Enzyme Mechanisms: Wedging open a catalytic site
Of the many ways to regulate blood pressure, the simplest is to dilate or narrow the blood vessels. In the body, the gas nitric oxide (NO) binds to the enzyme soluble guanylyl cyclase (sGC) to relax blood vessels and decrease blood pressure. When the interaction between NO and sGC is disrupted, people develop hypertension and pulmonary arterial hypertension, and have a higher risk of heart failure. This makes sGC a major target for the treatment of cardiovascular diseases.
Drugs that activate the sGC enzyme have been used for many years, often without understanding their mechanism of action. One striking example is nitroglycerin, which has been used to treat angina pectoris since the end of the 19th century. It took over 100 years to discover that nitroglycerin and other nitrates work by generating NO or its derivatives, which then stimulate sGC to produce cyclic guanosine monophosphate (cGMP). The cGMP produced in this way relaxes the coronary arteries and increases blood flow to the heart. Unfortunately, nitroglycerin and most NO donors induce resistance, meaning that the patient becomes less and less responsive to ever-increasing doses of the drug.
In 1994, a small molecule called YC-1, which is not an NO derivative, was found to dramatically enhance the ability of the sGC enzyme to produce cGMP at low NO concentrations (Ko et al., 1994; Friebe et al., 1998). In healthy individuals, NO is produced by endothelial cells, which line the inside of blood vessels. When these cells stop performing their normal roles, the resulting low levels of NO lead to vascular diseases.
Nowadays, pulmonary arterial hypertension is treated with molecules similar to YC-1 (such as Adempas), which can stimulate the sGC enzyme without inducing resistance. These drugs are also in clinical trials for the treatment of chronic heart failure and have the potential to be used for treating chronic kidney diseases, hypertension and fibrotic diseases (Buys et al., 2018; Sandner et al., 2018). Two questions have been the subject of intense scrutiny and conflicting data: where does YC-1 bind to sGC, and how does it increase the impact of NO. Now, in eLife, Michael Marletta, Jim Hurley and colleagues at Berkeley, including Ben Horst and Adam Yokom as joint first authors, report the results of cryo-electron microscopy (cryo-EM) and small angle X-ray scattering experiments that shed light on these questions (Horst et al., 2019).
Structurally and kinetically, sGC is a complex enzyme. It is a heterodimer formed by two subunits, α and β, which each contain four domains: an N-terminal heme nitric oxide/oxygen binding (HNOX) domain; a Per/Arnt/Sim (PAS)-fold domain; a coiled-coil (CC) domain; and a catalytic domain at the C-terminal (Figure 1A). NO binds to the heme in the β subunit. Over the years, X-ray structures of each domain have been obtained, but attempts to determine the full-length 3D structure of the sGC enzyme have failed repeatedly. Without such structures it is not clear how these domains rearrange upon NO binding to create the most favorable conformation for the production of cGMP (Montfort et al., 2017; Horst and Marletta, 2018). Moreover, it was impossible to determine how molecules similar to YC-1 achieve their therapeutic effect.
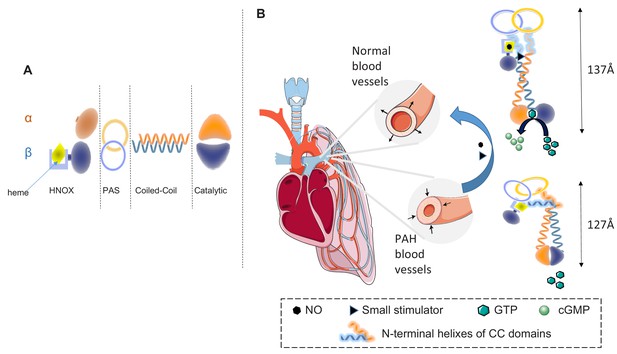
Soluble guanylyl cyclase (sGC) and the treatment of pulmonary arterial hypertension (PAH).
(A) The enzyme sGC contains two subunits, α and β, and each subunit is composed of four domains: the N-terminal heme nitric oxide/oxygen binding (HNOX) domain, the Per-Arnt-Sim (PAS) domain, the coiled-coil (CC) domain, and the catalytic domain. (B) In pulmonary arterial hypertension blood vessels contract excessively, thus increasing arterial pressure (left); this process can be reversed (blue arrow) by nitric oxide (NO, hexagons) and drugs called small sGC stimulators (triangles). These molecules bind to sGC and change its conformation so it becomes active and can catalyze the conversion of guanosine triphosphate (GTP, green hexagons) to cyclic guanosine monophosphate (cGMP, light green circles). This last molecule acts a secondary messenger and induces the dilation of blood vessels. Horst et al. have shed light on the mechanism of activation for the sGC enzyme. In the inactive state (bottom), the catalytic site is inaccessible, so the enzyme cannot catalyze the reaction that converts GTP to cGMP; there is also a sharp bend in each coiled-coil domain. When NO binds to the heme in the HNOX domain of the β subunit, the HNOX and PAS domains rotate by 71° leading to the straightening of the bend in the two coiled-coil domains, which opens the catalytic site, thus allowing the enzyme to catalyze the conversion of GTP to cGMP (top). Small stimulators such as YC-1 bind into the space created between the β HNOX and CC domains, acting as a wedge that keeps the enzyme active for longer.
Horst et al. employed cryo-EM to obtain the full-length 3D structures of sGC in a non-activated state and in an activated state after the addition of NO and YC-1. Comparing these two structures reveals the structural changes caused by NO binding and suggest a likely mechanism for the therapeutic action of YC-1-like drugs. When NO binds to the sGC enzyme, a major rearrangement of the HNOX and PAS domains in the β subunit takes place, inducing the CC domains, which include a bend, to become straighter. This twist, in turn, creates an interface between the HNOX and CC domains in the β subunit. These conformational changes lead to opening of the catalytic pocket, making it possible for the sGC enzyme to bind guanosine triphosphate (GTP) and catalyze the production of cGMP (Figure 1B, right panel). Interestingly, YC-1 was found in a site created by the NO-induced rearrangement of the β HNOX and CC domains, where it could act as a wedge to maintain an unbent active conformation.
The structures reported by Horst et al. are similar to recent cryo-EM structures of sGC with and without excess NO (Kang et al., 2019). This suggests that activation at low NO concentrations enhanced by YC-1 is structurally comparable to that at high NO concentrations in the absence of YC-1. These models of sGC activation end years of frustration in the field, and provide a structural basis to further improve the design of small sGC stimulators that could be used to treat cardiovascular diseases (Figure 1).
It remains to be resolved whether YC-1 binds to the same site in the absence of NO, since there are a number of other possible sites (Stasch et al., 2001; Lamothe et al., 2004; Wales et al., 2018). In the current study, small-angle X-ray scattering models indicate that, in the absence of YC-1, sGC enzymes with an NO-equivalent molecule bound to the heme are a mix of bent-inactive and partially extended-activated structures. However, it is still unclear how higher concentrations of NO induce the conversion to the fully extended-activated structure. The mechanism of deactivation also remains unknown. Resolving these two matters is a priority for researchers working on the physiological role of NO.
References
-
YC-1 potentiates nitric oxide- and carbon monoxide-induced cyclic GMP effects in human plateletsMolecular Pharmacology 54:962–967.https://doi.org/10.1124/mol.54.6.962
-
Structure and activation of soluble guanylyl cyclase, the nitric oxide sensorAntioxidants & Redox Signaling 26:107–121.https://doi.org/10.1089/ars.2016.6693
-
Discovery of stimulator binding to a conserved pocket in the heme domain of soluble guanylyl cyclaseJournal of Biological Chemistry 293:1850–1864.https://doi.org/10.1074/jbc.RA117.000457
Article and author information
Author details
Publication history
- Version of Record published: November 8, 2019 (version 1)
Copyright
© 2019, Beuve
This article is distributed under the terms of the Creative Commons Attribution License, which permits unrestricted use and redistribution provided that the original author and source are credited.
Metrics
-
- 740
- views
-
- 65
- downloads
-
- 3
- citations
Views, downloads and citations are aggregated across all versions of this paper published by eLife.
Download links
Downloads (link to download the article as PDF)
Open citations (links to open the citations from this article in various online reference manager services)
Cite this article (links to download the citations from this article in formats compatible with various reference manager tools)
Further reading
-
- Biochemistry and Chemical Biology
- Plant Biology
Transmembrane signaling by plant receptor kinases (RKs) has long been thought to involve reciprocal trans-phosphorylation of their intracellular kinase domains. The fact that many of these are pseudokinase domains, however, suggests that additional mechanisms must govern RK signaling activation. Non-catalytic signaling mechanisms of protein kinase domains have been described in metazoans, but information is scarce for plants. Recently, a non-catalytic function was reported for the leucine-rich repeat (LRR)-RK subfamily XIIa member EFR (elongation factor Tu receptor) and phosphorylation-dependent conformational changes were proposed to regulate signaling of RKs with non-RD kinase domains. Here, using EFR as a model, we describe a non-catalytic activation mechanism for LRR-RKs with non-RD kinase domains. EFR is an active kinase, but a kinase-dead variant retains the ability to enhance catalytic activity of its co-receptor kinase BAK1/SERK3 (brassinosteroid insensitive 1-associated kinase 1/somatic embryogenesis receptor kinase 3). Applying hydrogen-deuterium exchange mass spectrometry (HDX-MS) analysis and designing homology-based intragenic suppressor mutations, we provide evidence that the EFR kinase domain must adopt its active conformation in order to activate BAK1 allosterically, likely by supporting αC-helix positioning in BAK1. Our results suggest a conformational toggle model for signaling, in which BAK1 first phosphorylates EFR in the activation loop to stabilize its active conformation, allowing EFR in turn to allosterically activate BAK1.
-
- Biochemistry and Chemical Biology
- Neuroscience
Amyloid β (Aβ) peptides accumulating in the brain are proposed to trigger Alzheimer’s disease (AD). However, molecular cascades underlying their toxicity are poorly defined. Here, we explored a novel hypothesis for Aβ42 toxicity that arises from its proven affinity for γ-secretases. We hypothesized that the reported increases in Aβ42, particularly in the endolysosomal compartment, promote the establishment of a product feedback inhibitory mechanism on γ-secretases, and thereby impair downstream signaling events. We conducted kinetic analyses of γ-secretase activity in cell-free systems in the presence of Aβ, as well as cell-based and ex vivo assays in neuronal cell lines, neurons, and brain synaptosomes to assess the impact of Aβ on γ-secretases. We show that human Aβ42 peptides, but neither murine Aβ42 nor human Aβ17–42 (p3), inhibit γ-secretases and trigger accumulation of unprocessed substrates in neurons, including C-terminal fragments (CTFs) of APP, p75, and pan-cadherin. Moreover, Aβ42 treatment dysregulated cellular homeostasis, as shown by the induction of p75-dependent neuronal death in two distinct cellular systems. Our findings raise the possibility that pathological elevations in Aβ42 contribute to cellular toxicity via the γ-secretase inhibition, and provide a novel conceptual framework to address Aβ toxicity in the context of γ-secretase-dependent homeostatic signaling.