Acetylcholine acts on songbird premotor circuitry to invigorate vocal output
Figures
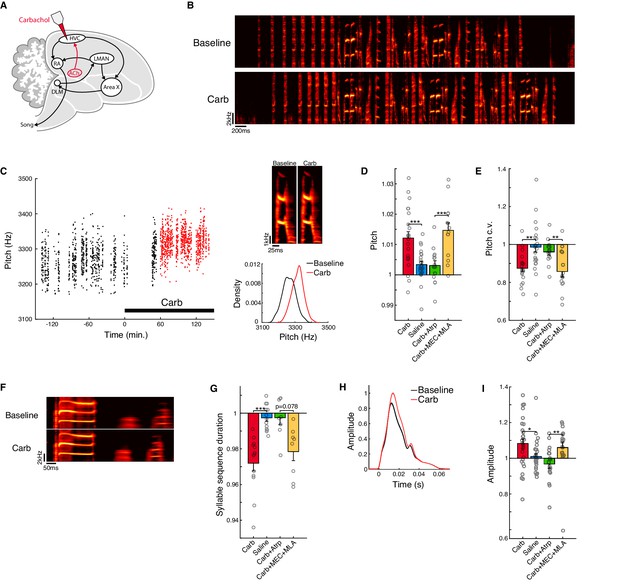
Activation of muscarinic receptors in HVC increases motor vigor.
(A) Experiment schematic and song system. Carbachol (Carb) was microdialyzed into HVC. HVC receives a cholinergic projection from a homolog of the nucleus basalis (ACh, red). The avian song control system consists of a direct motor pathway that includes the cortical nuclei HVC (used as a proper name) and RA (the robust nucleus of the arcopallium), which projects to the brainstem premotor regions that control vocal musculature; and an Anterior Forebrain Pathway (AFP) that includes the basal ganglia homologue Area X, the thalamic nucleus DLM (the dorsolateral nucleus of the medial thalamus), and the cortical nucleus LMAN (the lateral magnocellular nucleus of the anterior nidopallium), which projects back to the motor pathway at the level of RA. (B) Example song bouts before and after carbachol. (C) Change in pitch produced by carbachol for one example syllable (one experiment). Left, time course of raw pitch values. Red points indicate data used for analysis of carbachol effects (60–180 min. following onset of dialysis). Top right, rendition-averaged spectrograms. Lower right, pitch distributions during baseline (black) and Carb (red) conditions. (D) Normalized (drug/baseline) pitch (mean ± s.e.m. increase in pitch for Carb: 1.2 ± 0.21%, n = 20 experiments; Saline: 0.34 ± 0.11%, n = 17 experiments; Carb+Atrp: 0.31 ± 0.16%, n = 10 experiments; Carb+MEC+MLA: 1.5 ± 0.24%, n = 10 experiments; Carb vs. Saline, p=0.00088, two-tailed signed-rank test, n = 22 syllables, eight birds; Carb+Atrp vs. Carb+MEC+MLA, p=0.00024, two-tailed signed-rank test, n = 14 syllables, five birds). (E) Normalized (drug/baseline) pitch c.v. (mean ± s.e.m. reduction in pitch c.v. for Carb: 13 ± 1.6%, n = 20 experiments; Saline: 1.4 ± 2.7%, n = 17 experiments; Carb+Atrp: 4.0 ± 1.7%, n = 10 experiments; Carb+MEC+MLA: 14 ± 2.9%, n = 10 experiments; Carb vs. Saline, p=0.0014, two-tailed signed-rank test, n = 22 syllables, eight birds; Carb+Atrp vs. Carb+MEC+MLA, p=0.0023, two-tailed signed-rank test, n = 14 syllables, five birds). (F) Rendition-averaged spectrograms of one example syllable sequence before and after carbachol (one experiment). (G) Normalized (drug/baseline) syllable sequence duration (mean ± s.e.m. reduction in sequence duration for Carb: 2.8 ± 0.43%, n = 20 experiments; Saline: 0.26 ± 0.20%, n = 15 experiments; Carb+Atrp: 0.25 ± 0.39%, n = 9 experiments; Carb+MEC+MLA: 2.2 ± 0.51%, n = 10 experiments; Carb vs. Saline, p=0.00024, two-tailed signed-rank test, n = 13 syllable sequences, eight birds; Carb+Atrp vs. Carb+MEC+MLA, p=0.078, two-tailed signed-rank test, n = 8 syllable sequences, five birds). (H) Amplitude envelopes (mean ± s.e.m.) for one example syllable before and after carbachol (one experiment). Amplitude envelopes were normalized to the maximum value in the carbachol condition. (I) Normalized (drug/baseline) amplitude (mean ± s.e.m. increase in amplitude for Carb: 8.3 ± 2.5%, n = 18 experiments; Saline: 0.99 ± 1.6%, n = 17 experiments; Carb+Atrp: −3.2 ± 2.4%, n = 10 experiments; Carb+MEC+MLA: 6.1 ± 3.1%, n = 9 experiments; Carb vs. Saline, p=0.012, two-tailed signed-rank test, n = 30 syllables, eight birds; Carb+Atrp vs. Carb+MEC+MLA, p=0.0033, two-tailed signed-rank test, n = 18 syllables, five birds). ***p<0.001, **p<0.01, *p<0.05. For panels D, E, G, and I, each point represents one syllable or syllable sequence averaged over experiments; Atrp = the muscarinic antagonist atropine; MEC and MLA = the nicotinic antagonists mecamylamine and methyllycaconitine.
-
Figure 1—source data 1
Linear mixed effects model analysis of the behavioral effects of carbachol.
- https://cdn.elifesciences.org/articles/53288/elife-53288-fig1-data1-v1.docx
-
Figure 1—source data 2
Source data for the summary analyses in figure panels D, E, G, and I.
- https://cdn.elifesciences.org/articles/53288/elife-53288-fig1-data2-v1.mat
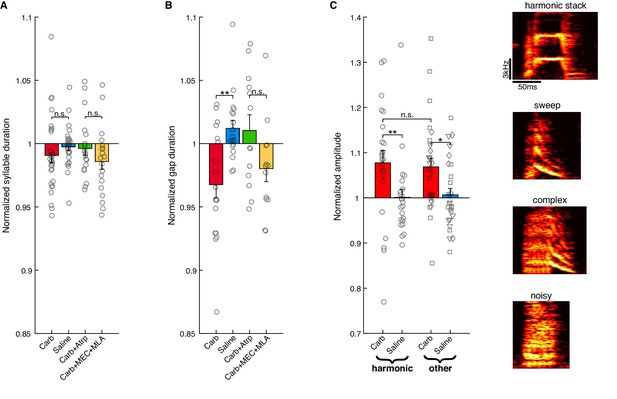
Further characterization of the tempo and amplitude effects of carbachol.
(A) Normalized (drug/baseline) syllable duration (mean ± s.e.m. reduction in syllable duration for Carb: 0.93 ± 0.58%, n = 20 experiments; Saline: 0.26 ± 0.31%, n = 16 experiments; Carb+Atrp: 0.39 ± 0.55%, n = 10 experiments; Carb+MEC+MLA: 1.4 ± 0.62%, n = 10 experiments; Carb vs. Saline, p=0.12, two-tailed signed-rank test, n = 28 syllables, eight birds; Carb+Atrp vs. Carb+MEC+MLA, p=0.11, two-tailed signed-rank test, n = 19 syllables, five birds). (B) Normalized (drug/baseline) gap duration (mean ± s.e.m. reduction in gap duration for Carb: 3.2 ± 1.0%, n = 17 experiments; Saline: −1.2 ± 0.63%, n = 14 experiments; Carb+Atrp: −1.0 ± 1.2%, n = 8 experiments; Carb+MEC+MLA: 2.0 ± 1.0%, n = 8 experiments; Carb vs. Saline, p=0.0025, two-tailed signed-rank test, n = 18 gaps, seven birds; Carb+Atrp vs. Carb+MEC+MLA, p=0.17, two-tailed signed-rank test, n = 13 gaps, four birds). (C) Left: normalized (drug/baseline) amplitude grouped by syllable type. ‘Other’ syllables includes sweeps (triangles), complex syllables (diamonds), and noisy syllables (squares). Right: example spectrograms for each of the syllable types. All spectrograms have the same time and frequency scales. See Methods for additional details (mean ± s.e.m. increase in amplitude for harmonic syllables, Carb: 7.7 ± 2.7%, n = 20 experiments, 23 syllables, eight birds; harmonic syllables, Saline: 0.060 ± 1.9%, n = 19 experiments, 23 syllables, eight birds; other syllables, Carb: 6.9 ± 1.9%, n = 20 experiments, 28 syllables, eight birds; other syllables, Saline: 0.67 ± 1.4%, n = 19 experiments, 28 syllables, eight birds; Carb vs. Saline, harmonic syllables: p=0.0074, two-tailed signed-rank test; Carb vs. Saline, other syllables: p=0.023, two-tailed signed-rank test; Carb harmonic vs. Carb other: p=0.43, two-tailed rank-sum test). **p<0.01,*p<0.05, n.s., not significant.
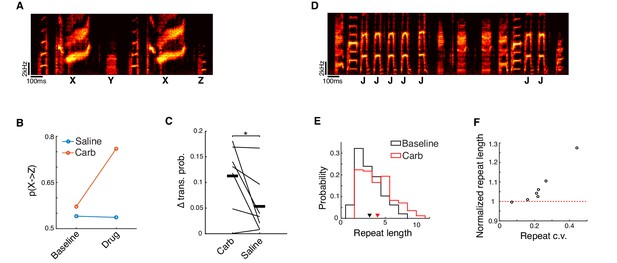
Microdialysis of carbachol into HVC alters song sequencing.
(A) Spectrogram of a song with a divergent branch point. Syllable ‘X’ can transition to syllable ‘Y’ or syllable ‘Z’. (B) Transition probabilities before and after either carbachol or saline dialysis for the branch point shown in panel A. (C) Change in transition probability averaged across all branch points for each bird (see Methods; mean ± s.e.m. change for Carb: 0.11 ± 0.025, Saline: 0.053 ± 0.022; Carb vs. Saline, p=0.047, two-sided signed-rank test, n = 15 branch points, seven birds, 11 experiments for Carb, 12 experiments for Saline). On a case-by-case basis, transition probabilities at 6 out of 15 branch points were significantly affected by carbachol, while only 1 out of 15 was significantly affected by saline (p<0.05, generalized likelihood ratio test for homogeneity, see Methods). (D) Song spectrogram depicting a variably repeated syllable (syllable 'J'). (E) Histogram of repeat counts before and after carbachol for the repeated syllable shown in panel D. (F) Scatter plot of repeat length c.v. vs. normalized repeat count (carbachol/baseline) after carbachol (p=0.0012, test for non-zero Pearson's correlation coefficient, corr. coeff. = 0.95; mean ± s.e.m. increase in repeat length: 7.4 ± 3.6%; mean ± s.e.m. repeat length c.v.: 0.23 ± 0.043, n = 7 repeated syllables, six birds, 12 experiments). *p<0.05.
-
Figure 2—source data 1
Source data for the summary analyses in figure panels C and F.
- https://cdn.elifesciences.org/articles/53288/elife-53288-fig2-data1-v1.mat
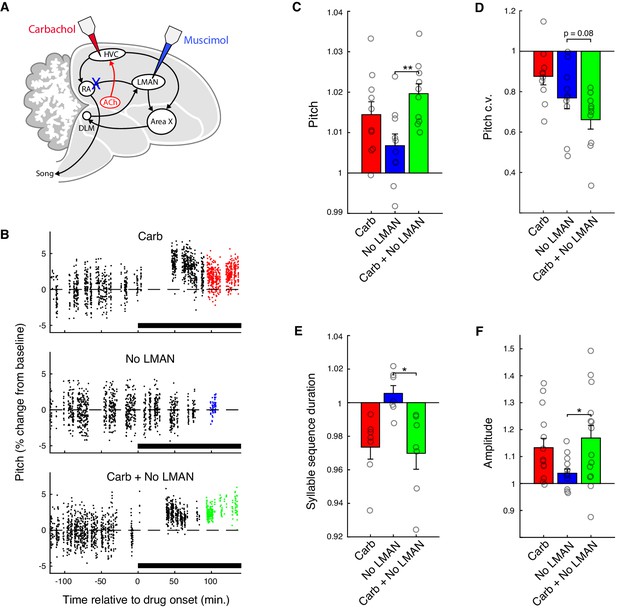
Carbachol invigorates song via the cortical-brainstem motor pathway.
(A) Experiment schematic. Carbachol (Carb) was microdialyzed into HVC, and LMAN was inactivated with muscimol (No LMAN), concurrently or separately. (B) Time course of pitch values for representative experiments from one bird (the same syllable is shown for each condition). For each condition, pitch is plotted as percent change relative to the average value during baseline (−120 to 0 min.). Points in color indicate data used for analysis of drug effects (90 to 150 min.). (C) Normalized (drug/baseline) pitch (mean ± s.e.m. increase in pitch for Carb: 1.4 ± 0.32%, No LMAN: 0.68 ± 0.29%, Carb+No LMAN: 2.0 ± 0.24%; Carb+No LMAN vs. No LMAN, p=0.0020, two-tailed signed-rank test, n = 10 syllables, four birds). (D) Normalized (drug/baseline) pitch c.v. (mean ± s.e.m. reduction in pitch c.v. for Carb: 12 ± 4.2%, No LMAN: 23 ± 5.3%, Carb+No LMAN: 34 ± 4.7%; Carb+No LMAN vs. No LMAN, p=0.084, two-tailed signed-rank test, n = 10 syllables, four birds). (E) Normalized (drug/baseline) syllable sequence duration (mean ± s.e.m. reduction in sequence duration for Carb: 2.7 ± 0.72%, No LMAN: −0.55 ± 0.47%, Carb+No LMAN: 3.0 ± 0.95%; Carb+No LMAN vs. No LMAN, p=0.031, two-tailed signed-rank test, n = 7 syllable sequences, four birds). (F) Normalized (drug/baseline) amplitude (mean ± s.e.m. increase in amplitude for Carb: 13 ± 3.5%, No LMAN: 3.8 ± 1.5%, Carb+No LMAN: 17 ± 4.7%; Carb+No LMAN vs. No LMAN, p=0.035, two-tailed signed-rank test, n = 14 syllables, four birds). **p<0.01, *p<0.05. For panels C–F, each point represents one syllable or syllable sequence averaged over experiments. For panels C, D, and E, n = 9 experiments for all conditions. For panel F, n = 8 experiments for Carb, nine for No LMAN, and nine for Carb + No LMAN.
-
Figure 3—source data 1
Linear mixed effects model analysis of combined carbachol and LMAN inactivation experiments.
- https://cdn.elifesciences.org/articles/53288/elife-53288-fig3-data1-v1.docx
-
Figure 3—source data 2
Source data for the summary analyses in figure panels C−F.
- https://cdn.elifesciences.org/articles/53288/elife-53288-fig3-data2-v1.mat
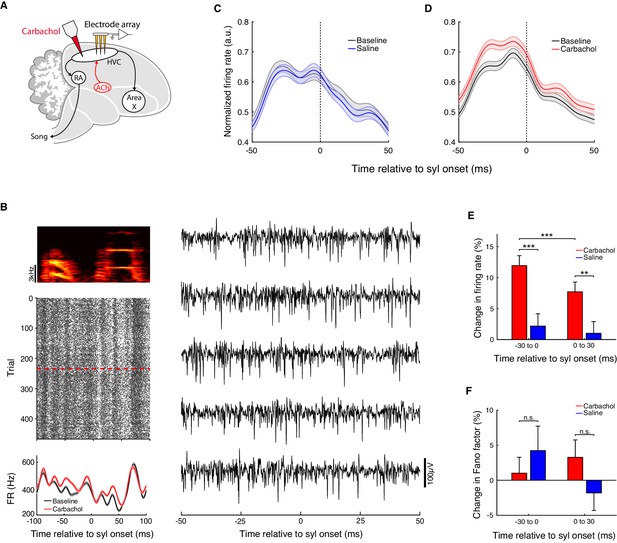
Carbachol increases HVC multi-unit firing rates.
(A) Experiment schematic. Carbachol was microdialyzed into HVC while recording neural activity with multi-electrode arrays. (B) Example multi-unit site (one experiment; activity aligned to the onset of one syllable). Top left, spectrogram of the syllable used for alignment. Middle left, raster plot of the multi-unit site (red dashed line: onset of carbachol). Bottom left, rendition-averaged firing rates (mean ± s.e.m., smoothed with a 5 ms SD gaussian kernel). Right, example raw traces for this multi-unit site (bandpass filtered between 300 and 4000 Hz). (C) Population average firing rates aligned to syllable onsets, before and after saline. Prior to averaging across sites/syllables, mean firing rates from both baseline and saline blocks were normalized by the maximum of both conditions in a 100 ms window centered on the syllable onset (n = 118 multi-unit sites x syllables, five birds, eight experiments). (D) Population average firing rates aligned to syllable onsets, before and after carbachol. Data are normalized as in panel C (n = 202 multi-unit sites x syllables, five birds, eight experiments). (E) Percent change in firing rate after switch to carbachol relative to baseline, or after switch to saline relative to baseline (mean ± s.e.m. increase in firing rate for Carb in −30 to 0 ms window: 12 ± 1.6%; Carb 0 to 30 ms: 7.7 ± 1.6%, Saline −30 to 0 ms: 2.2 ± 2.0%, Saline 0 to 30 ms: 1.0 ± 1.9%; Carb vs. Saline in −30 to 0 ms window, p=1.3e-4, two-tailed rank-sum test; Carb vs. Saline 0 to 30 ms, p=0.0039, two-tailed rank-sum test; Carb −30 to 0 ms vs. Carb 0 to 30 ms, p=5.4e-4, two-tailed signed-rank test). (F) Percent change in Fano factor after switch to carbachol relative to baseline, or after switch to saline relative to baseline (mean ± s.e.m. change in Fano factor for Carb in −30 to 0 ms window: 1.0 ± 2.3%; Carb 0 to 30 ms: 3.3 ± 2.5%, Saline −30 to 0 ms: 4.2 ± 3.5%, Saline 0 to 30 ms: −1.8 ± 2.5%; Carb vs. Saline in −30 to 0 ms window, p=0.73, two-tailed rank-sum test; Carb vs. Saline 0 to 30 ms, p=0.29, two-tailed rank-sum test). ***p<0.001, **p<0.01, n.s., not significant.
-
Figure 4—source data 1
Linear mixed effects model analysis of multi-unit firing rate changes following microdialysis of carbachol or saline.
- https://cdn.elifesciences.org/articles/53288/elife-53288-fig4-data1-v1.docx
-
Figure 4—source data 2
Source data for the summary analyses in figure panels C−F.
- https://cdn.elifesciences.org/articles/53288/elife-53288-fig4-data2-v1.mat
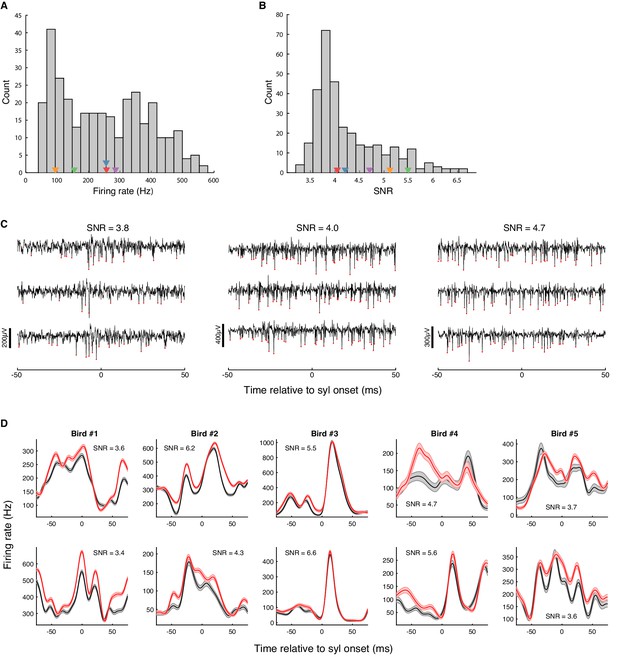
Additional characterization of multi-unit recordings from microdialysis experiments.
(A) Distribution of firing rates at baseline. Colored markers correspond to the mean for each bird (mean ± s.e.m. firing rate: 250 ± 7.9 Hz, n = 320 multi-unit sites x syllables, five birds; pre periods from carbachol and saline experiments combined, sites below 50 Hz excluded). (B) SNR distribution at baseline (see Methods for definition of SNR; mean ± s.e.m. SNR: 4.3 ± 0.040). (C) Example raw voltage traces from the quartiles of the SNR distribution (traces were bandpass filtered between 300 and 4000 Hz). Red dots indicate events that were detected as spikes. (D) Rendition-averaged firing rates aligned to syllable onsets before (black) and after (red) microdialysis of carbachol into HVC (mean ± s.e.m.; smoothed with 5 ms SD gaussian kernel). Examples of two multi-unit sites are shown for each of the five birds used in this study.
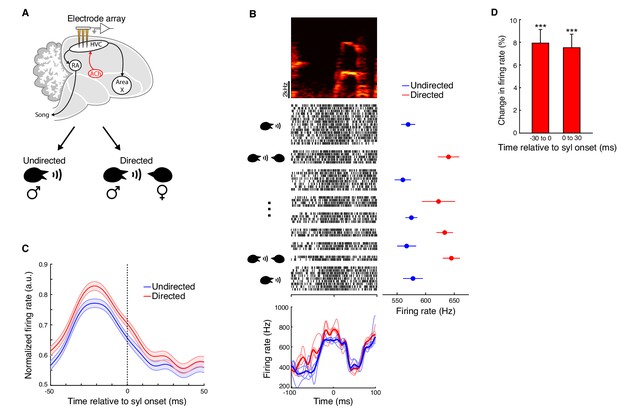
HVC activity is modulated by social context.
(A) Experiment schematic. Multi-unit activity in HVC was recorded during interleaved female-directed and undirected song sessions. (B) Example multi-unit site (one experiment; activity aligned to the onset of one syllable). Top, spectrogram of the syllable used for alignment. Middle, raster plot of the multi-unit site (activity plotted chronologically from top to bottom; spaces separate blocks of directed or undirected singing). Middle right, mean ± s.e.m. firing rates for each block of singing (firing rates were computed in a 100 ms window centered on the syllable onset). Bottom, rendition-averaged firing rates (smoothed with a 5 ms SD gaussian kernel; bold lines show mean firing rates for all renditions, light lines show mean firing rates for each block of directed or undirected song). (C) Population average firing rates aligned to syllable onsets, for directed and undirected conditions. Prior to averaging across sites/syllables, mean firing rates from directed and undirected renditions were normalized by the maximum of both conditions in a 100 ms window centered on the syllable onset (n = 151 multi-unit sites x syllables, five birds, 10 experiments). (D) Percent change in firing rate during directed song relative to undirected song (mean ± s.e.m. increase in firing rate in −30 to 0 ms window: 7.9 ± 1.2%; 0 to 30 ms window: 7.5 ± 1.2%; directed vs. undirected in −30 to 0 ms window: p=9.7e-11, 0 to 30 ms window: 1.4e-12, firing rate change in −30 to 0 ms window vs. 0 to 30 ms window: p=0.31, two-tailed signed-rank test). ***p<0.001.
-
Figure 5—source data 1
Linear mixed effects model analysis for comparing multi-unit firing rates in directed and undirected song.
- https://cdn.elifesciences.org/articles/53288/elife-53288-fig5-data1-v1.docx
-
Figure 5—source data 2
Source data for the summary analysis in figure panels C and D.
- https://cdn.elifesciences.org/articles/53288/elife-53288-fig5-data2-v1.mat
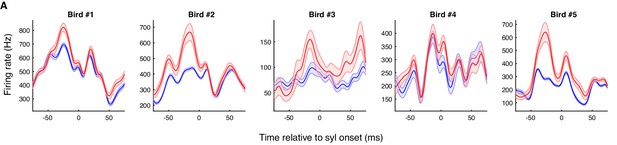
Example multi-unit sites from each bird demonstrating social modulation of HVC activity.
(A) Rendition-averaged firing rates aligned to syllable onsets for undirected (blue) and directed (red) trials (mean ± s.e.m.; smoothed with 5 ms SD gaussian kernel).
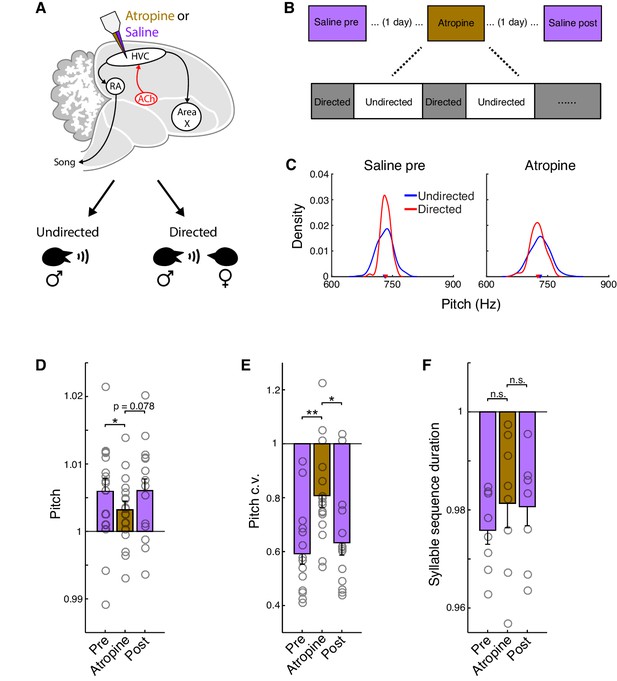
Atropine attenuates the social modulation of song.
(A) Experiment schematic. Atropine or saline was microdialyzed into HVC during interleaved female-directed and undirected song sessions. (B) Song was recorded on three separate days for each bird in the following order: Saline pre, Atropine, Saline post, with one day between sessions. See Methods for details. (C) Pitch distributions for one example syllable. (D) Normalized pitch (directed/undirected) for all syllables (mean ± s.e.m. increase in pitch for Saline pre: 0.59 ± 0.19%, Atropine: 0.32 ± 0.13%, Saline post: 0.60 ± 0.17%; Saline pre vs. Atropine, p=0.046, one-tailed signed-rank test; Saline post vs. Atropine, p=0.078, one-tailed signed-rank test). (E) Normalized pitch c.v. (directed/undirected) for all syllables (mean ± s.e.m. reduction in pitch c.v. for Saline pre: 41 ± 3.9%, Atropine: 19 ± 4.4%, Saline post: 37 ± 4.6%; Saline pre vs. Atropine, p=0.0028, one-tailed signed-rank test; Saline post vs. Atropine, p=0.012, one-tailed signed-rank test). For pitch and pitch c.v., n = 16 syllables, six birds, six experiments per condition. (F) Normalized syllable sequence duration (directed/undirected) for all syllable sequences (mean ± s.e.m. reduction in sequence duration for Saline pre: 2.4 ± 0.29%, Atropine: 1.9 ± 0.50%, Saline post: 1.9 ± 0.39%; Saline pre vs. Atropine, p=0.098, one-tailed signed-rank test; Saline post vs. Atropine, p=0.47, one-tailed signed-rank test, n = 8 syllable sequences, seven birds, seven experiments per condition). **p<0.01, *p<0.05, n.s., not significant.
-
Figure 6—source data 1
Linear mixed effects model analysis of atropine dialysis experiments during directed and undirected song.
- https://cdn.elifesciences.org/articles/53288/elife-53288-fig6-data1-v1.docx
-
Figure 6—source data 2
Source data for the summary analyses in figure panels D−F.
- https://cdn.elifesciences.org/articles/53288/elife-53288-fig6-data2-v1.mat
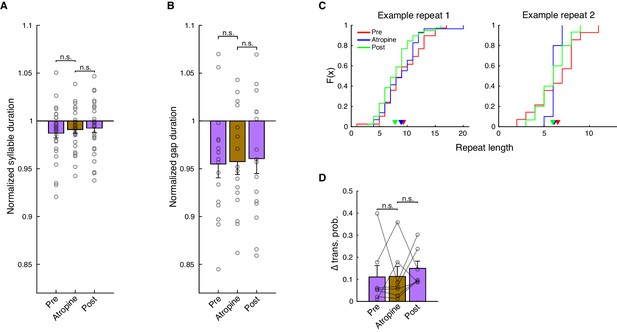
Social modulation of syllable durations, gap durations, and song sequencing.
(A) Normalized syllable durations (directed/undirected; mean ± s.e.m. reduction in syllable duration for Saline pre: 1.3 ± 0.51%, Atropine: 0.89 ± 0.41%, Saline post: 0.73 ± 0.51%; Saline pre vs. Atropine, p=0.15, one-tailed signed-rank test; Saline post vs. Atropine, p=0.78, one-tailed signed-rank test, n = 29 syllables, seven birds, seven experiments per condition). (B) Normalized gap durations (directed/undirected; mean ± s.e.m. reduction in gap duration for Saline pre: 4.5 ± 1.4%, Atropine: 4.3 ± 1.3%, Saline post: 3.9 ± 1.6%; Saline pre vs. Atropine, p=0.24, one-tailed signed-rank test; Saline post vs. Atropine, p=0.35, one-tailed signed-rank test, n = 16 gaps, five birds, five experiments per condition). (C) Empirical cumulative distribution functions of repeat length (# of syllable repetitions) for two repeated syllables that exhibited significant social modulation (distributions are from directed songs; test for increase in repeat length during directed vs. undirected, p<0.05 for both Saline pre and Saline post, one-tailed rank-sum test; n = 2 birds, two experiments per condition). Triangles depict the mean from each condition. In neither case did we observe a significant attenuation of this increase with atropine (mean ± s.e.m. increase in repeat length from undirected to directed, Saline pre: 49 ± 9.7%, atropine: 42 ± 5.7%, Saline post: 44 ± 20%; directed pre vs. directed atropine and directed post vs. directed atropine, p>0.05, one-tailed rank-sum test). (D) Change in transition probability from directed to undirected song (see Methods; mean ± s.e.m. change in transition probability for Saline pre: 0.11 ± 0.052; Atropine: 0.11 ± 0.046; Saline post: 0.15 ± 0.033; Saline pre vs. Atropine, p=0.53; Saline post vs. Atropine, p=0.29, one-tailed signed-rank test, n = 7 branch points, six birds, six experiments per condition). n.s., not significant.
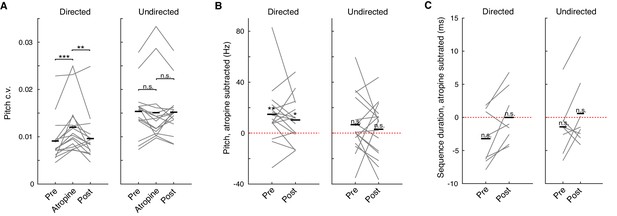
Directed but not undirected songs are affected by atropine.
(A) Pitch c.v. for all syllables from directed (left) and undirected (right) songs (mean ± s.e.m. pitch c.v. for directed pre: 0.0091 ± 0.0011, directed atropine: 0.012 ± 0.0013, directed post: 0.0096 ± 0.0012, undirected pre: 0.015 ± 0.0013, undirected atropine: 0.015 ± 0.0017, undirected post: 0.015 ± 0.0013; directed pre vs. directed atropine, p=0.00024; directed post vs. directed atropine, p=0.0039; undirected pre vs. undirected atropine, p=0.35; undirected post vs. undirected atropine, p=0.41). (B) Pitch for all syllables. For each syllable, we subtracted its atropine pitch value from both the pre and post saline pitch values. This aids visualization by reducing the variance within a condition, which is irrelevant. Note also that this transformation does not affect the test statistic used in the signed-rank test (mean ± s.e.m. pitch for directed pre: 15 ± 5.8 Hz, directed post: 10 ± 4.4 Hz, undirected pre: 6.6 ± 5.7 Hz, undirected post: 3.0 ± 5.3 Hz; directed pre vs. directed atropine, p=0.0045; directed post vs. directed atropine, p=0.037; undirected pre vs. undirected atropine, p=0.86; undirected post vs. undirected atropine, p=0.67). For pitch and pitch c.v., n = 16 syllables, six birds, six experiments per condition. (C) Sequence duration for all syllable sequences. For each syllable sequence, we subtracted its atropine sequence duration value from both pre and post measurements as in panel B (mean ± s.e.m. sequence duration for directed pre: −3.2 ± 1.4 ms, directed post: −0.0042 ± 1.5 ms, undirected pre: −1.4 ± 1.5 ms, undirected post: 0.58 ± 1.9 ms; directed pre vs. directed atropine, p=0.055; directed post vs. directed atropine, p=0.53; undirected pre vs. undirected atropine, p=0.93; undirected post vs. undirected atropine, p=0.63; n = 8 syllable sequences, seven birds, seven experiments per condition). All statistical tests in this figure were performed using the one-sided signed-rank test. For directed comparisons, we tested for attenuation of social modulation by atropine (e.g., we tested for a reduction in pitch for atropine relative to saline). For undirected comparisons, we tested the opposite tail (e.g., we tested for an increase in pitch for atropine relative to saline, to ensure that the reduction in normalized pitch for the atropine condition was not due to atropine causing an increase in pitch during undirected song). ***p<0.001, **p<0.01, *p<0.05, n.s., not significant.
Additional files
-
Source code 1
Source code for generating figures.
- https://cdn.elifesciences.org/articles/53288/elife-53288-code1-v1.m
-
Transparent reporting form
- https://cdn.elifesciences.org/articles/53288/elife-53288-transrepform-v1.docx