Proximal CA1 20–40 Hz power dynamics reflect trial-specific information processing supporting nonspatial sequence memory
Figures
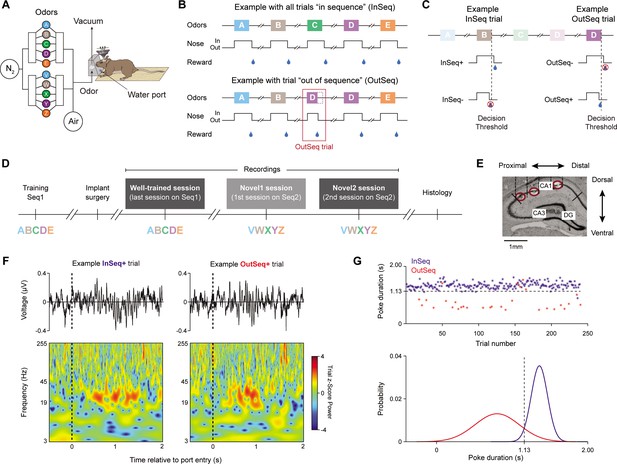
Local field potential (LFP) activity was recorded from hippocampal region CA1 as rats performed a nonspatial sequence memory task.
(A) Using an automated odor delivery system located at one end of a linear track, rats were presented with a series of five odors delivered in the same odor port. (B) In each session, the same sequence of five odors was presented multiple times. Approximately half the presentations included all items ‘in sequence’ (InSeq; e.g., ABCDE; top) and the other half including one ‘out of sequence’ item (OutSeq; e.g., ABDDE; bottom). For each odor presentation, rats were required to behaviorally indicate whether the presented odor was InSeq (by holding their nosepoke response until the auditory signal at 1.2 s) or OutSeq (by withdrawing their nose before the signal; <1.2 s) to receive a water reward. After completion of each sequence (correctly or incorrectly), animals were required to run to the opposite end of the linear track and return to the odor port before the next sequence could be presented. (C) For InSeq items, hold durations longer than the decision threshold were considered correct responses (InSeq+) by the hardware and shorter ones incorrect responses (InSeq-). For OutSeq items, hold durations shorter than the decision threshold were considered correct responses (OutSeq+) and longer ones incorrect responses (OutSeq-). (D) Data analysis focused on LFP activity recorded from three sessions: a session in which animals were tested on the sequence learned preoperatively in which they performed at a high level (ABCDE; well-trained session), as well as two successive sessions testing a novel sequence (VWXYZ; novel 1 and novel 2 sessions) in which they performed at low and moderate levels, respectively. (E). Sample coronal section (~4 mm posterior of bregma) showing the range of tetrode tip locations, which spanned much of the proximodistal axis of CA1 (three tip locations shown; red circles). For each animal, a set of four tetrodes equally distributed across the proximodistal axis (with comparable locations across animals) was used for local field potential activity analysis. (F) Representative LFP traces (top) and corresponding time-frequency plots (bottom) from example InSeq+ and OutSeq+ trials. (G) Nosepoke durations on InSeq and OutSeq trials from one representative animal (well-trained session; top). Normal distribution functions were fit separately for observed InSeq and OutSeq trials (bottom) and the intersection of the two distributions (1.13 s in this case) was used as the time cutoff to classify ‘hold’ and ‘withdraw’ responses during analysis (to account for anticipation errors occurring a few ms before the decision threshold). Panels from this figure were adapted from our previous work (Allen et al., 2016; Shahbaba et al., 2022).
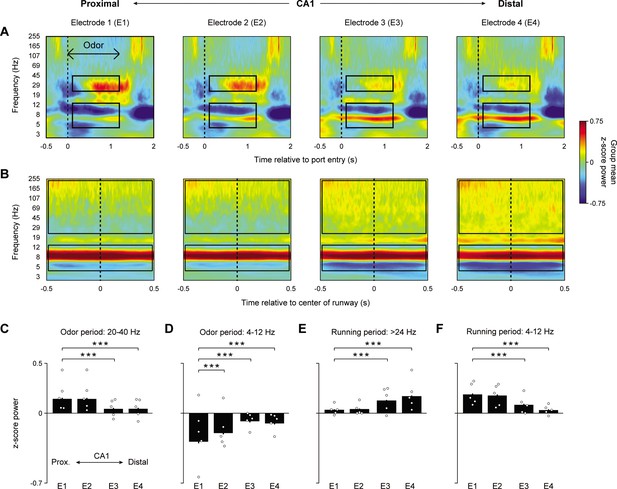
Odor sequence processing and running are associated with distinct CA1 oscillatory states, which vary across the proximodistal axis.
(A) Group peri-event spectrograms (n = 5) during odor sampling period in four electrode locations along the CA1 proximodistal axis (InSeq+ trials only; 0 ms = port entry). Black boxes indicate the time-frequency periods (defined a priori) examined in (C) and (D). (B) Group peri-event spectrograms from the same electrodes during the running period (0 ms = center of the runway). Black boxes refer to the time-frequency periods examined in (E) and (F). (C) Mean z-score 20–40 Hz power during 110–1200 ms period of odor presentation significantly increased toward proximal CA1 (significant effect of electrode: F3,2214 = 41.995, p<0.001). (D) Same as in (C), but for theta (4–12 Hz) showing increased power in the opposite direction (toward distal CA1; significant effect of electrode: F3,2214 = 60.952, p<0.001). (E) Mean z-score higher frequency power during running (>24 Hz to avoid theta’s first harmonic) increases toward distal CA1 (significant effect of electrode: F3,2768 = 181.34, p<0.001). (F) Same as in (E), but for theta showing increased power in the opposite direction (toward proximal CA1; significant effect of electrode: F3,2768 = 71.005, p<0.001). Bar plots display mean ± SEM, with individual subject means overlaid as circles. All data were extracted from the well-trained recording session (high-performance level). ***p<0.001 two-tailed t-tests (Bonferroni-corrected).
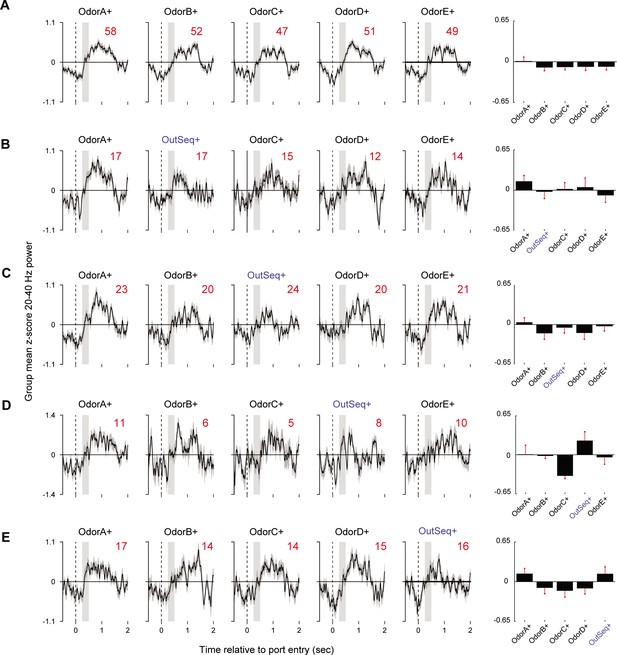
CA1 20–40 Hz power as a function of position in the sequence.
(A) Mean z-score 20–40 Hz power traces aligned relative to port entry as a function of position in the sequence (InSeq+ trials only; left). Mean power in the 250–500 ms period after port entry for each item (right). (B) Same as in (A) but for sequences in which the second item was OutSeq+. (C–E) Same as in (B), but for when the OutSeq+ item was presented in the positions 3–5, respectively. Bar plots display mean ± SEM.
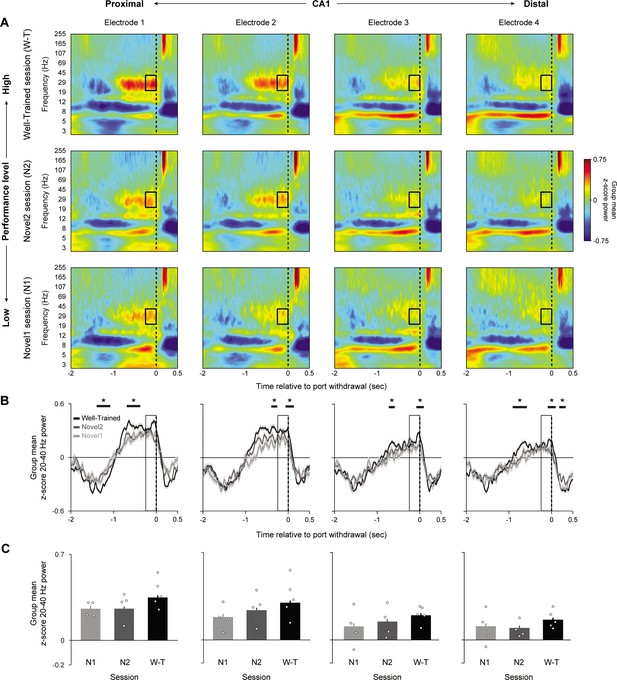
CA1 20–40 Hz power increases with knowledge of the sequence.
Group peri-event spectrograms across three sessions in which performance levels were low (novel 1, first session on novel sequence; n = 4 rats; bottom row), moderate (novel 2, second session on novel sequence; n = 4; middle row), and high (well-trained session; n = 5; top row). All plots are aligned to port withdrawal (0 ms = port withdrawal) and only include InSeq+ trials. Black box indicates the 250 ms window, defined a priori to capture the 20–40 Hz power plateau, which is analyzed in (C). (B) Mean z-score 20–40 Hz power traces (± SEM) from data in (A). Horizontal black bars indicate epochs with significant performance effect identified using a data-driven approach (cluster-based permutation testing [CBPT], n = 1000 permutations). (C) Mean z-score power in the 250 ms period prior to port withdrawal (indicated by boxes in A and B) in each of the three sessions, shown separately for each CA1 site. Power increased with session performance and toward the most proximal sites (significant effect of session: F2,4806 = 17.387, p<0.001; significant effect electrode: F3,4806 = 8.9081, p<0.001). Bar plots display mean ± SEM, with individual subject means overlaid as circles. *Significant epochs identified in CBPT analysis (p<0.05, corrected for multiple comparisons).
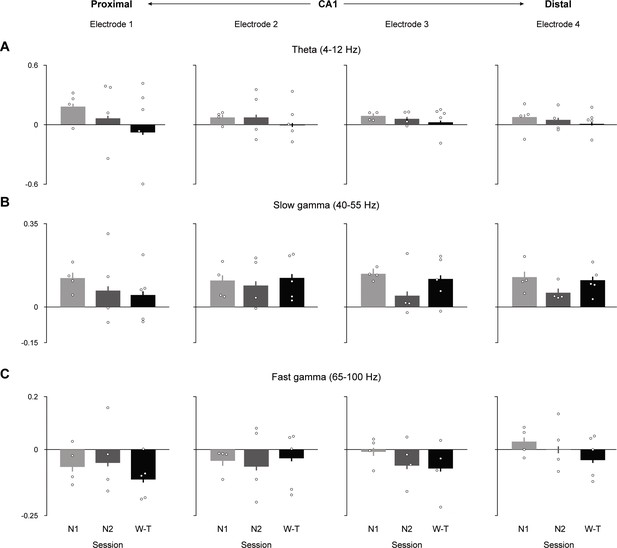
Frequency bands outside the 20–40 Hz range do not show increased power with session performance.
Mean z-score power in theta (A), slow gamma (B), and fast gamma (C) bands during the 250 ms period preceding port withdrawal in the three sessions, shown separately for each CA1 site (compare with 20–40 Hz results in Figure 3C). (A) Theta (4–12 Hz) power significantly decreases with session performance (F2,4806 = 7.6814, p<0.001) and toward distal CA1 (significant effect: F3,4806 = 3.5256, p=0.0143), with a significant interaction between performance and CA1 site (F6,4806 = 4.0794, p<0.001). (B) Slow gamma (40–55 Hz, to exclude overlap with 20–40 Hz band) power showed a significant effect of session performance (a nonlinear relationship; F2,4806 = 7.6814, p=0.0263). Power did not significantly vary along the CA1 axis, and the interaction between performance and electrode was nonsignificant (F3,4806 = 0.2596, p=0.8545 and F6,4806 = 1.9127, p=0.0750, respectively). (C) Fast gamma (60–100 Hz) power showed a general decrease with session performance, but the effect varied by electrode. Specifically, we found a significant effect of session performance (F2,4806 = 8.1471, p<0.001), CA1 site (F3,4806 = 6.4976, p<0.001), and a significant interaction between performance and CA1 site (F6,4806 = 3.9286, p<0.001). Bar plots display mean ± SEM, with individual subject means overlaid as circles.
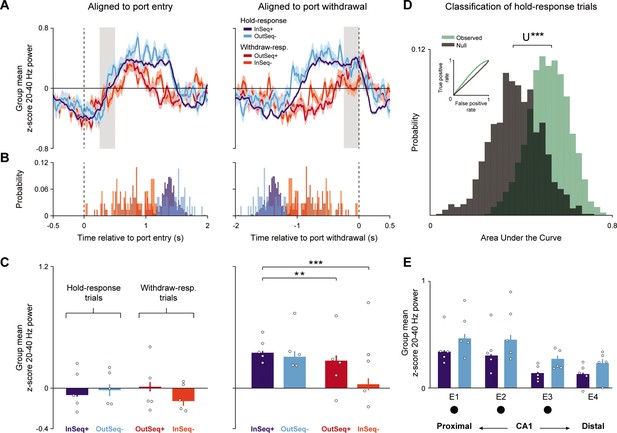
CA1 20–40 Hz power steady-state dynamics are attained on hold-response trials and their level predicts trial accuracy.
(A) Mean 20–40 Hz power traces (± SEM) for the four trial types, with activity aligned to port entry (left) and port withdrawal (right). Trial types are color coded by response type: hold responses are displayed in blue hues (InSeq+, OutSeq-) and withdraw responses in red hues (OutSeq+, InSeq-). Data are from most proximal CA1 electrode during the well-trained session (n = 5 rats). (B) Distribution of port withdrawal times (left; relative to port entry) and port entry times (right; relative to port withdrawal) for the trial data shown in (A). Note that poke durations are longer for hold-response trials (InSeq+, OutSeq-) and that 20–40 Hz power reached steady-state with longer trial durations. (C) Mean z-score 20–40 Hz power in the 250–500 ms period after port entry (left) and in the last 250 ms period prior to port withdrawal (right) for each trial type (epochs indicated by shaded regions in A). No differences were observed across conditions in the epoch relative to port entry (nonsignificant effect of trial type: F3,749 = 0.4804, p=0.6935). Power differed across conditions in the 250 ms period relative to port withdrawal (significant effect of trial type: F3,743 = 9.451, p<0.001), with significantly lower power during the OutSeq+ and InSeq- (withdraw) trials compared to InSeq+ (hold) trials (t743 = –2.829, p=0.0048 and t743 = –4.787, p<0.001, respectively). (D) Random forest classifier mean area under the curve (AUC) distributions for classifying between the hold-response trial types (class 1: InSeq+, class 2: OutSeq-) using observed and null data (mean observed and null AUCs of 0.5879 and 0.4772, respectively). Classifier yielded significantly higher performance when using the observed compared to the null data (U = 839538, p<0.001). 3-D classification feature vector consisted of 20–40 Hz power in electrodes 1–3 during the 650–1200 ms period after port entry (see Figure 4—figure supplement 1A for feature selection procedure). Inset shows mean observed and null receiver operating characteristics (ROCs) for the classification in (D). Classifier mean observed AUC, true positive rate [TPR], and false positive rate [FPR] were 0.5879, 0.5619, and 0.4444, respectively. (E) Mean z-score 20–40 Hz power in the 650–1200 ms period (identified through feature selection) in each of the four CA1 sites (filled black circles indicate electrodes identified by feature selection). Steady-state power was significantly higher in the OutSeq- compared to the InSeq+ condition (significant effect of trial type: F1,2406 = 32.687, p<0.001). Overall power was significantly higher toward proximal CA1 (significant effect of electrode: F3,2406 = 90.573, p<0.001). Bar plots display mean ± SEM, with individual subject means overlaid as circles. **p<0.01, ***p<0.001 two-tailed t-tests (Bonferroni-corrected). U***p<0.001 Mann–Whitney U test.
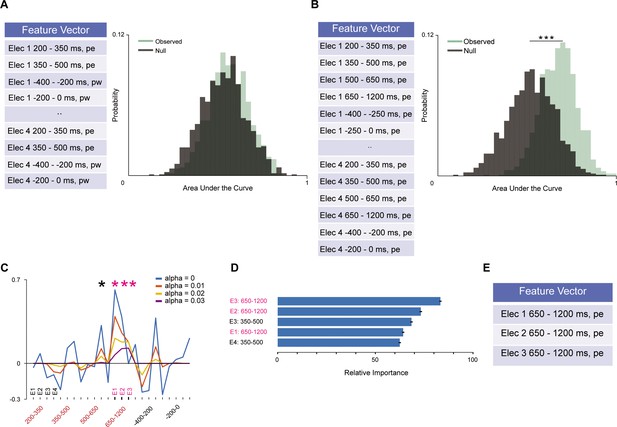
Feature definition and selection for random forest classifier analysis comparing 20–40 Hz power between hold-response trials (InSeq+ vs. OutSeq-).
Hypothesis-determined 16-D feature vector was defined as 20–40 Hz normalized power in two epochs relative to port entry (200–350, 350–500 ms) and two epochs relative to port withdrawal (−400 to –200, –200 to 0 ms) in each of the four CA1 sites (left). Random forest classifier performance was at chance (mean area under the curve [AUC], true positive rate [TPR], and false positive rate [FRP] of 0.4923, 0.4965, and 0.4740, respectively) using this feature vector (right). (B) Same as in (A), but using data-driven definition of a 24-D feature vector over entire power time course, including four epochs relative to port entry (200–350, 350–500, 500–650, 650–1200 ms) and two epochs relative to port withdrawal (−400 to –250, –250 to –0 ms) in each of the four CA1 sites (left). Classifier performed with a mean AUC, TPR, and FPR of 0.5727, 0.5532, and 0.4367, respectively (right). Performance was significantly higher for the observed compared to the null AUC values (U = 821138, p<0.001; indicated by ***). (C) Feature selection from the starting feature vector defined in (B) using lasso at increasing definitions of alpha (promoting sparsity). Features indicated by asterisks contained nonzero weights for all alpha definitions. (D) Gini impurity-based feature selection displaying the five features with highest relative importance out of the feature vector defined in (B). (E) Final feature vector for Figure 4 analysis involved the overlapping features identified by the lasso and Gini impurity approaches (indicated in magenta in C, D). pe, port entry; pw, port withdrawal.
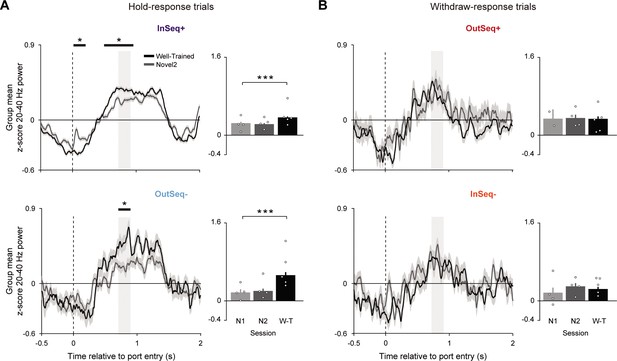
Hold-response trials, but not withdraw-response trials, show an increase in 20–40 Hz power with session performance.
(A) Mean z-score 20–40 Hz power traces (± SEM) for the hold-response trial types (top: InSeq+; bottom: OutSeq-) during the well-trained (n = 5 rats) and novel 2 (n = 4) sessions using the most proximal CA1 site. Significant epochs are indicated with black horizontal bands, performed separately for each trial type (cluster-based permutation testing [CBPT], p<0.05). Bar plots show power (mean ± SEM; individual subject means overlaid as circles) using a fixed epoch across all trial types (significant OutSeq- epoch, the overlap between hold response identified epochs; gray shaded region in line plots). Power during the InSeq+ and OutSeq- trial types significantly increased with session performance, with higher power during the well-trained compared to the novel 1 session (significant effects of performance: F2,1229 = 14.184, p<0.001 and F2,173 = 12.072, p<0.001, respectively). (B) Same as in (A), but for the withdraw-response trial types. Power during the OutSeq+ (top) and InSeq- (bottom) trial types did not significantly differ with performance (nonsignificant effects of performance: F2,128 = 0.3839, p=0.682 and F2,116 = 0.7962, p=0.4534). Individual animal values are overlaid for each bar plot. *Significant epochs identified in CBPT analysis (corrected for multiple comparisons). ***p<0.001 two-tailed t-tests (Bonferroni-corrected).
Additional files
-
Supplementary file 1
Trial counts for each session and animal.
Values in parentheses indicate trials included in the analyses (after artifact rejection; counts from most proximal electrode).
- https://cdn.elifesciences.org/articles/55528/elife-55528-supp1-v2.docx
-
Transparent reporting form
- https://cdn.elifesciences.org/articles/55528/elife-55528-transrepform1-v2.docx