RNA-guided retargeting of Sleeping Beauty transposition in human cells
Figures
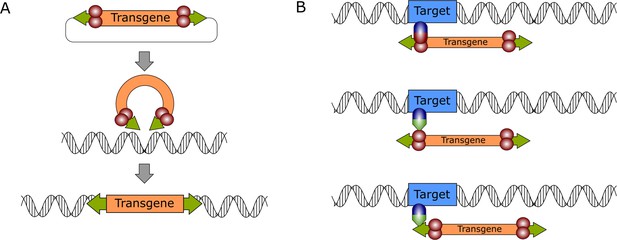
General mechanism of DNA transposition and molecular strategies for targeted gene integration.
(A) The transpositional mechanism of a DNA transposon in a biotechnological context. The transgene, which is flanked by transposon ITRs (green arrows) is excised from a plasmid by the transposase enzyme (red spheres), which is supplied in trans. The genetic cargo is then integrated in the target genome. (B) Transposition can be retargeted by foreign factors that can be DNA-binding domains (blue spheres) directly fused to the transposase or to adapter domains (green triangles) that interact either with the transposase (middle) or the transposon DNA (bottom).
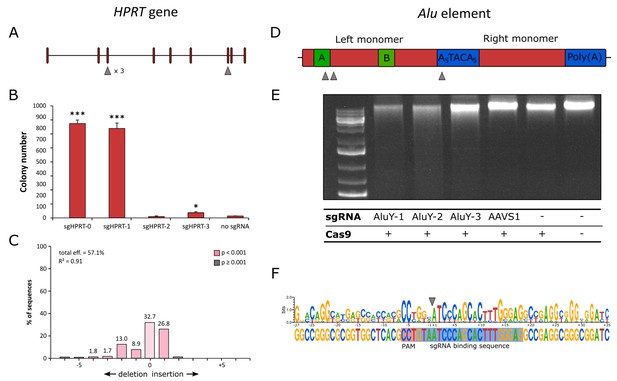
CRISPR/Cas9 components and their validation for transposon targeting.
(A) Schematic exon-intron structure of the HPRT gene and positions of the sgRNA binding sites. (B) Numbers of 6-TG resistant colonies after treatment with Cas9 and HPRT-directed sgRNAs. Significance is calculated in comparison to the no sgRNA sample (n = 3, biological replicates for all samples, *p≤0.05, ***p≤0.001, error bars represent SEM). (C) Indel spectrum of the HPRT locus after treatment with Cas9 and sgHPRT-0, as determined by TIDE assay. (D) Structure of an Alu element and relative positions of sgRNA binding sites. (E) Agarose gel electrophoresis of human gDNA digested with Cas9 and AluY-directed sgRNAs. An sgRNA targeting the human AAVS1 locus (a single-copy target) as well as samples containing no Cas9 or no sgRNA were included as negative controls. (F) Sequence logo generated by aligning sequenced gDNA ends after fragmentation with Cas9 and sgAluY-1 (the sequence represents the top strand targeted by the sgRNA). The position of the sgRNA-binding site and PAM is indicated by blue and red background, respectively. The cleavage site is marked by the gray arrow. The sequence upstream of the cleavage site is generated from 12 individual sequences, the sequence downstream is generated from 19 individual sequences. The bottom sequence represents the AluY consensus sequence.
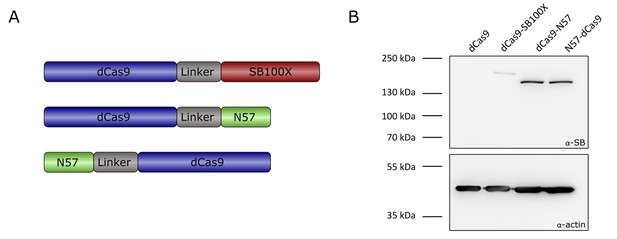
Transposase-derived targeting factors.
(A) Schematic representation of the targeting constructs. (B) Western blot of proteins expressed by the targeting constructs. The top half of the membrane was treated with α-SB antibody, the bottom half was treated with α-actin as a loading control. dCas9 was included as a negative control, and is therefore not expected to produce a signal with an antibody against the SB transposase. Expected sizes were 202.5 kDa for dCas9-SB100X and 169.7 kDa for dCas9-N57 and N57-dCas9.
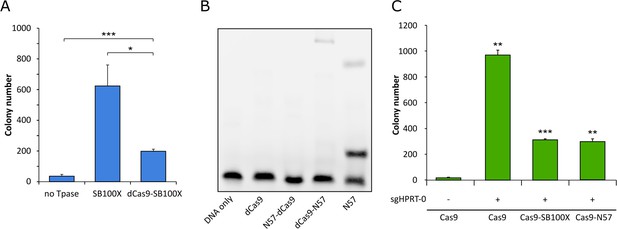
Functional testing of dCas9 fusions.
(A) Numbers of puromycin-resistant colonies in the transposition assay. The dCas9-SB100X fusion protein catalyzes ~30% as many integration events as unfused SB100X transposase (n = 3, biological replicates, *p≤0.05, ***p≤0.001, error bars represent SEM). (B) EMSA with dCas9-N57 fusion proteins. dCas9 serves as negative control, N57 as positive control. Binding can be detected for dCas9-N57, but not for N57-dCas9. The upper band in the positive control lane is likely a multimeric complex of DNA-bound N57 molecules, in line with N57’s documented activity in mediating protein-protein interaction between transposase subunits and in forming higher-order complexes (Izsvák et al., 2002). (C) Numbers of 6-TG resistant colonies after Cas9 cleavage. No disruption of the HPRT gene, as measured by 6-TG resistance over background, can be detected without the addition of an sgRNA. In the presence of sgHPRT-0, all Cas9 constructs cause significant disruption of the HPRT gene (n = 3, biological replicates, **p≤0.01, ***p≤0.001, error bars represent SEM).
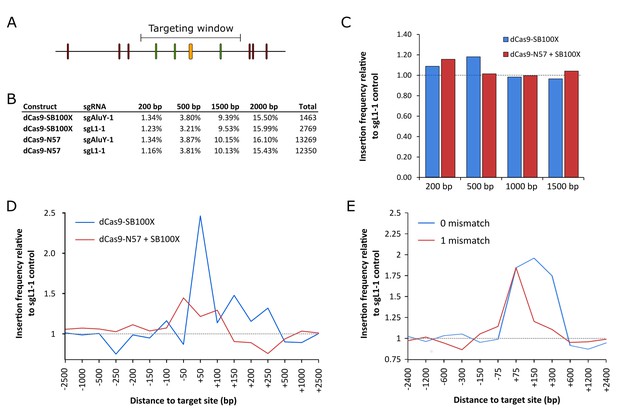
RNA-guided Sleeping Beauty transposition in human cells.
(A) Schematic representation of the analysis of SB retargeting. Targeting windows are defined as DNA extending a certain number of base pairs upstream or downstream of the sgRNA target sites (yellow – sgRNA target, green – ‘hit’ insertion, red – ‘miss’ insertion). (B) Percentages of integrations recovered from windows of different sizes along with the total numbers of integrations in the respective libraries. (C) Insertion frequencies relative to a dataset obtained with sgL1-1, in windows of various sizes around the targeted sites. Slight enrichment can be observed in a 200 bp window with dCas9-N57 and in a 500 bp window with dCas9-SB100X, although neither enrichment is statistically significant. The windows are cumulative, that is the 500 bp window also includes insertions from the 200 bp window. (D) Insertion frequencies in windows of various sizes, relative to a dataset obtained with sgL1-1, upstream and downstream of the target sites. Enrichment with dCas9-SB100X occurs downstream of the sgRNA target site, within a total insertion window of 300 bp (~1.5 fold enrichment, p=0.019). (E) The effect of the number of mismatches on the targeting efficiency of dCas9-SB100X. Relative insertion frequencies of the dCas9-SB100X sample into cumulative windows around perfectly matched target sites as well as sites with a single mismatch.
-
Figure 5—source data 1
Sleeping Beauty transposon integration sites obtained with dCas9-N57+SB100X and sgAluY-1.
- https://cdn.elifesciences.org/articles/53868/elife-53868-fig5-data1-v2.txt
-
Figure 5—source data 2
Sleeping Beauty transposon integration sites obtained with dCas9-N57+SB100X and sgL1-1.
- https://cdn.elifesciences.org/articles/53868/elife-53868-fig5-data2-v2.txt
-
Figure 5—source data 3
Sleeping Beauty transposon integration sites obtained with dCas9-SB100X and sgAluY-1.
- https://cdn.elifesciences.org/articles/53868/elife-53868-fig5-data3-v2.txt
-
Figure 5—source data 4
Sleeping Beauty transposon integration sites obtained with dCas9-SB100X and sgL1-1.
- https://cdn.elifesciences.org/articles/53868/elife-53868-fig5-data4-v2.txt
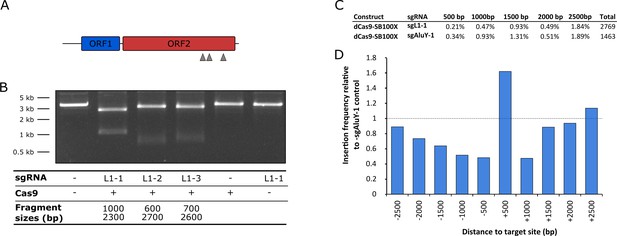
Design, in vitro validation and impact of sgRNAs against human L1 retrotransposon sequences.
(A) Schematic representation of the human L1 retrotransposon and relative positions of the sgRNA binding sites. (B) In vitro digestion of a ~ 3.3 kb plasmid fragment carrying the target sites of sgRNAs with purified Cas9 and the three L1-specific sgRNAs. All three sgRNAs resulted in digestion of the input DNA and the resulting fragments’ relative sizes match the expected values. (C) Fractions of insertions into cumulative windows around sgL1-1 target sites. (D) Relative insertion frequencies of SB in the presence of sgL1-1 as compared to insertion frequencies of SB in the presence of sgAlu-1. An overall depletion of insertions and some enrichment in a 500 bp window downstream of the sgL1-1 binding sites is apparent. However, these ratios are based on only a few insertions falling into the mapping windows, and therefore lack statistical significance.
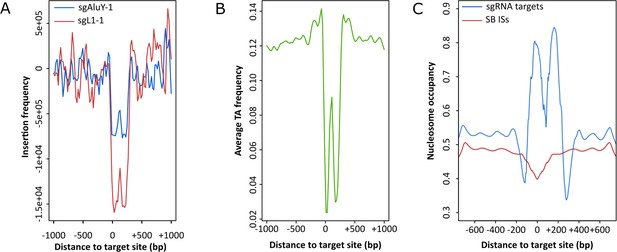
Analysis of targeted chromosomal regions.
(A) Insertion frequencies of the targeted (blue) and non-targeted (red) dataset show that statistically significant (p=0.019) enrichment occurs within a 300 bp window downstream of sites targeted by sgAluY-1, which is generally disfavored for SB integration. (B) Reduced average TA di-nucleotide frequency the targeted 300 bp window. (C) Computationally predicted nucleosome occupancy around the sites targeted by sgAluY-1 (blue) and around untargeted SB insertion sites (ISs, red).
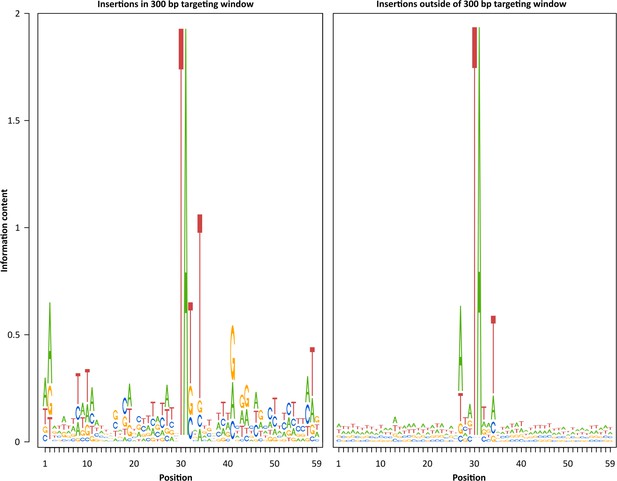
Sequence logos generated from sequences around insertion sites catalyzed by dCas9-SB100X with sgAluY-1 within the 300 bp targeting window (left) and outside of the window (right). The left logo has higher variation at most positions, because of the lower number of insertions.
Additional files
-
Supplementary file 1
Sequences of DNA oligos used in this study.
- https://cdn.elifesciences.org/articles/53868/elife-53868-supp1-v2.docx
-
Transparent reporting form
- https://cdn.elifesciences.org/articles/53868/elife-53868-transrepform-v2.docx