A conserved RNA degradation complex required for spreading and epigenetic inheritance of heterochromatin
Figures
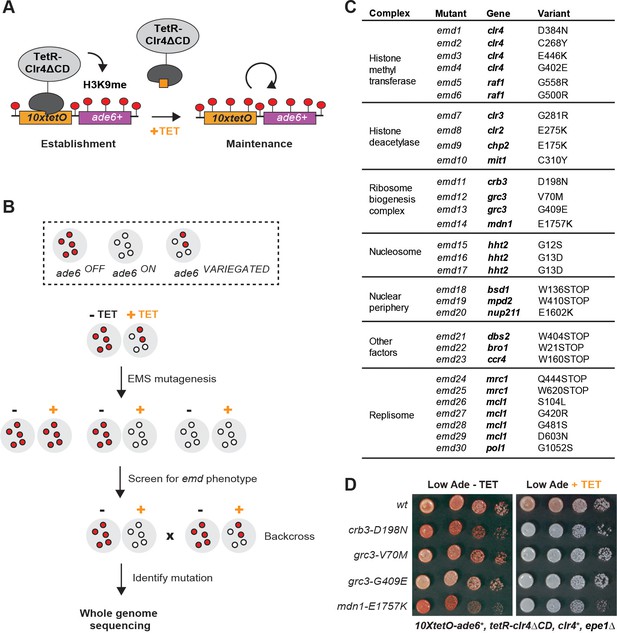
A Genetic Screen Identifies Factors Essential for Heterochromatin Inheritance.
(A) Schematic diagram representing an inducible reporter system for heterochromatin establishment and inheritance (Ragunathan et al., 2015, Audergon et al., 2015). 10xtetO, 10 copies of the tet operator; TetR-Clr4ΔCD, fusion of TetR and Clr4 lacking the chromodomain; H3K9me, di- and tri-methyl histone H3 Lys 9; TET, tetracycline. (B) Schematic diagram of the screen design. EMS, ethyl methanesulfonate; emd, epigenetic maintenance defective. (C) List of mutations identified by whole genome sequencing, with their respective genes and the protein complexes that they are known to associate with, where applicable. (D) Silencing assay on low adenine medium in the absence (-TET) or presence (+TET) of tetracycline for mutants emd11-14, carrying point mutations in subunits of the rixosome. Ade, adenine.
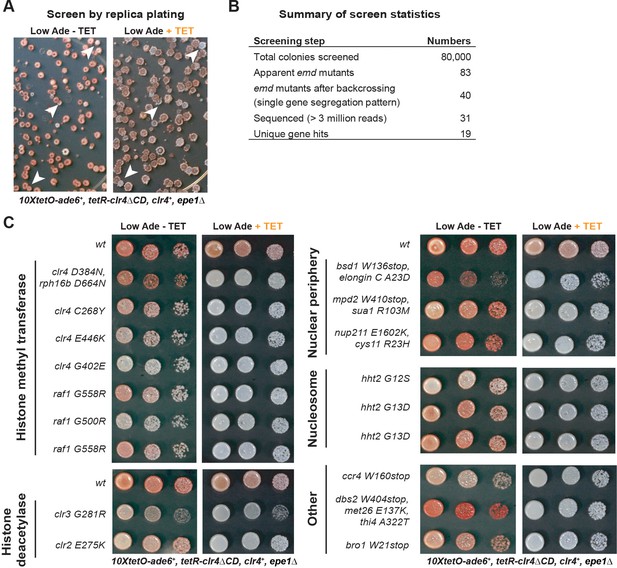
Screen for Isolation of Inheritance Defective Mutants.
(A) Representative images of plate replicas on low adenine medium lacking or containing tetracycline. Colonies were tracked between the replicas and those red on -TET and white on +TET plates were selected for further analysis (white arrows). (B) Summary of screen data. (C) Silencing assays for mutants isolated from the screen, together with the top hits recovered by whole genome sequencing. In some mutants, multiple linked variants were detected with high confidence (present in >90% of pooled mutant spores) and are listed together here.
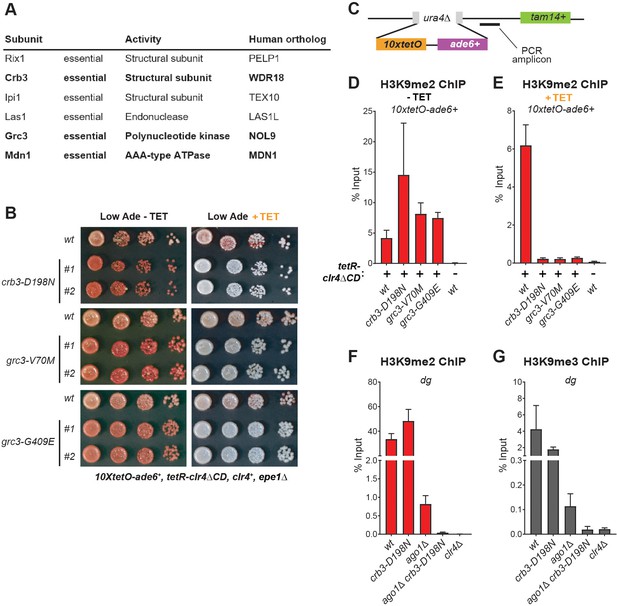
The Rixosome is Required for the Inheritance of Heterochromatin.
(A) Subunits of the rixosome, their specific activities in the context of ribosome biogenesis, and their human orthologs. Subunits identified in our screen are highlighted in bold. (B) Silencing assays to validate the phenotypes of mutants derived from the screen. Three mutations in rixosome subunits were reconstituted de novo in 10xtetO-ade6+ reporter cells and plated on low adenine medium in the presence or absence of tetracycline to assess heterochromatin establishment and maintenance. (C) Map of the 10xtetO-ade6+ reporter inserted at the endogenous ura4+ locus. The position of the primer pair used for ChIP-qPCR (PCR amplicon) experiments in panels D and E is indicated. (D, E) Chromatin immunoprecipitations (ChIPs) for di-methylated histone H3 Lys 9 (H3K9me2) at the 10xtetO-ade6+ reporter under establishment and maintenance conditions in epe1Δ cells, carrying wild-type or mutant rixosome subunits. (F, G) ChIP for H3K9me2 and H3K9me3 at the pericentromeric dg repeats. Mutations in the rixosome subunit crb3 abolish H3K9me2 and me3 in the absence of ongoing establishment by the RNAi pathway.
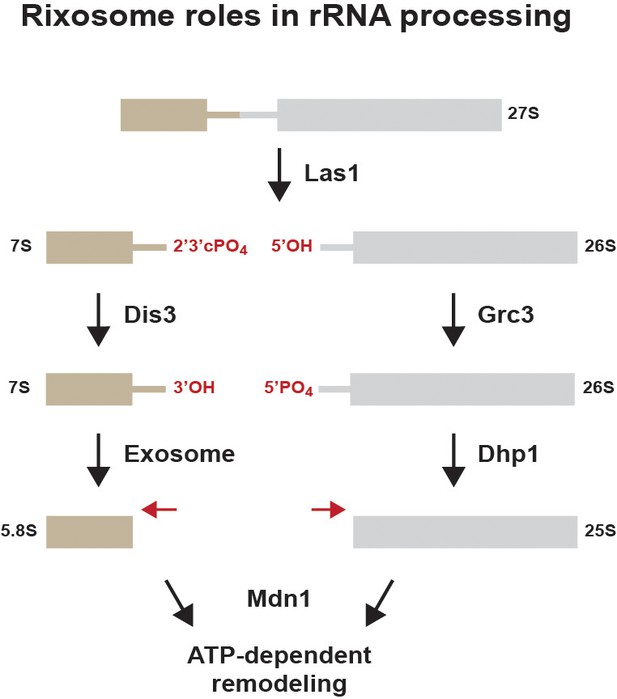
Summary of previously described rixosome functions during ribosome biogenesis (Gasse et al., 2015).
The 27S rRNA precursor is cleaved by the endonuclease Las1, creating a free 5’ hydroxyl (5’OH) group and a 2’3’ cyclic phosphate (2’3’PO4). The 3’ end is processed by the exosome. The 5’ end is phosphorylated by the polynucleotide kinase activity of Grc3 to produce 5’PO4 and processed by the 5’−3’ exonuclease Dhp1 (XRN2). The AAA+ ATPase Mdn1 remodels the RNA-protein complex to release chaperones to produce mature 60S ribosomal subunits.
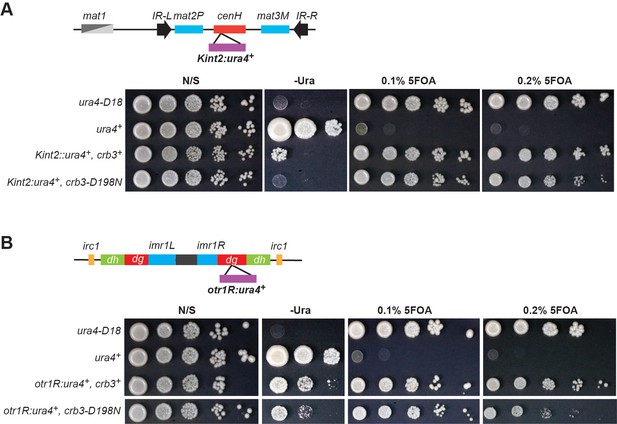
Heterochromatin establishment at endogenous heterochromatic domains is largely unaffected in rixosome mutations.
(A) Top: Schematic diagram of DNA sequence organization of the mating type locus with position of the Kint2::ura4+ reporter. cenH, centromere homology region; mat2P and mat3M, silent mating type loci; IR-L and IR-R, boundary elements. Bottom: Silencing assays for Kint2::ura4+ expression on nonselective medium (N/S), medium lacking uracil (-Ura), or medium supplemented with 0.1% and 0.2% 5-Fluoroorotic acid (5FOA). ura4-D18, a null allele, and ura4+ cells were used as controls. The higher 5FOA concentration allows the detection of minor defects in heterochromatic silencing. (B) Top: Schematic diagram of DNA sequence organization of the pericentromeric repeats on chromosome 1 and position of the otr1R:ura4+ reporter. otr, outermost repeats; dg and dh, outermost dg and dh repeats; imr, innermost repeats; irc, inverted repeat centromere sequences. Bottom: Silencing assays for otr1R:ura4+ expression on nonselective medium (N/S), medium lacking uracil (-Ura), or medium supplemented with 5FOA. ura4-D18, a null allele, and ura4+ cells were used as controls.
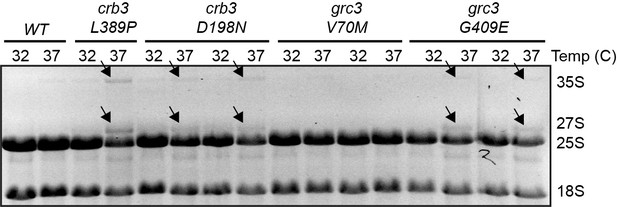
Effects of the rixosome mutants on rRNA processing A denaturing gel of total RNA, EtBr staining.
Mature rRNA species are labelled as 25S and 18S, rRNA precursors as 27S and 35S. The presence of the higher molecular weight precursors indicates rRNA processing defects.
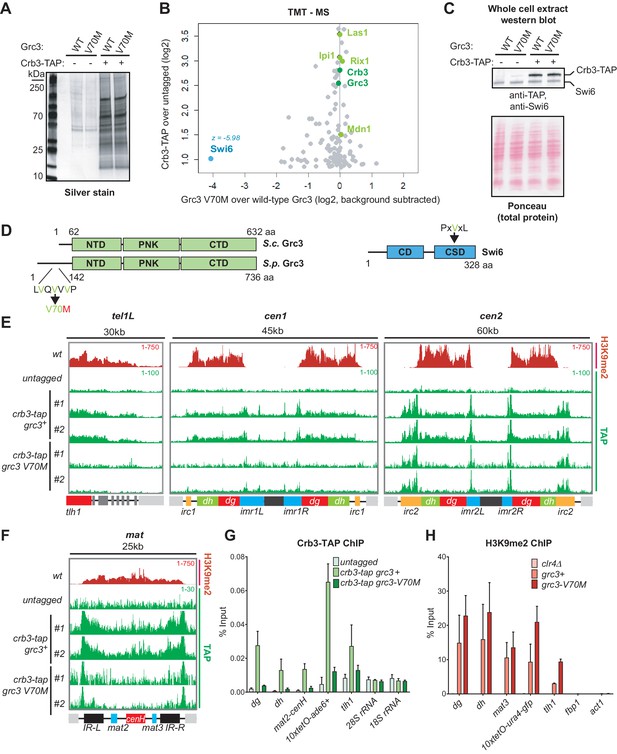
A mutation in the rixosome Grc3 subunit abolishes its interaction with the HP1 protein Swi6 and releases the mutant complex from heterochromatin.
(A) Silver staining of immunoprecipitations of wild-type and grc3-V70M mutant rixosome complexes from cell extracts. (B) Tandem mass tag mass spectrometry (TMT-MS) of the immunoprecipitations in A. Subunits of rixosome highlighted in green, HP1 protein Swi6 in blue, ribosome biogenesis and other factors in gray. For detailed description of TMT-MS data analysis see the STAR Methods section. (C) Top, western blot from whole cell extracts for the rixosome subunit Crb3-TAP and the HP1 protein Swi6 from grc3+ and grc3-V70M cells. Bottom, ponceau staining is shown as a loading control. (D) Schematics of domain organizations of Grc3 (left) and Swi6 (right). Homologous regions of S. pombe (S.p.) Grc3 and S. cerevisiae (S.c.) Grc3 are indicated (Pillon et al., 2017). The extended N-terminus of S.p. Grc3 lacks similarity to S.c. Grc3. The position and amino acid context of the V70M mutation are shown. The three residues highlighted in green are a variant of a consensus motif (PxVxL), recognized by the chromoshadow domain of HP1 proteins. NTD, N-terminal domain; PNK, polynucleotide kinase domain; CTD, C-terminal domain; CD, chromodomain; CSD, chromoshadow domain. (E, F) ChIP sequencing profiles of H3K9me2 and Crb3-TAP at heterochromatin in grc3+ and grc3-V70M cells. tel, telomere; cen, centromere; mat, mating type locus; kb, kilobases. Numbers in top right corner of each panel show reads per million. For detailed notations, see Figure 2—figure supplement 1 legend. (G, H) ChIP-qPCR quantification of rixosome and H3K9me2 enrichment at different heterochromatic regions in wild-type and grc3-V70M mutant epe1Δ cells. dg and dh, tandem repeats in pericentromeric DNA repeats; tlh1, subtelomeric gene; act1 and fbp1, euchromatic controls.
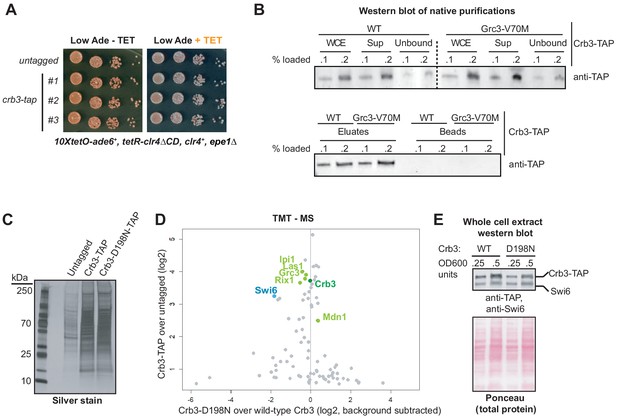
Identification of proteins associated with wild-type and crb3-D198N mutant complexes.
(A) Silencing assay in cells carrying TAP-tagged or untagged Crb3, showing that the presence of the tag does not disrupt establishment or maintenance of silencing at the 10xtetO-ade6+ reporter locus. (B) Western blot of different fractions from immunoprecipitations of the rixosome. The complex is efficiently solubilized and immunodepleted from whole cell extracts (WCEs), as seen from the amounts of Crb3-TAP in the WCE, supernatant incubated with IgG beads (Sup), and unbound fractions. (C) Silver staining of immunoprecipitations of wild-type and crb3-D198N mutant rixosome complexes from cell extracts. (D) TMT-MS of the immunoprecipitations in D. Rixosome subunits highlighted in green, HP1 protein Swi6 in blue and other factors in gray. (E) Top, western blot with anti-TAP and anti-HP1/Swi6 antibody on crb3-tap and crb3-D198N-tap whole cell extracts. Bottom, ponceau staining is shown as a loading control.
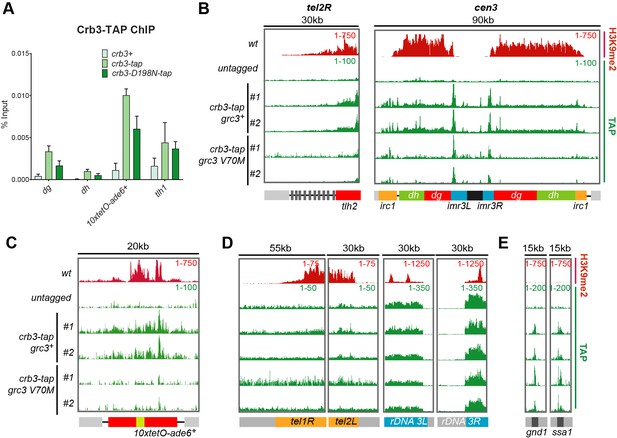
Recruitment of rixosome complexes to heterochromatin in wild-type, crb3-D198N and grc3-V70M cells.
(A) Quantification of rixosome enrichment at different heterochromatic regions in wild-type and crb3-D198N mutant cells by ChIP-qPCR. All strains are epe1Δ. (B, C, D) ChIP sequencing profiles of H3K9me2 and Crb3-TAP at heterochromatic loci in grc3+ and grc3-V70M cells. Numbers in top right corner of each panel denote reads per million. For detailed notations, see Figure 2—figure supplement 1 legend. (E) ChIP-seq profiles of H3K9me2 and Crb3-TAP at euchromatic regions in grc3+ and grc3-V70M cells. Numbers in top right corner of each panel denote reads per million.
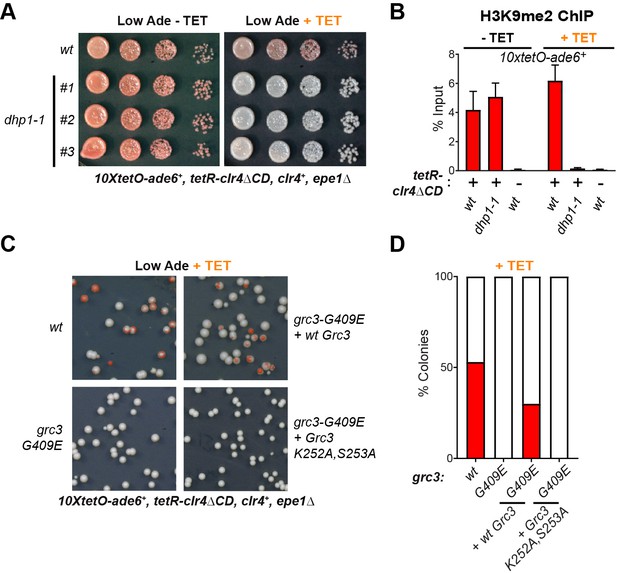
The 5’−3’ exonuclease Dhp1/Xrn2 and the catalytic activity of the rixosome subunit Grc3 are essential for epigenetic inheritance of gene silencing.
(A) Silencing assay for heterochromatin establishment and maintenance at the 10xtetO-ade6+ reporter locus in a Dhp1/Xrn2 hypomorphic mutant, dhp1-1. (B) ChIP for H3K9me2 at the 10xtetO-ade6+ reporter locus under establishment and maintenance conditions in cells carrying dhp1+ or dhp1-1 alleles. For primer positions, see Figure 2C. (C) Rescue of maintenance defect by expression of wild-type or catalytically dead (K252A, S253A) Grc3 in grc3-G409E cells. Silencing assay on low adenine medium shows that catalytic activity is essential to restore maintenance.(D) Quantification of the plating assays in (C).
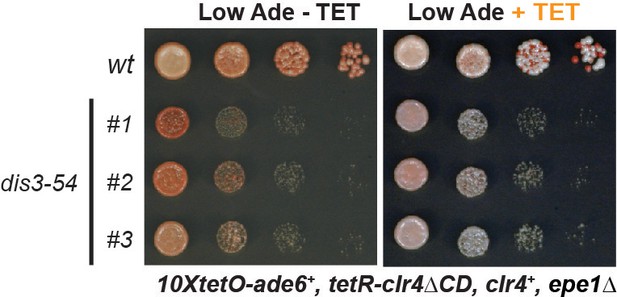
A mutation that impairs exosome function does not affect heterochromatin maintenance.
Silencing assay for heterochromatin maintenance at the 10xtetO-ade6+ reporter locus in the exosome mutant dis3-54.
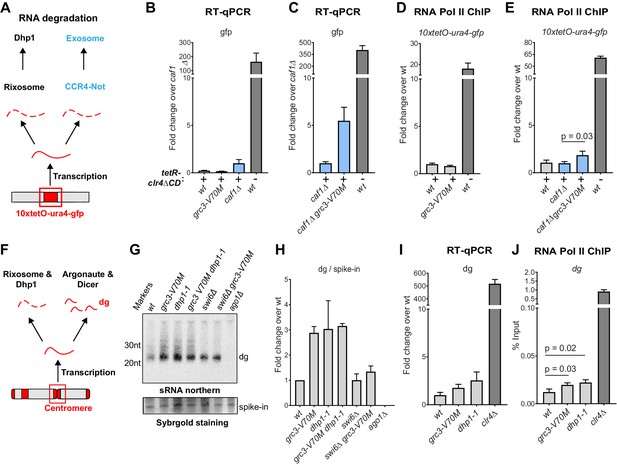
The rixosome channels heterochromatic RNAs for degradation via the 5’−3’ exonuclease Dhp1/Xrn2.
(A) Diagram of possible RNA degradation pathways at the 10xtetO-ura4-gfp/ade6+ reporter. The rixosome cleaves RNAs produced from the reporter on heterochromatin and targets them for degradation by the nuclear Dhp1/Xrn2 exonuclease. If an RNA escapes degradation by the rixosome, it is deadenylated by the Ccr4-Not complex, in which Caf1 is a key subunit, in preparation for decapping and degradation by the cytoplasmic exosome. Deletion of the deadenylation pathway unmasks the effect of the rixosome and Xrn2/Dhp1 on reporter RNAs. (B, C) Reverse transcription (RT) -qPCR assay for RNA accumulation at the 10xtetO-ura4-gfp/ade6+ reporter locus in wild-type, grc3-V70M and caf1Δ cells (B) and in caf1Δ and caf1Δ grc3 cells (C). All strains are also epe1Δ. Caf1 is a subunit of the major cytoplasmic RNA deadenylase complex, Ccr4-Not. (D, E) ChIP for RNA polymerase II (Pol II) at the reporter locus in wild-type and grc3-V70M cells (D) and in wild-type, caf1Δ and caf1Δ grc3-V70M cells (E), also carrying epe1Δ. (F) Model for the role of the rixosome in RNA degradation at the pericentromeric repeats. The rixosome acts on full length RNAs transcribed from the repeats in competition with RNAi and targets them for degradation through the exonuclease Xrn2/Dhp1. When the rixosome is released from heterochromatin or when Dhp1 activity is reduced, dg transcripts normally degraded by the rixosome and Dhp1 are channeled for processing by RNAi, resulting in increased siRNA accumulation. (G) Small RNA Northern blot, measuring the accumulation of small RNAs originating from the pericentromeric dg repeats in wild-type and different mutants. A 20mer oligoribonucleotide was added to each sample prior to purification as an internal loading control and used for normalization. Fold changes with standard deviations relative to the spike-in 20mer are shown below each lane. See Figure 5—figure supplement 1E for uncropped images. (H) Quantification of the blot from panel G for dg siRNAs, normalized to the internal spike-in control. The plot displays two data points, their mean and standard deviation for each genotype. See Figure 5—figure supplement 1D for normalization using snoR69. (I) ChIP for RNA polymerase II (Pol II) at the pericentromeric dg repeats.
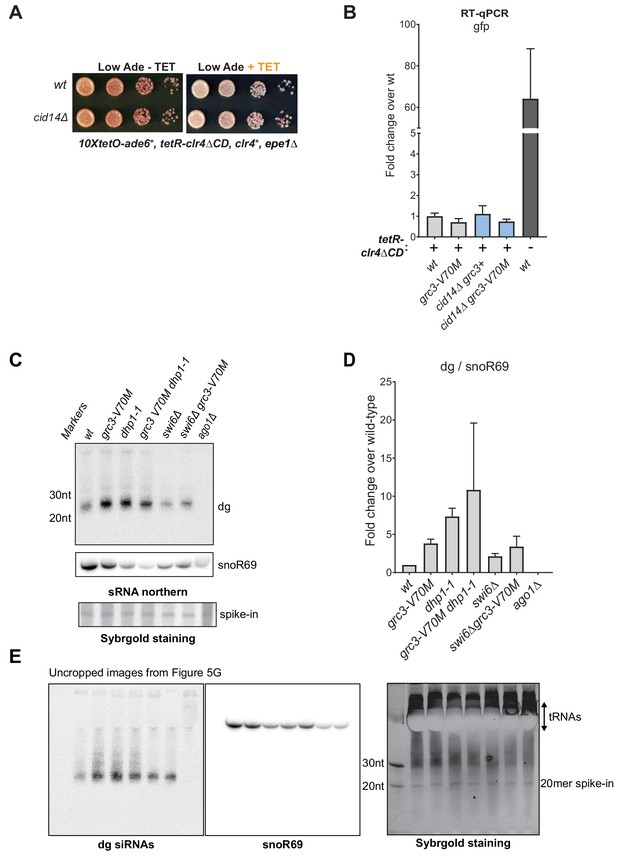
Extended data for RNA degradation at heterochromatin.
(A) Silencing assay for heterochromatin maintenance at the 10xtetO-ade6+ reporter locus in cells lacking the Cid14 subunit of the TRAMP complex (cid14Δ). The TRAMP complex targets RNAs for degradation by the exosome in the nucleus. (B) RT-qPCR assay for RNA accumulation at the 10xtetO-ura4-gfp reporter locus for wild-type and grc3-V70M mutant rixosome in wild-type and cid14Δ cells. All strains also carry epe1Δ allele. Loss of Cid14 had no effect on the levels of RNAs originating from the 10xtetO-ura4-gfp reporter. (C) Small RNA northern blot showing the accumulation of small RNAs originating from the pericentromeric dg repeats in wild-type and the indicated mutant cells. A 20mer oligoribonucleotide was added to each sample prior to purification as an internal loading control and used for normalization shown in Figure 5H. (D) Quantification of dg signal, normalized to snoR69, another control commonly used for small RNA northern blots, from panel C and E. The plot displays two data points, their mean and standard deviation for each genotype. (E) Uncropped images of the northern blots for dg siRNAs (left) and snoR69 (middle) and of the SybrGold-stained sRNA gel (right) from Figure 5G. Distortions caused by the strong tRNA bands overlapping snoR69 may result in its variable transfer to the northern membrane.
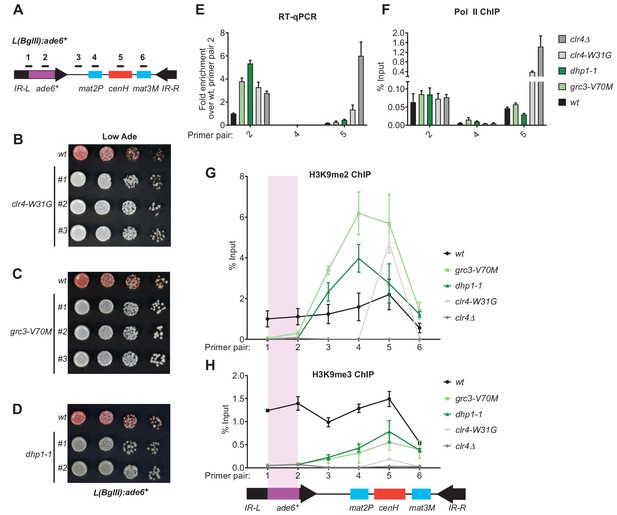
Rixosome and Dhp1 are required for RNAi-independent heterochromatin spreading and promote the Clr4-catalyzed transition H3K9me2 to H3K9me3.
(A) Schematic of DNA sequence organization of the mating type locus with position of the L(BglII):ade6+ reporter and centromere homology (cenH, red) region highlighted. For detailed notations, see Figure 2—figure supplement 1 legend. (B, C, D) Silencing assays of the L(BglII):ade6+ reporter in wild type, clr4-W31G, grc3-V70M and dhp1-1 cells. (E) Qunatitative Reverse Transcription PCR (qRT) for transcripts along the mating type locus in wild type cells and cells carrying clr4-W31G, grc3-V70M, dhp1-1, and clr4∆ mutant alleles. (F) ChIP-qPCR for RNA Polymerase II occupancy along the mating type locus in wild type cells and cells carrying clr4-W31G, grc3-V70M, dhp1-1, and clr4∆ mutant alleles. (G, H) ChIP-qPCR for H3K9me2 and H3K9me3 at the L(BglII):ade6+ reporter and surrounding regions at the mating type locus in wild type cells and cells carrying clr4-W31G, grc3-V70M, dhp1-1, and clr4∆ mutant alleles.
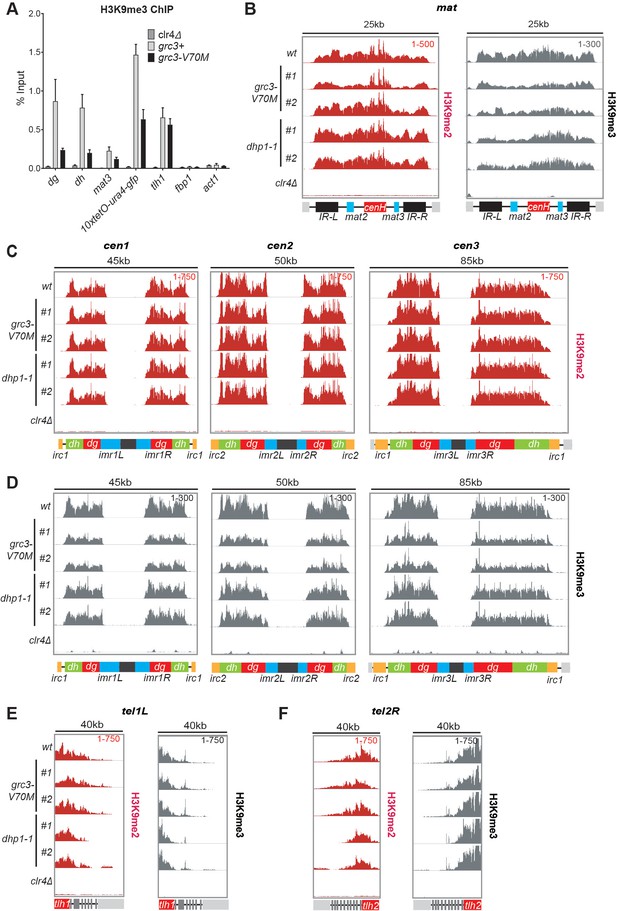
The rixosome and Dhp1 are required for efficient H3K9 tri-methylation.
(A) Quantification by ChIP-qPCR of tri-methylation levels at different heterochromatic domains in grc3-V70M and wild-type cells, carrying epe1Δ deletion. Error bars represent standard deviation for three biological replicates. (B, C, D) ChIP-seq profiles of H3K9me2 and H3K9me3 at the pericentromeric regions of chromosomes 1, 2 and 3 (cen1, cen2 and cen3) and the mat locus. Numbers in top right corner of each panel denote reads per million. For detailed notations, see Figure 2—figure supplement 1 legend. (E, F) ChIP-seq profiles of H3K9me2 and H3K9me3 at the subtelomeric loci tlh1 and tlh2 in wild-type, grc3-V70M and dhp1-1 cells. Numbers in top right corner of each panel denote reads per million. Panels for H3K9me2 in wild-type cells in (B), (C), (E) and (F) use data shown in Figure 3 (E) and (F), and are shown here for ready comparison.
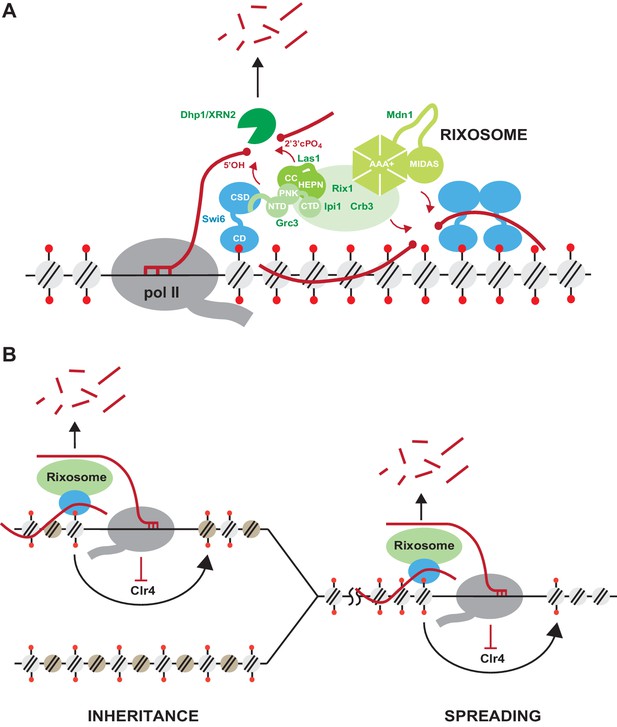
Model for rixosome-mediated degradation of nascent transcripts and its role in spreading and inheritance of heterochromatin.
(A) The rixosome localizes to heterochromatin via a direct interaction between the N-terminus of Grc3 with Swi6. The endonuclease Las1 and the polynucleotide kinase (PNK) Grc3, subunits of the rixosome, cleave nascent (left, pol II-associated) and/or mature chromatin-associated (right) transcripts and phosphorylate the free 5’ hydroxyl end at the site of cleavage, respectively. These processing steps prepare the RNA for subsequent degradation by the Dhp1/XRN2 exonuclease. CC, coiled coil domain; HEPN, higher eukaryotes and prokaryotes nucleotide-binding domain; AAA+, ATPase domain; pol II, RNA polymerase II. (B) Heterochromatic RNA clearance enables the spreading and inheritance of heterochromatin by Clr4-mediated read-write. Nascent transcripts and their associated transcriptional complexes, or mature RNAs retained at chromatin by heterochromatic proteins, present obstacles in the path of the read-write pathway. By degrading these RNAs, the rixosome facilitates read-write-dependent spreading and inheritance of heterochromatin.
Tables
Reagent type (species) or resource | Designation | Source or reference | Identifiers | Additional information |
---|---|---|---|---|
Strain, strain background (Schizosaccharomyces pombe) | various | This paper | see Supplementary file 1 | |
Antibody | Anti-H3K9me2 antibody (mouse monoclonal) | Abcam | Ab1220 | ChIP. 2 µg |
Antibody | 8WG16 (mouse monoclonal) | Biolegend | MMS-126R-500 | ChIP, 4 µg |
Antibody | Anti-H3K9me3 (monoclonal, recombinant) | Diagenode | C15500003 | ChIP, 1 µg |
Antibody | Rabbit IgG (rabbit, polyclonal) | Sigma | 15006 | ChIP, 1.5 µg |
Antibody | Peroxidase Anti-Peroxidase Soluble Complex antibody (rabbit) | Sigma | P1291 | Western, 1:2000 |
Antibody | anti-Swi6 antibody (rabbit, polyclonal) | PMID:25730778 | Western, 1:5000 | |
Antibody | IRDye 700DX-conjugated secondary antibody (goat, anti-rabbit) | VWR | RL611-130-122 | Western, 1:10000 |
Sequence-based reagent | Oligonucleotides | This paper | Supplementary file 2 | |
Commercial assay or kit | ZR Fungal/Bacterial DNA MiniPrep | Zymoresearch | 11–321 | |
Commercial assay or kit | QIAquick PCR Purification Kit | Qiagen | 28106 | |
Commercial assay or kit | Silver Stain SNAP Kit | Thermo Fisher Scientific | 24612 | |
Commercial assay or kit | RNeasy Mini Kit | Qiagen | 74106 | |
Commercial assay or kit | mirVana miRNA isolation kit | Ambion | AM1561 | |
Commercial assay or kit | Qubit dsDNA high sensitivity kit | Invitrogen | Q32854 | |
Chemical compound, drug | Ethyl methanesulfonate | Sigma Aldrich | M0880-5G | |
Chemical compound, drug | Anhydrotetracycline (hydrochloride) | Thermo Fisher Scientific | 10009542100 MG | |
Chemical compound, drug | 5-fluoroorotic acid | Goldbio | F-230–10 | |
Chemical compound, drug | EGS (Ethylene glycol bis[succinimidylsuccinate]) | Pierce (Fisher) | 21565 | |
Chemical compound, drug | Formaldehyde, 37% | Thermo Fisher Scientific | F79-500 | |
Chemical compound, drug | PMSF | Thermo Fisher Scientific | 36978 | |
Chemical compound, drug | cOmplete, EDTA-free Protease Inhibitor Cocktail Tablets | Sigma-Aldrich | 05056489001 | |
Chemical compound, drug | Dynabeads Protein A | Invitrogen | 100-02D | |
Chemical compound, drug | Dynabeads M-270 Epoxy | Invitrogen | 14301 | |
Chemical compound, drug | Dynabeads M-280 Streptavidin 10 ml | Fisher | 11206D | |
Chemical compound, drug | Dynabeads Pan Mouse IgG | Invitrogen | 110–41 | |
Chemical compound, drug | trypsin | Promega | V5111 | |
Chemical compound, drug | TMT-10plex reagent | Thermo Fisher Scientific | 90406 | |
Chemical compound, drug | Superscript III reverse transcriptase | Invitrogen | 18080–044 | |
Chemical compound, drug | TURBO DNase (2 U/µL) | Thermo Fisher | AM2239 | |
Chemical compound, drug | 4–20% Mini-PROTEANÒTGX Precast Protein Gels | Biorad | 4561093 | |
Chemical compound, drug | 0.5 mm glass beads 10 lbs bulk container | BioSpec | 11079105 | |
Chemical compound, drug | Proteinase K | Sigma-Aldrich | 03115828001 | |
Chemical compound, drug | Phenol:Chloroform:Isoamyl Alcohol 25:24:1 Saturated | Sigma-Aldrich | P2069-100ML | |
Chemical compound, drug | Acid-Phenol: Chloroform, pH 4.5 | Thermo Fisher Scientific | AM9720 | |
Software, algorithm | Mudi | PMID:24766403 | T. Iida | |
Software, algorithm | Bowtie | https://doi.org/10.1186/gb-2009-10-3-r25 | ||
Software, algorithm | IGV | https://www.broadinstitute.org/igv/ | ||
Software, algorithm | Geneious | http://www.geneious.com, PMID:22543367 | ||
Software, algorithm | DeepTools | PMID:27079975 |
Additional files
-
Supplementary file 1
List of S. pombe strains used in this study.
This Table outlines the list of fission yeast S. pombe strains that were used in this study and notes the specific figure in the paper in which each strain was used.
- https://cdn.elifesciences.org/articles/54341/elife-54341-supp1-v1.docx
-
Supplementary file 2
List of oligonucleotides used in this study.
List of DNA oligonucleotides used in RT-qPCR, ChIP-qPCR and northern blots.
- https://cdn.elifesciences.org/articles/54341/elife-54341-supp2-v1.docx
-
Supplementary file 3
The results of quantitative mass spectrometry experiments for the purification of Grc3-V70M mutant rixosome complexes.
List of proteins identified in purifications of wild-type and Grc3-V70M mutant rixosome complexes using isobaric tandem mass tags (TMT) mass spectrometry.
- https://cdn.elifesciences.org/articles/54341/elife-54341-supp3-v1.xlsx
-
Supplementary file 4
The results of quantitative mass spectrometry experiments for the purification of Crb3-D198N mutant rixosome complexes.
List of proteins identified in purifications of wild-type and Crb3-D198N mutant rixosome complexes using isobaric tandem mass tags (TMT) mass spectrometry.
- https://cdn.elifesciences.org/articles/54341/elife-54341-supp4-v1.xlsx
-
Transparent reporting form
- https://cdn.elifesciences.org/articles/54341/elife-54341-transrepform-v1.pdf