n = 3, biological replicates). (e) Pseudotime trajectories developed through Monocle analysis for the eight vasti. The object was downsampled and 500 cells per cluster were used. Plot cells were labeled by cluster as in (a). Arrows represent the direction of pseudotime. Branch points 1–3 are marked with numbers along the trajectories. (f) Gene plots displaying the expression of specific genes through branch point ‘3’, as a function of pseudotime. (g) Schematic diagramming the in silico process for sorting satellite cells by expression of SPRY1 and HEY1 within the cells from the eight vasti. Satellite cells were first subclustered and separated by gene expression, followed by analysis to discover differentially expressed genes. (h) Violin plots displaying expressed genes in the SPRY1+HEY1+ and SPRY1-HEY1- experimental groups. Plots on the x-axis are colored by group. Specific gene expression is on the y-axis. The width of the violin plots depicts the larger probability density of cells expressing each gene particular gene at the indicated expression level.
-
Figure 3—source data 1
Quiescence molecular signature of human sorted satellite cells.
- https://cdn.elifesciences.org/articles/51576/elife-51576-fig3-data1-v2.pdf

Quiescence state of human satellite cells and pseudotime trajectory.
(a) Seurat Cell cycle scoring for the vasti lateralis. Bar plot of the proportion of cells assigned to the G1/G0, G2/M or S phase according to each cluster (myogenic cluster 0–10, 12, 15). (b) Violin plot displaying the RNA count per cluster myogenic clusters (0–10, 12, 15) for the eight pooled vasti lateralis. (c) Dot plot displaying the expression of genes associated with satellite cell stemness, quiescence, and activation in the satellite cells clusters 0–4 in the rectus femoris quadriceps muscle of an 84 year old male. (d) Pseudotime trajectories developed through Monocle analysis of all 5,062 cells from the rectus femoris quadriceps muscle labeled by cluster as in Figure 1—figure supplement 2b. Arrows represent the direction of pseudotime. Branch points 1–3 are marked with numbers along the trajectories. (e) Gene plots displaying the expression of specific genes through branch point ‘3’, as a function of pseudotime.
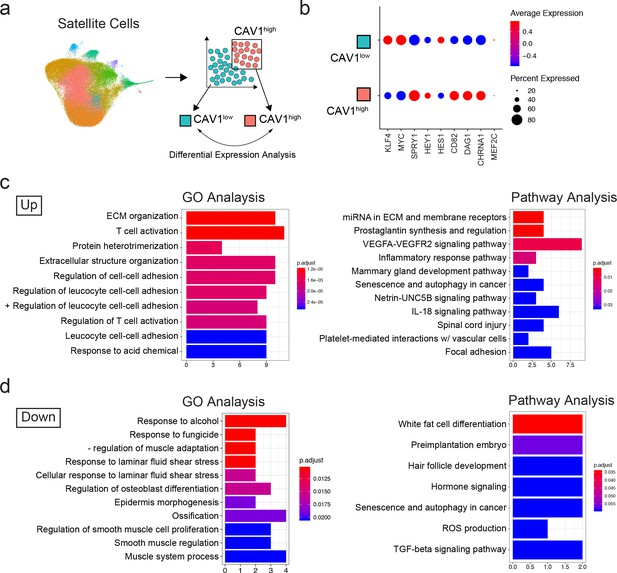
Transcriptomic analysis of CAV1 high expressing cells .
(a) Schematic diagramming the in silico process for sorting satellite cells by expression of CAV1. Satellite cells were first subclustered and separated by gene expression, followed by analysis to discover differentially expressed genes. (b) Dotplot displaying the average expression and percentage of cells expressing quiescence markers. (c–d) Gene ontology and Pathway analyses of differentially up- and down-regulated genes in CAV1 high expressing satellite cells.
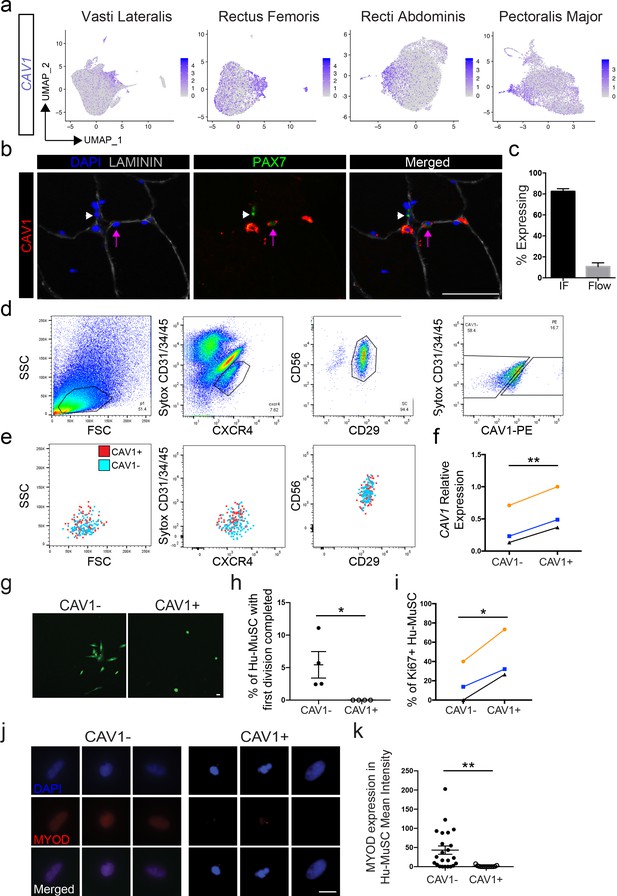
CAV1 high expressing human satellite cell phenotypes.
(a) Feature plots displaying localized CAV1 gene expression within the 2D UMAP space as shown in Figure 1b. Each dot represents a single cell. Deeper purple coloration represents increased expression. (b) Immunofluorescence staining of human satellite cells for CAV1 within sections of human muscle. Lavender arrows denote human satellite cells that are positive for the marker (scale, 50 µm). White arrowheads mark satellite cells that are negative for expression of the marker. (n = 3, biological replicates). (c) Bar plot displaying the quantification CAV1 expression of PAX7+ human satellite cells with both immunofluorescence staining and flow cytometry. (n ≥ 3, biological replicates). Data presented as mean ± SEM. (d) Representative flow cytometry profiles from the isolation of CXCR4/CD29/CD56 human satellite cells based on expression of CAV1. (e) Representative back-gating of CAV1+ (red) and CAV1- (blue) cells, demonstrating overlap of profiles within prior gates. (n = 3, biological replicates). (f). CAV1 gene expression in sorted CAV1- and CAV1+l satellite cells (n = 3, biological replicates) *p<0.05. (g) Morphology of sorted CAV1- and CAV1+ satellite cells stained with CellTracker Green 4.6 days after isolation (scale, 20µm). (h) Timelapse analysis to assess time to first division. Quantification of the percentage of cells dividing in the first 6 days after isolation (n = 4, biological replicates, *p<0.05, mean ± SEM). (i) Percentage of cells expressing Ki67 3 days after isolation (n = 3, biological replicates, *p<0.05). (j) Immunnofluorescence staining of MYOD 3 days after isolation (scale, 10 µm). (k) Quantification of MYOD expression at day 3 in vitro (n = 4, biological replicates, **p<0.01, mean ± SEM). Comparisons of CAV1+ and CAV1- satellite cells are from the same donor in each individual experiment.
-
Figure 5—source data 1
CAV1 high expressing human satellite cell phenotypes.
- https://cdn.elifesciences.org/articles/51576/elife-51576-fig5-data1-v2.pdf

PAX7 analysis of CAV1- and CAV1+l sorted satellite cells.
(a) PAX7 immunoflurescence staining of CAV1- and CAV1+l sorted satellite cells 3 hr after isolation (scale, 20 µm). (b) Gene expression analysis of PAX7 in CAV1- and CAV1+ sorted satellite cells (n = 3, biological replicates). Data presented as mean ± SEM. HEK293 cells were used as negative control for the expression of PAX7.
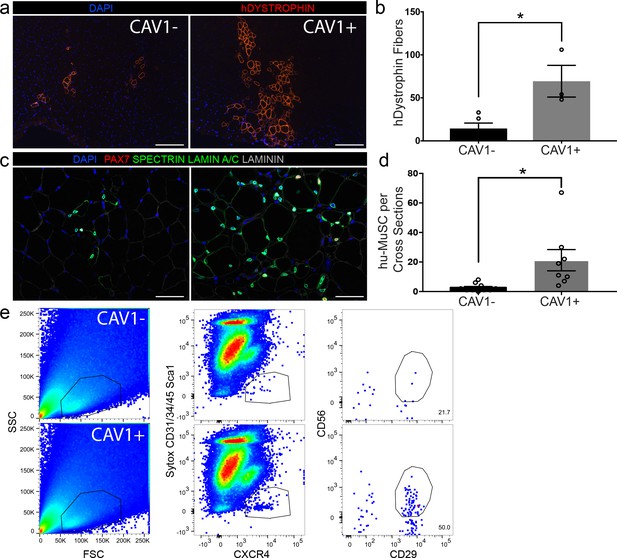
CAV1 high expressing human satellite cells engraft robustly after transplantation in mice.
(a) Representative immunofluorescence staining images for human specific DYSTROPHIN (scale, 200 µm) within NSG mouse muscle cross-sections in TAs transplanted with CAV1+ and CAV1- human satellite cells. 500 cells were transplanted in each NSG TA. (n = 3, using three separate donors.) (b) Bar graph depicting the number of DYSTROPHIN positive human fibers in mice transplanted with CAV1+ and CAV1- cells. (n = 3, biological replicates) *p<0.05. Data presented as mean ± SEM. (c) Representative immunofluorescence staining images for human PAX7, SPECTRIN, LAMIN A/C and LAMININ within NSG mouse muscle cross-sections in TAs transplanted with CAV1+ and CAV1- human satellite cells (scale, 100 µm). (n = 3, biological replicates). (d) Bar graph represents number of human PAX7+ cells in mice transplanted with both CAV1+ and CAV1- cells. (n = 3, biological replicates) *p<0.05. Data presented as mean ± SEM. (e) Human satellite cells were re-isolated by FACS from mice transplanted with CAV1+ and CAV1- satellite cells.
-
Figure 6—source data 1
CAV1 high expressing human satellite cells engraft robustly after transplantation in mice.
- https://cdn.elifesciences.org/articles/51576/elife-51576-fig6-data1-v2.pdf
Tables
Reagent type (species) or resource | Designation | Source or reference | Identifiers | Additional information |
---|---|---|---|---|
NSG mice | NOD.Cg-Prkdcscid Il2rgtm1Wjl/SzJ | https://www.jax.org/strain/005557 | 005557 | 8–12 week-old |
Sequenced-based reagent | Human RT-PCR Primers | Applied Biosystems Taqman Assays | B-actin Hs01060665_g1 | |
Sequenced-based reagent | Human RT-PCR Primers | Applied Biosystems Taqman Assays | CAV1 Hs00971716_m1 | |
Sequenced-based reagent | Human RT-PCR Primers | Applied Biosystems Taqman Assays | PAX7 Hs00242962_m1 | |
Antibody | Mouse monoclonal anti-Human DYSTROPHIN | DSHB | MANDYS104(7F7) | IF(1:10) |
Antibody | Mouse monoclonal anti-Human/Mouse PAX7 | DSHB | PAX7 | IF(1:10) |
Antibody | Rabbit polyclonal anti-LAMININ | Sigma-Aldrich | L9393 | IF(1:250) |
Antibody | Mouse monoclonal anti-Human SPECTRIN | Leica Microsystems | NCL-SPEC1 | IF(1:100) |
Antibody | Mouse monoclonal anti-Human LAMIN A/C | Vector Laboratories | VP-L550 | IF(1:100) |
Antibody | Mouse monoclonal anti-Human/Mouse MYOD | BD Pharmigen | 554130 | IF(1:100) |
Antibody | Rabbit polyclonal anti-CAV1 | abcam | ab2910 | IF(1:500) |
Antibody | Mouse monoclonal anti-CAV1 (7C8) | Santa Cruz Biotechnology | sc-53564 | IF(1:50) |
Antibody | Mouse monoclonal anti-DLK1 (MM0514-9D8) | abcam | ab89908 | IF(1:50) |
Antibody | Mouse monoclonal anti-ICAM1 (G-5) | Santa Cruz Biotechnology | sc-8439 | IF(1:50) |
Antibody | Rabbit monoclonal anti-VCAM1 (EPR5047) | abcam | ab134047 | IF(1:75) |
Antibody | Mouse monoclonal anti-Human CYCS | LifeSpan Biosciences | LS-B6577 | IF(1:100) |
Antibody | Mouse monoclonal anti-Human Ki67 | BD Pharmigen | 556003 | IF(1:100) |
Antibody | Rabbit polyclonal anti-Human SPROUTY1 | abcam | ab111523 | IF(1:50) |
Antibody | Mouse monoclonal anti-Human CD31 (Beads) | Miltenyi Biotec | 130-091-935 | FACS |
Antibody | Mouse monoclonal anti-Human CD45 (Beads) | Miltenyi Biotec | 130-045-801 | FACS |
Antibody | Mouse monoclonal anti-Human CD31 AF450 (WM-59) | Ebioscience | 48-0319-42 | FACS |
Antibody | Mouse monoclonal anti-Human CD34 eFluor450 (4H11) | Ebioscience | 48-0349-42 | FACS |
Antibody | Mouse monoclonal anti-Human CD45 AF450 (30-F11) | Ebioscience | 48-0451-82 | FACS |
Antibody | Mouse monoclonal anti-Human CD29 FITC (TS2/16) | Ebioscience | 11-0299-41 | FACS |
Antibody | Recombinant human anti-CD56 APC-vio-770 (REA196) | Miltenyi Biotec | 130-114-548 | FACS |
Antibody | Mouse monoclonal anti-Human CXCR4 PE (12G5) | Ebioscience | 12-9999-41 | FACS |
Antibody | Mouse monoclonal anti-Human CXCR4 APC (12G5) | Ebioscience | 17-9999-42 | FACS |
Antibody | Rabbit monoclonal anti-Human CAV1 PE (EPR15554) | abcam | ab212007 | FACS |
Antibody | Mouse monoclonal anti-Human ICAM1 PE (15.2) | abcam | ab210195 | FACS |
Antibody | Mouse monoclonal anti-Human VCAM1 PE (STA) | Ebioscience | 12-1069-42 | FACS |
Antibody | Mouse monoclonal anti-Human DLK1 PE (211309) | R and Dsystems | MAB1144 | FACS |
Antibody | FcR block | Miltenyi Biotec | 130-059-901 | |
Software, algorithm | GraphPad Prism | GraphPad Prism (https://graphpad.com) | ||
Software, algorithm | ImageJ | ImageJ (http://imagej.nih.gov/ij/) | ||
Software, algorithm | Seurat (3.1.2) | https://satijalab.org/seurat/ | ||
Software, algorithm | Monocle (2.12.0) | http://cole-trapnell-lab.github.io/monocle-release/ | ||
Software, algorithm | cellranger | https://support.10xgenomics.com/single-cell-gene-expression/software/pipelines/latest/feature-bc | ||
Software, algorithm | FlowJo | https://www.flowjo.com | ||
Cell Line (Homo sapiens) | HEK293 | ATCC Cat# PTA-4488, | RRID:CVCL_0045 |
Additional files
-
Source code 1
Multiple dataset analysis.
- https://cdn.elifesciences.org/articles/51576/elife-51576-code1-v2.r
-
Source code 2
Pseudotime analysis.
- https://cdn.elifesciences.org/articles/51576/elife-51576-code2-v2.r
-
Source code 3
HEY1highSPRY1high subsetting.
- https://cdn.elifesciences.org/articles/51576/elife-51576-code3-v2.r
-
Source code 4
CAV1high subsetting.
- https://cdn.elifesciences.org/articles/51576/elife-51576-code4-v2.r
-
Source code 5
Gene Ontology and Pathaway analyses.
- https://cdn.elifesciences.org/articles/51576/elife-51576-code5-v2.rmd
-
Supplementary file 1
Genes differentially expressed in each cluster for the combined vasti lateralis samples.
- https://cdn.elifesciences.org/articles/51576/elife-51576-supp1-v2.xlsx
-
Supplementary file 2
Genes differentially expressed in each cluster for the rectus femoris sample.
- https://cdn.elifesciences.org/articles/51576/elife-51576-supp2-v2.xlsx
-
Supplementary file 3
Genes differentially expressed in the SPRY1/HEY1 high expressing satellite cells.
- https://cdn.elifesciences.org/articles/51576/elife-51576-supp3-v2.xlsx
-
Supplementary file 4
Genes differentially expressed in the CAV1 high expressing satellite cells.
- https://cdn.elifesciences.org/articles/51576/elife-51576-supp4-v2.xlsx
-
Supplementary file 5
Type of muscle used per experiment.
- https://cdn.elifesciences.org/articles/51576/elife-51576-supp5-v2.xlsx
-
Transparent reporting form
- https://cdn.elifesciences.org/articles/51576/elife-51576-transrepform-v2.pdf