A loop structure allows TAPBPR to exert its dual function as MHC I chaperone and peptide editor
Figures
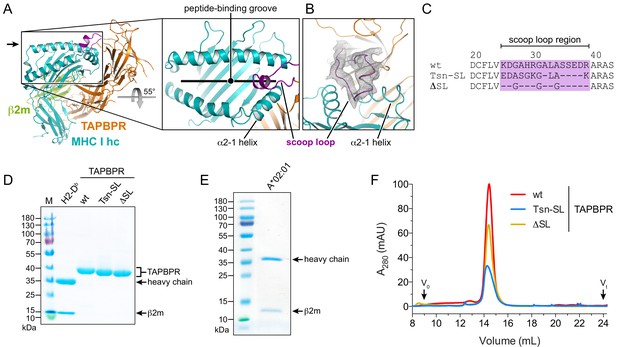
Expression and purification of different TAPBPR scoop-loop variants and MHC I chaperone clients.
(A) X-ray structure of the TAPBPR-MHC I complex in cartoon representation (PDB ID: 5OPI). The zoom-in shows how the TAPBPR scoop loop (purple) is inserted into the F-pocket region of the MHC I peptide-binding groove that is occupied by the C terminus of the peptide before peptide displacement. (B) 2Fo-Fc electron density of the X-ray structure in the region of the scoop loop, contoured at 0.8σ. The width of the helix cartoons has been reduced to facilitate visualization of the electron density. The viewing direction is indicated by the black arrow in panel (A). (C) Sequence alignment of the scoop-loop region in the TAPBPR constructs used in this study. (D, E) Purified proteins used in the current study were analyzed by non-reducing SDS-PAGE. The MHC I allomorphs H2-Db (mouse) and HLA-A*02:01 (human) were refolded in the presence of β2m and peptide. (F) The TAPBPR proteins, injected at different concentrations to facilitate comparison, eluted as monodisperse samples during size-exclusion chromatography (SEC). Abbreviations: MHC I hc: MHC I heavy chain; wt: wildtype; Tsn: tapasin; SL: scoop loop; M: protein marker; kDa: kilodalton; A280: absorption at 280 nm; V0: void volume; Vt: total volume.
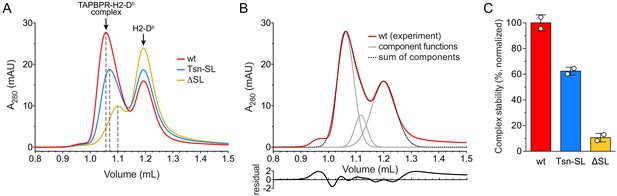
Complex formation between MHC I and TAPBPR variants.
(A) H2-Db (10 µM) loaded with a photo-cleavable peptide (RGPGRAFJ*TI, J* denotes photocleavable amino acid) was irradiated with UV light in the presence of TAPBPRwt (3 µM, red), TAPBPRTsn-SL (blue), or TAPBPRΔSL (yellow) and subsequently analyzed by SEC. The different elution volumes of the first main peak, marked by dashed lines, already hint at different complex stabilities. (B) Deconvolution of size-exclusion chromatogram from TAPBPRwt complex formation (experiment independent of the sample shown in (A)). The experimental chromatogram (red) was deconvoluted using three Gaussian functions (gray) that can be ascribed to the TAPBPR-H2-Db complex (1.06 mL), free TAPBPR (1.12 mL), and free H2-Db (1.20 mL). The sum of the three Gaussians is shown as dotted curve. The residual plot depicted beneath the main panel shows the difference between the experimental data and the sum. (C) Stability of complexes formed by TAPBPRwt, TAPBPRTsn-SL, and TAPBPRΔSL, respectively, as judged by the area of the complex peak obtained by deconvolution. Data represent mean ± SD (n = 2).
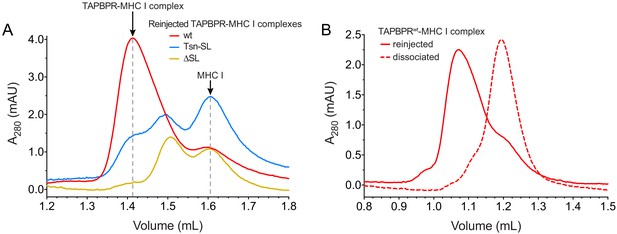
Stability of the MHC I complex formed by TAPBPRwt and the TAPBPR scoop-loop variants.
(A) Re-analysis by SEC (Superdex 200) of SEC-purified peptide-free TAPBPRwt-H2-Db (red trace), TAPBPRTsn-SL-H2-Db (blue trace), and TAPBPRΔSL-H2-Db (yellow trace) complex. Please note that dissociated peptide-deficient H2-Db is unstable and gets lost during the course of the experiment. (B) SEC-purified peptide-free TAPBPRwt-H2-Db complex was re-analyzed by SEC (Superdex 75) without (solid red trace) and with prior incubation with a 100-fold molar excess of high-affinity peptide (ASNENMETM) (dashed red trace). Please note that the extinction coefficient at 280 nm of the MHC I including β2m is 2.6-fold higher than the extinction coefficient of TAPBPR.
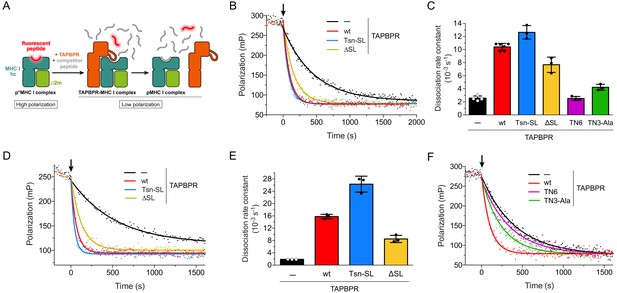
Peptide release from H2-Db and HLA-A*02:01 in peptide-rich environment.
(A) Schematic of peptide displacement assay. (B) Peptide dissociation kinetics from H2-Db (300 nM) loaded with fluorescently-labeled peptide (TQSC*NTQSI) was monitored in real time by fluorescence polarization. The arrow indicates the addition of a 1000-fold molar excess of unlabeled high-affinity competitor peptide (ASNENMETM) without TAPBPR (black trace) or in combination with 1 µM TAPBPR (red, blue, and yellow traces). (C) Average dissociation rate constants of uncatalyzed and catalyzed peptide dissociation from H2-Db, using the same conditions as in (B). Data represent mean ± SD (n = 2–6). (D) Representative fluorescence polarization traces of uncatalyzed and catalyzed peptide (FLPSDC*FPSF) dissociation from HLA-A*02:01 (300 nM). The arrow indicates the addition of a 1000-fold molar excess of unlabeled competitor peptide (FLPSDEEPYV, 300 µM) with and without TAPBPR (1 µM). (E) Average dissociation rate constants of uncatalyzed and catalyzed peptide dissociation from HLA-A*02:01, using the same experimental conditions as in (D). Data represent mean ± SD (n = 3). (F) Peptide dissociation from H2-Db (300 nM) after addition (arrow) of unlabeled competitor peptide (300 µM) without TAPBPR or in combination with the interface mutants TN6-TAPBPR and TN3-Ala-TAPBPR (1 µM each), respectively. A TAPBPRwt-catalyzed peptide release reaction is shown as reference. The average dissociation rate constants in the presence of TN6 (koff = 2.53 ± 0.30×10−3 s−1) and TN3-Ala (koff = 4.23 ± 0.45×10−3 s−1) are shown in panel (C). Abbreviations: β2m: β2-microglobulin; MHC I hc: MHC I heavy chain; pMHC I: peptide-MHC I; mP: milli-polarization units; wt: wildtype; Tsn: tapasin; SL: scoop loop.
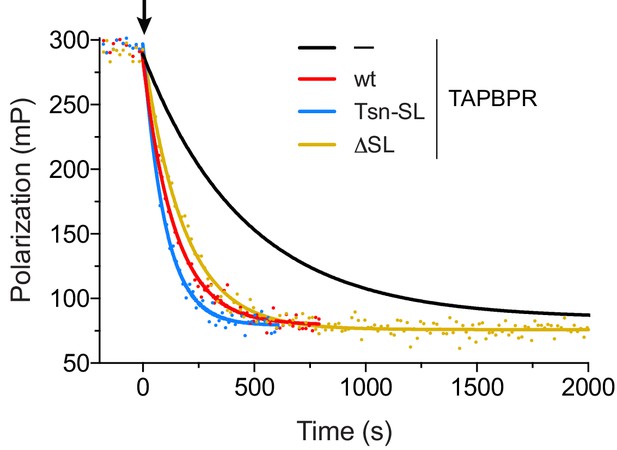
Catalyzed peptide displacement from H2-Db at low TAPBPR concentration.
Peptide dissociation kinetics from H2-Db (300 nM) loaded with fluorescently-labeled peptide (TQSC*NTQSI) was monitored in real time by fluorescence polarization. The arrow indicates the addition of a 1000-fold molar excess of unlabeled high-affinity competitor peptide (ASNENMETM) without TAPBPR (black fit, uncatalyzed reaction of Figure 3B) or in combination with 75 nM TAPBPR [red, blue, and yellow traces; koff (TAPBPRwt) = 7.05 × 10−3 s−1, koff (TAPBPRTsn-SL) = 10.12 × 10−3 s−1, koff (TAPBPRΔSL)=5.57 × 10−3 s−1].
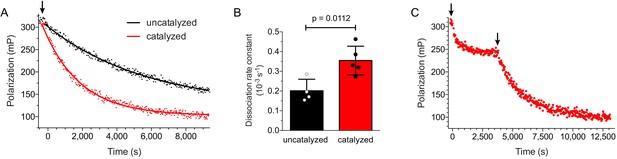
TAPBPRwt-catalyzed displacement of high-affinity peptide from H2-Db.
(A) Peptide dissociation kinetics (representative traces) from H2-Db (300 nM) loaded with a fluorescently-labeled high-affinity peptide (ASNC*NMETM) was monitored in real time by fluorescence polarization. The arrow indicates the addition of a 1000-fold molar excess of unlabeled high-affinity peptide (ASNENMETM, 300 µM) without TAPBPRwt (black trace) or in combination with TAPBPRwt (1 µM, red trace). (B) Average rate constants of uncatalyzed (0.20 ± 0.06 × 10−3 s−1) and catalyzed dissociation (0.35 ± 0.07 × 10−3 s−1) of high-affinity peptide from H2-Db, using the same conditions as in (A). Data represent mean ± SD (uncatalyzed: n = 4; catalyzed: n = 5). The p value was determined using an unpaired t test. (C) Representative trace (n = 3) of fluorescent high-affinity peptide displacement from H2-Db (300 nM), monitored by fluorescence polarization after addition of TAPBPRwt (1 µM, first arrow) and after subsequent addition of a 1000-fold molar excess of unlabeled high-affinity peptide (300 µM, second arrow). Abbreviation: mP: milli-polarization units.

The scoop loop acts as a selectivity filter during peptide editing.
(A) Schematic of two-step peptide exchange assay. (B) Peptide displacement from H2-Db (300 nM) loaded with fluorescently-labeled peptide (TQSC*NTQSI) was monitored by fluorescence polarization after addition of TAPBPR (1 µM, first arrow) and after subsequent addition of a 1000-fold molar excess of unlabeled high-affinity competitor peptide (ASNENMETM, 300 µM, second arrow). (C) Titration of peptide-loaded H2-Db (300 nM) with varying concentrations of TAPBPRwt (first arrow) and final addition of a 1000-fold molar excess of unlabeled high-affinity competitor peptide (300 µM, second arrow). (D) Peptide displacement from H2-Db (300 nM) loaded with fluorescently-labeled peptide monitored by fluorescence polarization after addition of 3 µM and 10 µM TAPBPRTsn-SL, respectively (first arrow), and after subsequent addition of a 1000-fold molar excess of unlabeled high-affinity competitor peptide (300 µM, second arrow). (E) Peptide displacement from H2-Db (300 nM) loaded with fluorescently-labeled peptide monitored by fluorescence polarization after addition of TAPBPRΔSL (3 µM, first arrow) and after subsequent addition of a 1000-fold molar excess of unlabeled high-affinity competitor peptide (300 µM, second arrow). Data shown in (B)-(E) are representative of three independent measurements.
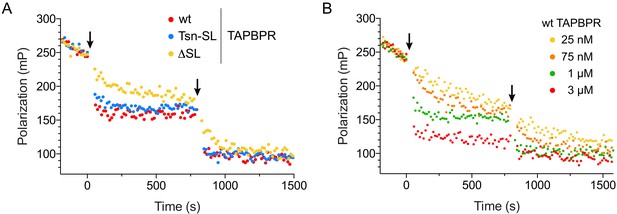
The scoop loop acts as a selectivity filter during peptide editing.
(A) Peptide displacement from HLA-A*02:01 (300 nM) loaded with fluorescently-labeled peptide (FLPSDC*FPSF) was monitored by fluorescence polarization after addition of TAPBPR (1 µM, first arrow) and after subsequent addition of a 1000-fold molar excess of unlabeled high-affinity competitor peptide (FLPSDEEPYV, 300 µM, second arrow). (B) Titration of peptide-loaded HLA-A*02:01 (300 nM) with varying concentrations of TAPBPRwt (first arrow) and final addition of a 1000-fold molar excess of unlabeled high-affinity competitor peptide (300 µM, second arrow). The data shown in (A) and (B) are representative of three independent measurements.

Proposed mechanistic functions of the scoop loop in catalyzed peptide proofreading.
MHC I molecules bound to low-affinity peptide are recognized by the peptide editor (TAPBPR) (step 1). The editor lowers the peptide affinity of the suboptimally-loaded MHC I and induces dissociation of the low- to medium-affinity peptide (step 2). The scoop loop, which inserts into the F-pocket region of the peptide-binding groove, crucially contributes to the stabilization of the empty MHC I. In the absence of suitable peptides, empty MHC I clients are thereby held in a stable state until they can be loaded with an optimal epitope, for example in the PLC. Re-binding of the low-affinity peptide (step 3) is impeded by the scoop loop, through direct competition and/or via allosteric means. Only high-affinity peptides are able to compete with the editor over key regions of the peptide-binding groove (step 4) to eventually displace the scoop loop and the editor from the MHC I (step 5). The displaced editor is now ready for a new round of peptide selection, and the stable pMHC I complex is licensed to travel via the Golgi apparatus to the cell surface.
Tables
Reagent type (species) or resource | Designation | Source or reference | Identifiers | Additional information |
---|---|---|---|---|
Gene (human) | TAPBPRwt | PMID:29025996 | lumenal domain | |
Gene (human) | TAPBPRΔSL | This study (Figure 1C, Materials and methods section) | lumenal domain | |
Gene (human) | TAPBPRTsn-SL | This study (Figure 1C, Materials and methods section) | lumenal domain | |
Gene (human) | TAPBPRTN3-Ala | PMID:19119025 | lumenal domain | |
Gene (human) | TAPBPRTN6 | PMID:19119025 | lumenal domain | |
Gene (human) | HLA-A*02:01 | This study (Materials and methods section) | ectodomain | |
Gene (human) | β2-microglobulin | PMID:29025996 | ||
Gene (mouse) | H2-Db | PMID:29025996 | ectodomain | |
Strain, strain background (Escherichia coli) | DH10Bac | Thermo Fisher Scientific | 10361012 | chemically competent |
Strain, strain background (Escherichia coli) | BL21(DE3) | Sigma-Aldrich | CMC0014 | chemically competent |
Recombinant DNA reagent | pET-22 | Novagen/ Merck Millipore | 69744 | vector for protein expression in E. coli |
Recombinant DNA reagent | pET-28 | Novagen/ Merck Millipore | 69864 | vector for protein expression in E. coli |
Recombinant DNA reagent | pFastBacI-gp67 | PMID:29025996 | transfer vector for Bac-to-Bac system | |
Cell line (Spodoptera frugiperda) | Sf9 | Thermo Fisher Scientific | 11496015 | |
Cell line (Spodoptera frugiperda) | Sf21 | Thermo Fisher Scientific | 11497013 | |
Peptide, recombinant protein | RGPGRAFJ*TI (photo-P18-I10) | PMID:26869717 | J* denotes photo-cleavable amino acid | |
Peptide, recombinant protein | ASNENMETM | IEDB: epitope ID 4602 | competitor peptide for H2-Db | |
Peptide, recombinant protein | FLPSDEEPYV | This study (Materials and methods section) | competitor peptide for HLA-A*02:01 | |
Peptide, recombinant protein | TQSC*NTQSI | This study (Materials and methods section) | C* denotes TAMRA-labeled Cys | |
Peptide, recombinant protein | FLPSDC*FPSF | This study (Materials and methods section) | C* denotes TAMRA-labeled Cys | |
Peptide, recombinant protein | ASNC*NMETM | This study (Materials and methods section) | C* denotes TAMRA-labeled Cys | |
Chemical compound, drug | TAMRA-5 maleimide | Thermo Fisher Scientific | T6027 | |
Chemical compound, drug | TAMRA-6 C2 maleimide | Thermo Fisher Scientific | 48180 | |
Chemical compound, drug | Fmoc-3-amino-3-(2-nitro)phenyl-propionic acid | Peptech | CAS #: 517905-93-0 | |
Software, algorithm | Prism 6 | GraphPad Software | ||
Software, algorithm | Fityk 1.3.1 | DOI: 10.1107/S0021889810030499 | ||
Other | Superdex 200 Increase 10/300 | GE Healthcare | 28990944 | SEC column |
Other | Superdex 200 Increase 3.2/300 | GE Healthcare | 28990946 | SEC column |
Other | Superdex 75 Increase 3.2/300 | GE Healthcare | 29148723 | SEC column |
Other | HiLoad Superdex 75 16/60 | GE Healthcare | 28989333 | SEC column |
Other | Fluorolog-3 | Horiba Jobin Yvon | spectro-fluorometer | |
Other | Äkta Purifier | GE Healthcare | protein purification | |
Other | Agilent 1200 | Agilent | analytical SEC | |
Other | Liberty Blue | CEM Corporation | peptide synthesizer | |
Other | X-tremeGENE HP | Sigma-Aldrich | 6366236001 | transfection reagent |