Essential function of the alveolin network in the subpellicular microtubules and conoid assembly in Toxoplasma gondii
Figures
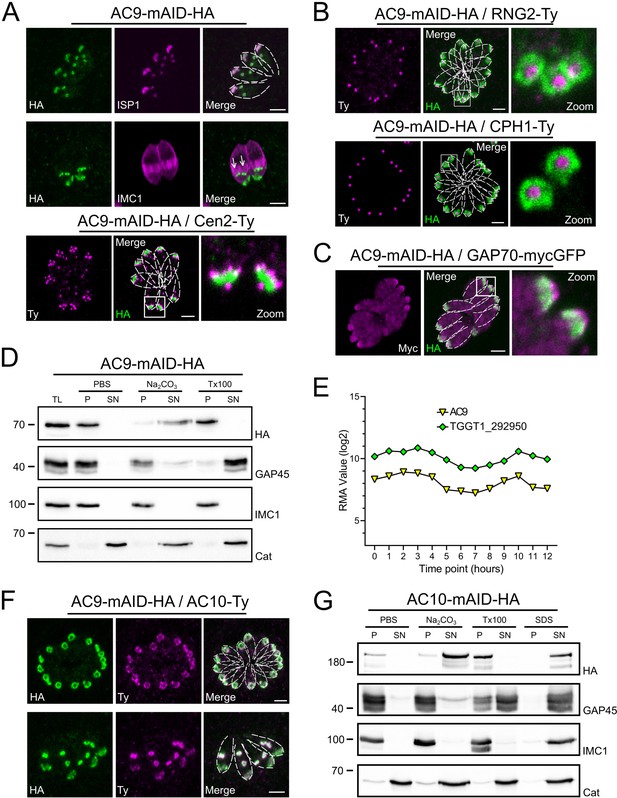
AC9 and the newly discovered AC10 are alveolin network proteins.
(A) AC9-mAID-HA localized to the apical cap of both mature and daughter cells (arrows). AC9 is confined to the apical IMC plate delimited by the Centrin2 marker. (B) AC9 surrounds the conoid (CPH1 marker) and the apical polar ring (RNG2 marker). (C) Colocalization with GAP70 revealed that AC9 is likely confined in the IMC/subpellicular network (SPN) and not associated with the PM. (D) AC9-mAID-HA is partially solubilized by sodium carbonate and precipitates in Triton X-100 insoluble fraction. (E) TGGT1_292950 was the top biotinylated hit. This hypothetical protein displayed an identical cyclic expression pattern compared to AC9. (F) TGGT1_292950 colocalized with AC9 in both daughter and mature parasite and was subsequently named AC10. (G) Similar to AC9, AC10-mAID-HA is partially solubilized only by sodium carbonate. Scale bars = 2 µm.
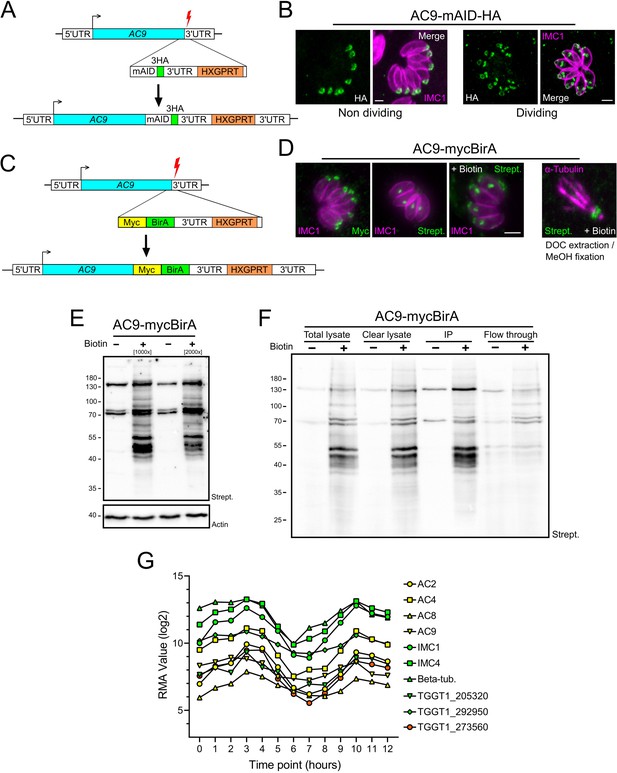
Proximity biotinylation by AC9.
(A) Schematic representation of the strategy employed to obtain the AC9-mAID-HA using CRISPR/Cas9 strategy. Red lightning represents Cas9 induced double-strand break. (B) AC9 localized in mature parasites and is recruited to daughter cells before IMC1. (C) Schematic representation of the strategy employed to obtain the AC9-mycBirA strain using CRISPR/Cas9 strategy. (D) AC9-mycBirA fusion was correctly localized and upon addition of biotin, enrichment of biotinylated proteins was detected by streptavidin-based fluorophore in both intracellular and extracellular DOC extracted parasites. (E) Upon addition of biotin, several biotinylated proteins were detected by western blot. (F) Parasites were cultivated for 24 hr in standard medium supplemented with biotin, harvested, solubilized in cytoskeletal buffer and streptavidin magnetic beads were used to precipitate (IP) biotinylated proteins that were sent for mass spectrometry analysis. (G) Similar expression profiles of identified proteins by mass spectrometry. Scale bars = 2 µm.
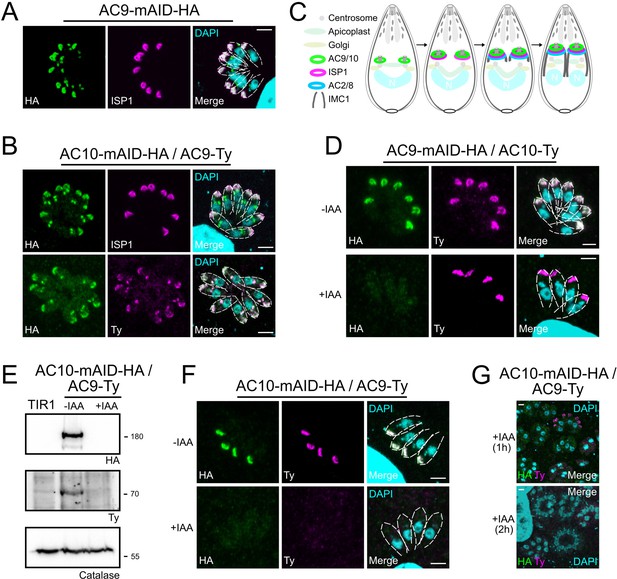
AC9 and AC10 are among the earliest markers of parasite division.
(A and B) AC9 and AC10 are both recruited very early during budding of daughter cells and before ISP1. (C) Schematic representation of the events during endodyogeny highlighting the sequential insertion of the different components of the IMC/SPN/cytoskeleton. (D) AC10 localization was not impacted upon depletion of AC9. (E and F) Depletion of AC10 caused degradation of AC9. (G) Shorter auxin treatments were sufficient to remove AC9 from the apical cap suggesting that AC9 and AC10 form a complex. Scale bars = 2 µm.
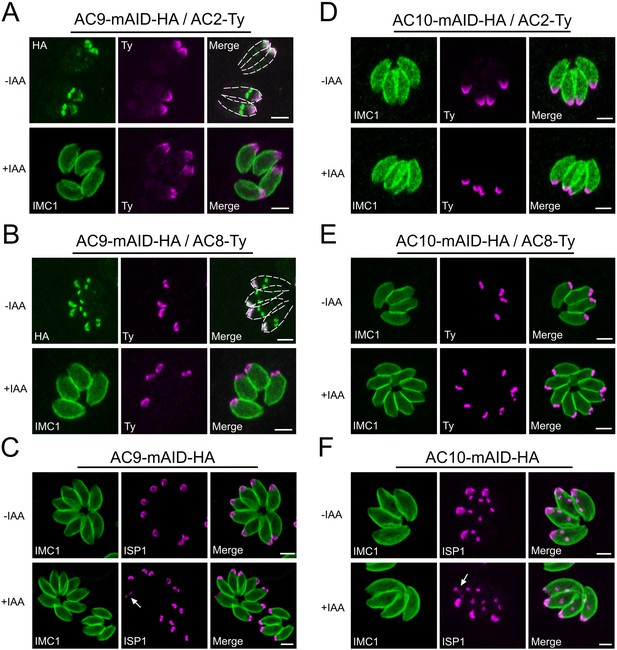
No alterations of AC2, AC8 and ISP1 upon depletion of AC9 and AC10.
(A and B) AC2 and AC8 are inserted very late during division in the daughter cells after AC9. None of AC2 and AC8 are lost in absence of AC9. (C) Apical cap protein ISP1 was not impacted upon depletion of AC9; however the apical region of one or few parasites per vacuole appeared enlarged (arrow). (D and E) AC2 and AC8 were not impacted by depletion of AC10. (F) ISP1 localization is not impacted upon degradation of AC10; similarly to AC9, some parasites presented an enlarged apical opening (arrow). Scale bars = 2 µm.
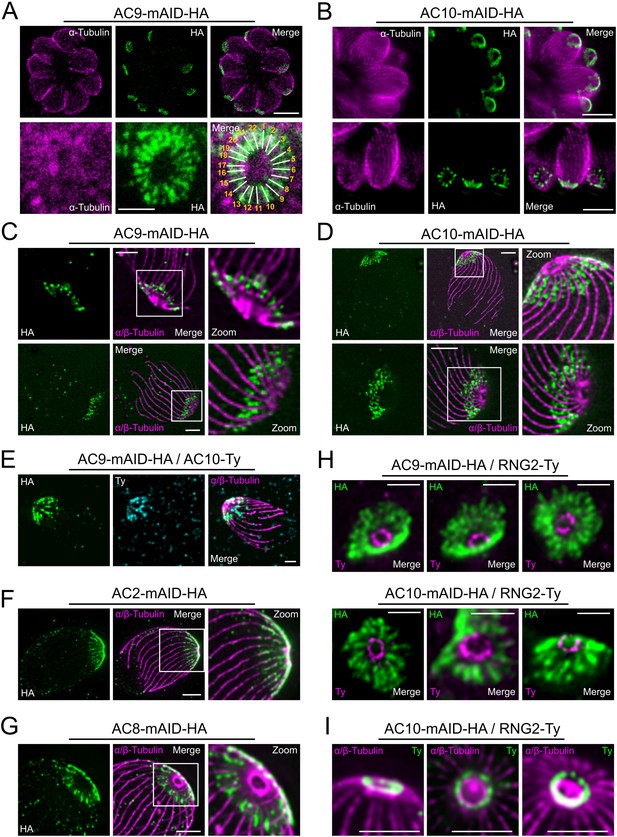
Super resolution microscopy revealed a peculiar localization of ACs proteins.
(A and B) Stimulated emission-depletion super-resolution microscopy (STED) revealed that AC9 and AC10 are arranged in periodical rows reminiscent of subpellicular microtubules (SPMTs) arrangement. (C and D) Ultrastructure Expansion microscopy (U-ExM) confirmed that AC9 and AC10 are arranged in regular rows and localized in between the SPMTs. (E) AC9 and AC10 colocalized at the apical cap level. (F) AC2 localized on SPMTs by U-ExM at the apical cap up to the APR. (G) As AC9 and AC10, AC8 localized in between SPMTs. (H and I) Colocalization with RNG2 revealed that both AC9 and AC10 surrounds the APR without making significant contacts with it. Surprisingly, the RNG2 signal did not appear to be homogeneous at the APR but rather forming discrete dots by STED and U-ExM. Scale bars = 2 µm, except for 3A (bottom panel) and 3H = 0.5 µm.
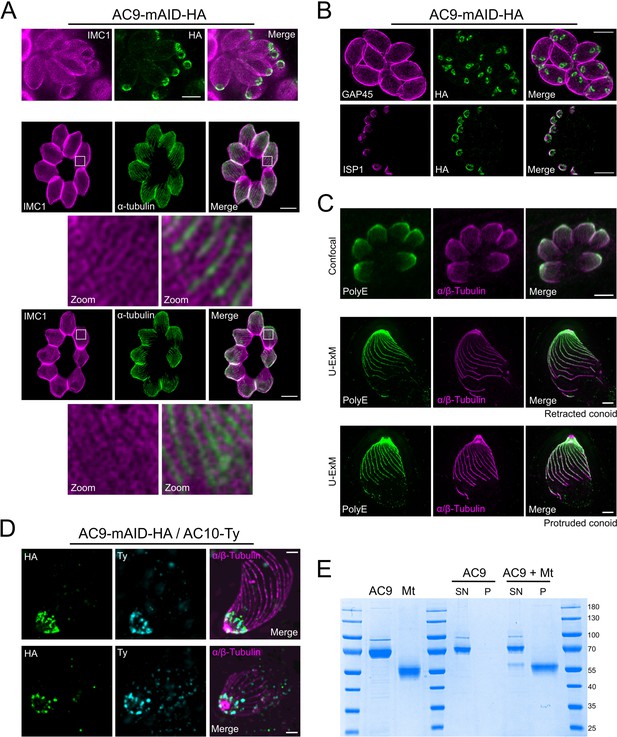
STED and U-ExM revealed unprecedented localization of alveolin network proteins and tubulin modification.
(A) The alveolin network (stained by IMC1) also appears to be arranged in a periodic pattern (PAF/GLU fixation). Colocalization with tubulin by STED microscopy highlights the localization of IMC1 in between SPMTs (MetOH fixation). (B) Colocalization by STED with GAP45 and ISP1 confirmed that AC9 is present in the alveolin network side of the IMC and not in the pellicle. (C) Confocal versus U-ExM techniques showing heavy poly-glutamylation of the SPMTs of T. gondii. (D) Additional images showing the colocalization of AC9 and AC10 at the apical cap by U-ExM. (E) Recombinant AC9 was not able to bind microtubules in in vitro assay. MT: microtubule; SN: supernatant; P: pellet. Scale bars = 2 µm.
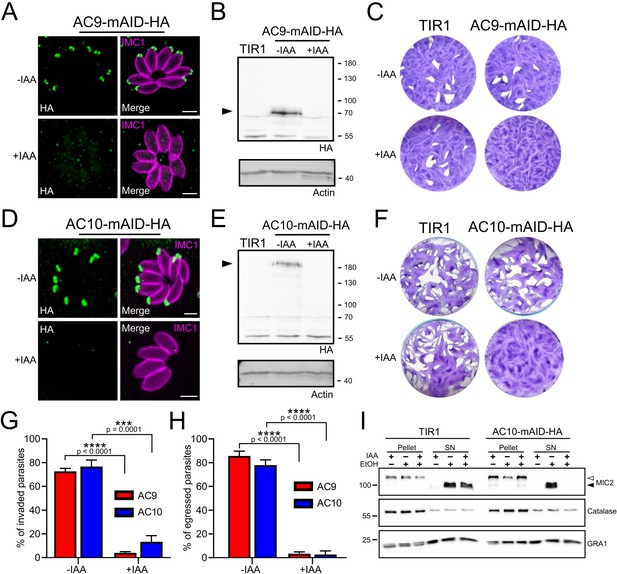
AC9 and AC10 are essential proteins for microneme secretion, invasion and egress.
(A), (B) and (C) AC9-mAID-HA was tightly regulated by addition of IAA and AC9 depleted parasites failed to form lysis plaques after 7 days. TIR1 represents the parental strain and was used as a control. (D), (E) and (F) AC10-mAID-HA was rapidly degraded upon addition of IAA and its depletion resulted in no visible plaque formation after 7 days. (G and H) Both invasion and egress were severely impaired in absence of AC9 and AC10. Data represented are mean values ± standard deviation (SD) from three independent biological experiments. A parametric paired t-test was used to assess significance; the two-tailed p-values are written on the graphs. (I) Microneme secretion was completely abolished in AC10 depleted parasites stimulated by ethanol. Pellets and supernatants (SN) were analyzed using α-MIC2 antibodies for secretion (white arrow: full length MIC2; black arrow: secreted MIC2), α-catalase (CAT) to assess parasites lysis and α-dense granule 1 (GRA1) for constitutive secretion. Scale bars = 2 µm.
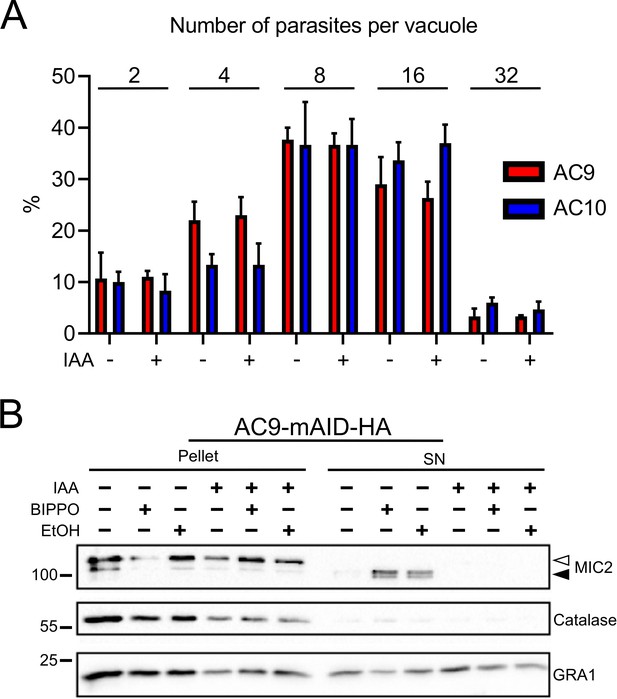
AC9 and AC10 depletion did not cause any defect in parasite replication.
(A) Intracellular development was not impacted upon depletion of AC9 and AC10. (B) AC9 depletion resulted in a complete block of microneme secretion. Extracellular parasites were stimulated with ethanol and BIPPO. Data represented are mean values ± standard deviation (SD) from three independent biological experiments.
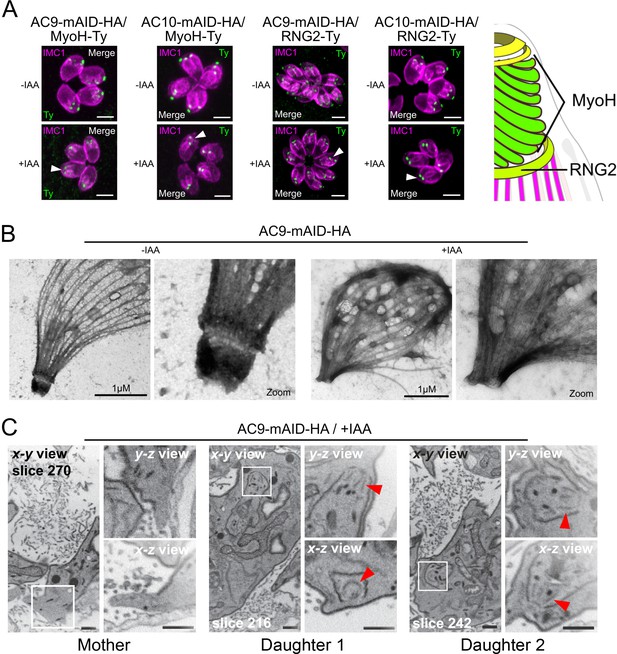
AC9 and AC10 depleted mother parasites lost the APR and the conoid.
(A) Both conoid-associated MyoH and apical polar ring RNG2 staining were completely lost in mature parasites while still present in forming daughter cell (arrowhead) in absence of AC9 and AC10. (B) AC9 depletion caused loss of the conoid and the apical polar ring in agreement with IFA analysis using RNG2 marker. Parasite were grown for 48 hr -/+ IAA, syringe lysed and processed as described in Materials and methods. (C) FIB-SEM confirmed that loss of AC9 impacted the conoid and APR exclusively in the mother cell. Red arrows highlight conoids. Scale bars = 2 µm.
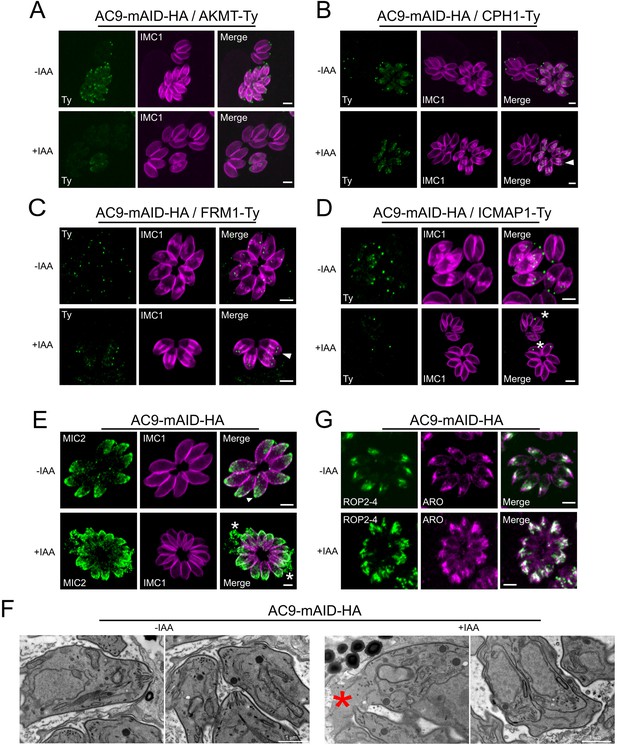
Destabilization of apical complex components upon depletion of AC9.
(A), (B) and (C) Apical complex methyltransferase (AKMT), conoid protein hub 1 (CPH1) and apical actin nucleator FRM1 were all lost from the mature tachyzoites but still present in daughter cells (arrowhead). (D) ICMAP1 was also lost from the apical tip; however in some parasites per vacuole, ICMAP staining was still present ‘floating’ in the parasite cytoplasm (asterisk). (E) Conoidal micronemes (arrowhead) were absent in parasites depleted of AC9 and some vacuoles showed leaking of micronemes in the vacuolar space (asterisk). (F) By electron microscopy, the conoid was completely lost from mother tachyzoites in AC9 depleted parasites while still present in the growing daughter cells. As showed by IFA, releasing of cytoplasmic material in the vacuolar space was also observed (red asterisk). (G) Rhoptries were mildly affected by the loss of AC9; they remained attached to the apical tip but appeared to be more dispersed and not compacted together compared to untreated parasites. Scale bars = 2 µm.
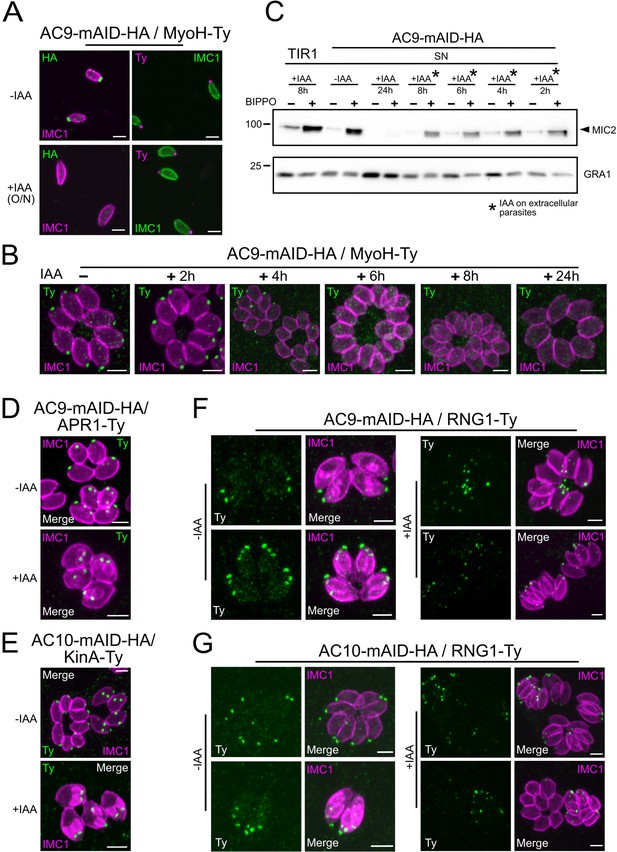
Loss of the APR and conoid occur during the last stages of division.
(A) IAA overnight treatment of extracellular parasites did not result in MyoH loss from the apical tip of non-dividing mature cell. (B) MyoH is lost only during parasite division after more than 2 hr treatment (i.e. the time to complete at least one division cycle). (C) IAA was added at different time point on extracellular parasites showing that microneme secretion is not affected in such conditions. (D and E) APR1 and KinA are lost in mature parasites upon deletion of AC9 and AC10, respectively. (F and G) RNG1 is incorporated very late in daughter cell, way after the alveolin network scaffold stained with IMC1. Upon depletion of both AC9 and AC10, RNG1 failed to be properly anchored at the APR in most parasites. Scale bars = 2 µm.
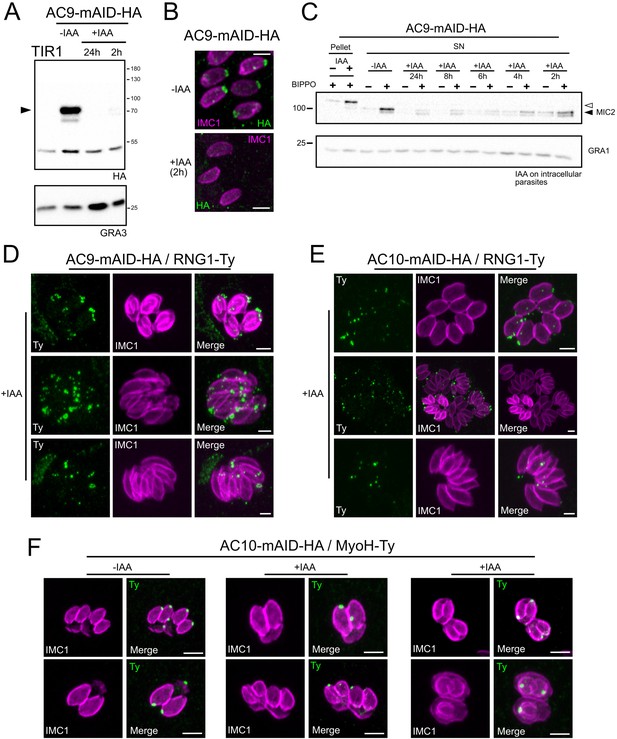
Loss of the APR and conoid just before the emergence of daughter cells.
(A) AC9-mAID-HA was rapidly degraded by western blot and (B) IFA analysis. (C) Intracellular treated parasites lost the ability to secrete microneme in a time-dependent manner. (D and E) Additional images showing RNG1 failing to be properly anchored at the APR in most parasites depleted of AC9 and AC10. (F) IFA were fixed after approximately 15–16 hr of IAA treatment resulting in parasite having completed around 1 or 2 division cycles. In such conditions, MyoH staining could be observed in the cytosol or degraded in the last steps of parasite division/daughter cell emergence. Scale bars = 2 µm.
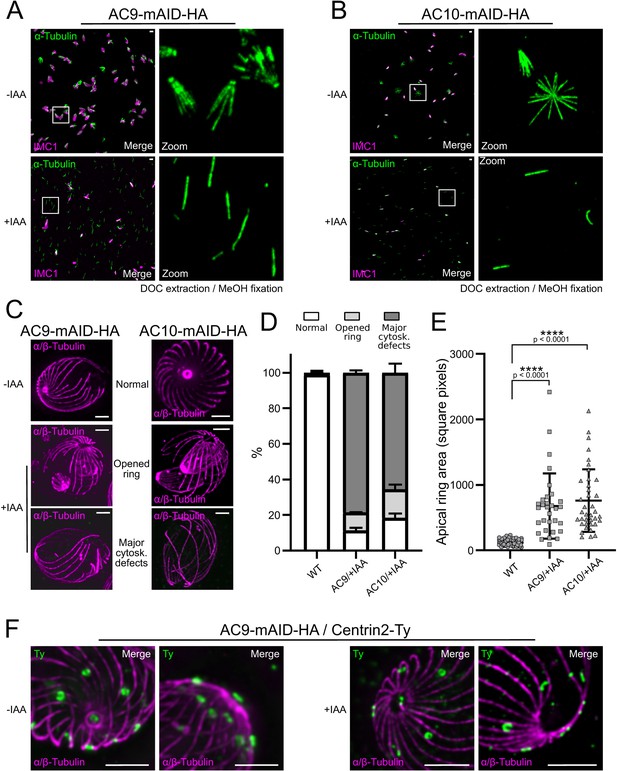
Depletion of AC9 and AC10 caused major cytoskeletal defects.
(A and B) Freshly egressed parasites were extracted with DOC on gelatin coverslips. In AC9 and AC10 depleted parasites the microtubular cytoskeleton collapsed and only single microtubules can be visualized. (C) U-ExM confirmed that parasites depleted of AC9 and AC10 presented an enlarged apical opening while the microtubular cytoskeleton presented major structural defects. (D) Quantification of the phenotypical defects displayed in panel (C). Two independent preparations show statistically significant differences between the WT group and the AC9/+IAA and AC10/+IAA groups for each of the three categories (normal, open ring, major cytoskeletal defects). Chi2 tests, standardized deviation from expected values and Fisher’s test were performed to ensure the statistical differences between each categories of the WT group versus AC9/+IAA or AC10/+IAA groups. (Figure 7—source data 1) (E) Measure of the area of the apical polar ring show statistically significant differences between the three groups. A non-parametric test (ANOVA) was performed to ensure statistical significance. Tukey all pairs comparison test was used to assess significance between groups (p-values are shown on the figure) (Figure 7—source data 2) (F) AC9 depletion caused the loss of pre-conoidal rings while the peripheral annuli were not compromised. Scale bars = 2 µm.
-
Figure 7—source data 1
Statistical analysis for the Figure 7D.
- https://cdn.elifesciences.org/articles/56635/elife-56635-fig7-data1-v2.xlsx
-
Figure 7—source data 2
Statistical analysis for the Figure 7E.
- https://cdn.elifesciences.org/articles/56635/elife-56635-fig7-data2-v2.xlsx
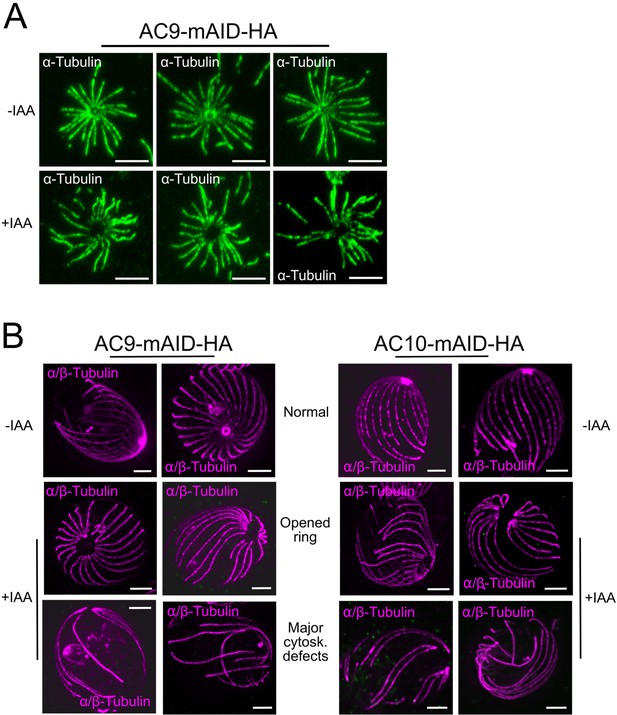
Structural defects upon depletion of AC9 and AC10.
(A) In rare cases, shorter treatment with DOC (deoxycholate) did not result in cytoskeleton collapsing but the absence of the apical polar ring and the conoid can be easily observed in IAA treated parasites. (B) Additional images of cytoskeletal structural defects in absence of AC9 and AC10. Scale bars = 2 µm.
Videos
FIB-SEM analysis highlighted the specific loss of conoid in the mother cell but not in the growing daughter cells.
Additional files
-
Supplementary file 1
Top hits of AC9 BioID identified by mass spec with accession numbers, transcriptomic data, number of peptides detected and CRISPR-Cas9 essentiality score.
- https://cdn.elifesciences.org/articles/56635/elife-56635-supp1-v2.xlsx
-
Supplementary file 2
Mass spectrometry data of AC9-BirA experiment.
- https://cdn.elifesciences.org/articles/56635/elife-56635-supp2-v2.xlsx
-
Supplementary file 3
Key resources table.
- https://cdn.elifesciences.org/articles/56635/elife-56635-supp3-v2.docx
-
Supplementary file 4
List of primers used in this study.
- https://cdn.elifesciences.org/articles/56635/elife-56635-supp4-v2.xlsx
-
Transparent reporting form
- https://cdn.elifesciences.org/articles/56635/elife-56635-transrepform-v2.pdf