α-synuclein strains that cause distinct pathologies differentially inhibit proteasome
Figures
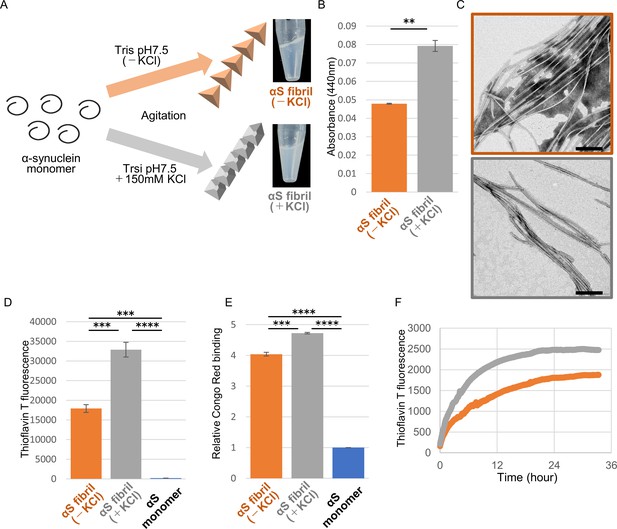
Preparation and characterization of two α-synuclein strains.
(A) Schematic representation of two α-synuclein (αS) strains (left) and the resulted two α-synuclein assemblies (right). (B) Turbidity of these assemblies. Analysis was performed using student t test. (mean ± S.E.M; n = 3) Formation of aggregates rather than soluble oligomer is shown in Figure 1—figure supplement 1. (C) Transfer electron microscopy (TEM) images of these assemblies. Scale bar, 200 nm. (D) Thioflavin T fluorescence of these assemblies and αS monomer. Analysis was performed using one-way ANOVA and Tukey post hoc test. (mean ± S.E.M; n = 3) (E) Congo red bindings of these strains and αS monomer. (mean ± S.E.M; n = 3) (F) In vitro seeding activity of these strains. α-synuclein monomers were incubated with α-synuclein fibril (-) (orange) or α-synuclein fibril (+) (gray). Kinetics of Thioflavin T fluorescence were shown. Analysis was performed using one-way ANOVA and Tukey post hoc test. **p<0.01, ***p<0.001, ****p<0.0001.
-
Figure 1—source data 1
Quantification for graph in Figure 1B,D and E.
- https://cdn.elifesciences.org/articles/56825/elife-56825-fig1-data1-v2.xlsx
-
Figure 1—source data 2
Three independent data in Figure 1F.
- https://cdn.elifesciences.org/articles/56825/elife-56825-fig1-data2-v2.xlsx
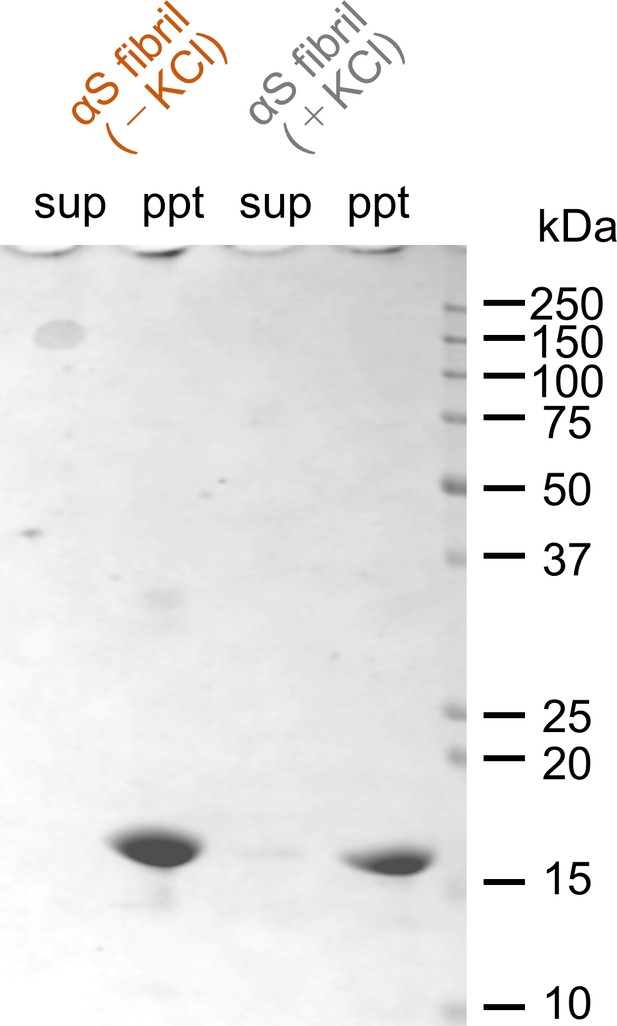
Formation of α-synuclein aggregates.
α-synuclein monomers are incubated for 7 days and then centrifuged at 10,000 x g for 10 min to separate soluble oligomers and insoluble aggregates. CBB staining of soluble fractions (sup) and insoluble fractions (ppt) are shown. Soluble oligomers are hardly detected in both α-synuclein strains.
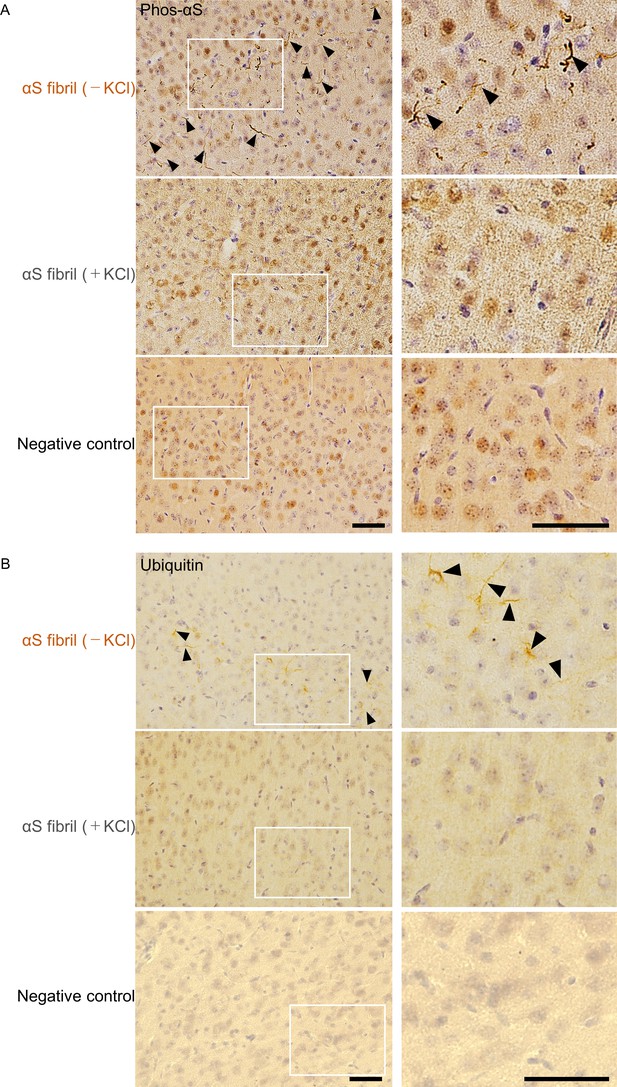
Comparison of pathologies in WT mouse brains by inoculation of two α-synuclein strains.
(A) Distribution of phosphorylated α-synuclein pathology in mouse brain. α-synuclein fibrils (-) or α-synuclein fibrils (+) were injected into the striatum of WT mouse brain and stained with phosphorylated α-synuclein antibody 1 month after injection. Regions surrounded by white rectangles in left panels are magnified and shown in right panels. Typical pathological α-synuclein deposits are indicated by the arrowheads. Sections were counterstained with hematoxylin. Representative images of cortex of mice injected with α-synuclein fibrils (-) (upper), α-synuclein fibrils (+) (middle) or not injected mice as negative controls (lower) are shown. Representative images of corpus callosum and striatum are shown in Figure 2—figure supplement 1. Representative images of cortex of other two mice injected with α-synuclein fibrils are also shown in Figure 2—figure supplement 2. (B) Distribution of ubiquitin pathology in mouse brain. α-synuclein fibrils (-) or α-synuclein fibrils (+) were injected into the striatum of WT mouse brain and stained with ubiquitin antibody 1 month after injection. Representative images of cortex of mice injected with α-synuclein fibrils (-) (upper), α-synuclein fibrils (+) (middle) or not injected mice as negative controls (lower) are shown. Regions surrounded by white rectangles in left panels are magnified and shown in right panels. Typical pathological ubiquitin positive deposits are indicated by the arrowheads. Sections were counterstained with hematoxylin. Scale bars, 50 µm.
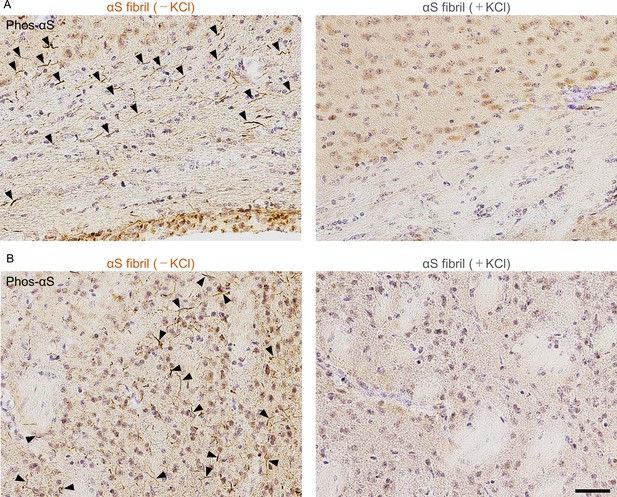
Comparison of pathologies in WT mouse brains by inoculation of two α-synuclein strains.
Distribution of phosphorylated α-synuclein pathology in mouse brain. α-synuclein fibrils (-) or α-synuclein fibrils (+) were injected into the striatum of WT mouse brain and stained with phosphorylated α-synuclein antibodies 1 month after injection. Representative images of corpus callosum (A) and striatum (B) are shown. Typical pathological α-synuclein deposits are indicated by the arrowheads. Sections were counterstained with hematoxylin. Scale bar, 50 µm.
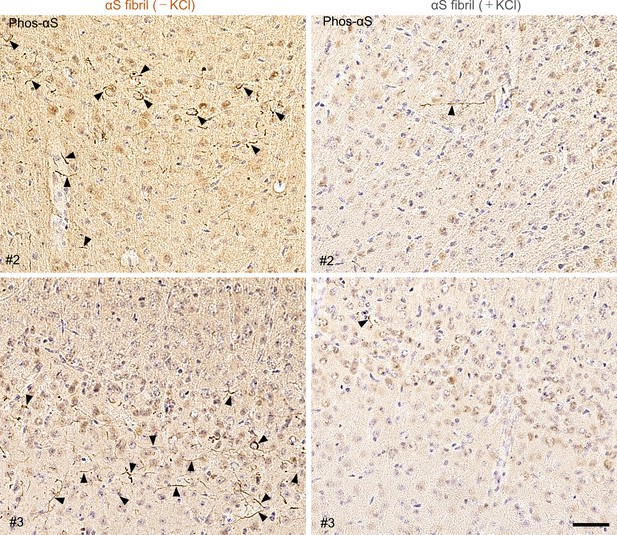
Images of cortex of other two mice injected with the α-synuclein fibrils.
Distribution of phosphorylated α-synuclein pathology in mouse brain. α-synuclein fibrils (-) or α-synuclein fibrils (+) were injected into three mice each and stained with phosphorylated α-synuclein antibodies 1 month after injection. Representative images of cortex of other two mice (#2 and #3), not shown in Figure 2, are shown. Typical pathological α-synuclein deposits are indicated by the arrowheads. Sections were counterstained with hematoxylin. Scale bar, 50 µm.
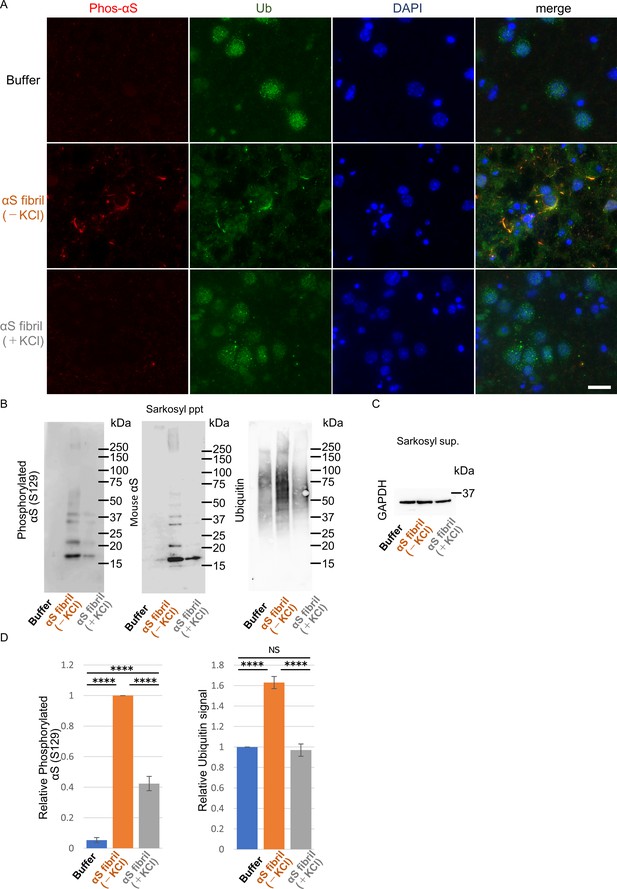
Seeding activities α-synuclein strains in primary mouse cortical neurons.
The two α-synuclein fibrils were transduced into primary mouse cortical cells. 14 days after fibril transduction, the accumulation of abnormal phosphorylated α-synuclein and ubiquitinated aggregates are detected by immunofluorescence microscopy and western blotting. (A) Phosphorylated α-synuclein (Phos-αS), ubiquitin (Ub) and nuclei (DAPI) were stained. Scale bar, 20 µm. Images of cells double stained with antibody against phosphorylated α-synuclein and neuronal or astrocyte markers are shown in Figure 3—figure supplement 1. Uptake of these α-synuclein fibrils into cells are shown in Figure 3—figure supplement 2. (B) Detection of sarkosyl insoluble phosphorylated α-synuclein (left), endogenous mouse α-synuclein (center) and ubiquitinated proteins (right) by western blotting. (C) Detection of sarkosyl soluble GAPDH as a loading control. (D) The quantification data of sarkosyl insoluble phosphorylated α-synuclein (left) and ubiquitinated proteins (right) shown in (B) (mean ± S.E.M; n = 3). Analysis was performed using one-way ANOVA and Tukey post hoc test. ****p<0.0001.
-
Figure 3—source data 1
Quantification for graph in Figure 3D and Figure 3—figure supplement 2C.
- https://cdn.elifesciences.org/articles/56825/elife-56825-fig3-data1-v2.xlsx
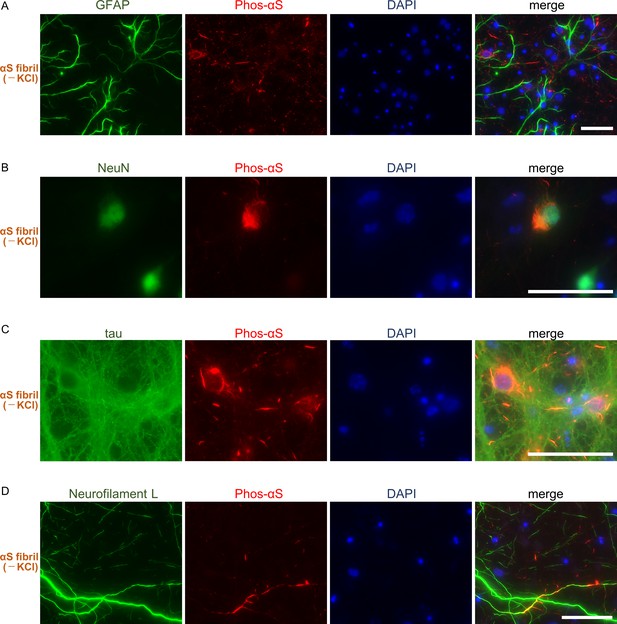
Double stained Images of phosphorylated α-synuclein and neuronal or astrocyte markers.
α-synuclein fibrils (-), which induced the accumulation of phosphorylated α-synuclein, were transduced into primary mouse cortical cells. 14 days after fibril transduction, phosphorylated α-synuclein and neuronal or astrocyte markers were doubly stained. (A) Phosphorylated α-synuclein (Phos-αS), GFAP (an astrocyte marker) and nuclei (DAPI) were stained. (B) Phosphorylated α-synuclein (Phos-αS), NeuN (a mature neuronal cell marker) and nuclei (DAPI) were stained. (C) Phosphorylated α-synuclein (Phos-αS), tau (a mature neuronal cell marker) and nuclei (DAPI) were stained. (D) Phosphorylated α-synuclein (Phos-αS), Neurofilament-L (a mature neuronal cell marker) and nuclei (DAPI) were stained. Scale bars, 50 µm.
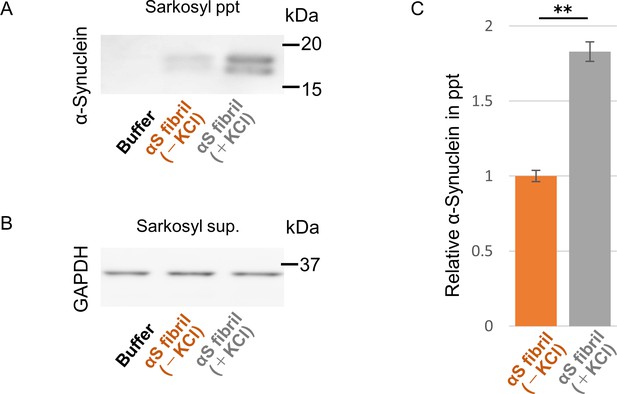
Uptake of the α-synuclein fibrils into the cells.
The two α-synuclein fibrils were transduced into primary mouse cortical cells. A day after fibril transduction, cells were washed and treated with trypsin to digest extracellular α-synuclein fibrils. Cells were collected and lysed in the presence of sarkosyl. The cell lysates were centrifuged at 135,000 × g for 20 min, then the supernatant (sup) was collected as sarkosyl-soluble fraction, and the protein concentration was determined by Bradford assay. The precipitate (ppt) was solubilized in SDS-sample buffer and used sarkosyl-insoluble fractions. (A) α-synuclein detected in sarkosyl- insoluble fractions were regarded as the α-synuclein fibrils taken up by the cells. (B) GAPDH detected in sarkosyl- soluble fractions as loading controls. (C) The quantification data of sarkosyl insoluble α-synuclein shown in (A) (mean ± S.E.M; n = 3). Analysis was performed using student t test. **p<0.01.
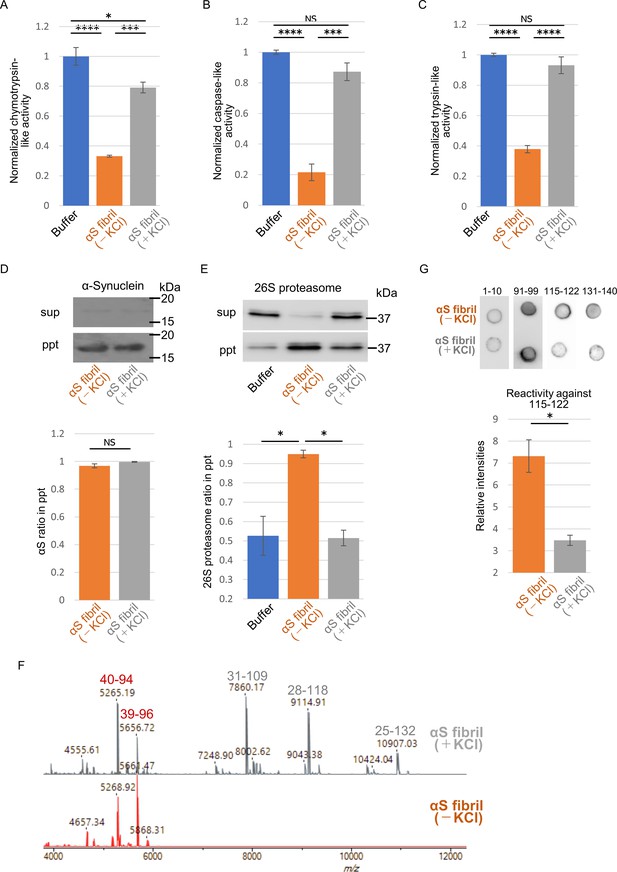
Different interaction of α-synuclein strains with 26S proteasome.
(A–C) Effects of the α-synuclein strains on the 26S proteasome activity. 26S proteasome was purified from budding yeast as shown in Figure 4—figure supplement 1. 26S proteasome activities in the presence of the two α-synuclein fibrils were measured. Chymotrypsin like activity was measured by LLVY-MCA hydrolysis(A). Caspase like activity was measured by LLE-MCA hydrolysis (B). Trypsin like activity was measured by LRR-MCA hydrolysis (mean ± S.E.M; n = 3). (D and E) Co-precipitation of 26S proteasome with the α-synuclein strains. 26S proteasome was mixed with the two α-synuclein fibrils and centrifuged. Resulted supernatants (sup) and precipitates (ppt) were analyzed by western blotting against α-synuclein (D, upper) and Rpn11-HA (E, upper). Quantification of the α-synuclein (D, lower) and Rpn11-HA (E, lower) in ppt fractions were shown. (mean ± S.E.M; n = 3) (F) The core regions of the α-synuclein strains. These α-synuclein fibrils were mildly digested by proteinase K and centrifuged. The resulted pellet fractions were denatured by guanidine and analyzed by MALDI-TOF-MS. Peptide peaks identified by mass analysis and the predicted peptide regions corresponding to each peak are shown. (G) Dot blot analysis of the α-synuclein strains. These α-synuclein fibrils were spotted on nitrocellulose membranes and detected by various antibodies against α-synuclein (upper). Reactivity against the antibody raised against 115–122 region of α-synuclein was quantified (mean ± S.E.M; n = 3) (lower). Analysis was performed using one-way ANOVA and Tukey post hoc test. *p<0.05, ***p<0.001, ****p<0.0001 (A, B, C and E). Analysis was performed using student t test. *p<0.05 (D and G).
-
Figure 4—source data 1
Quantification for graph in Figure 4A,B and C.
- https://cdn.elifesciences.org/articles/56825/elife-56825-fig4-data1-v2.xlsx
-
Figure 4—source data 2
Quantification for graph in Figure 4D,E and G.
- https://cdn.elifesciences.org/articles/56825/elife-56825-fig4-data2-v2.xlsx
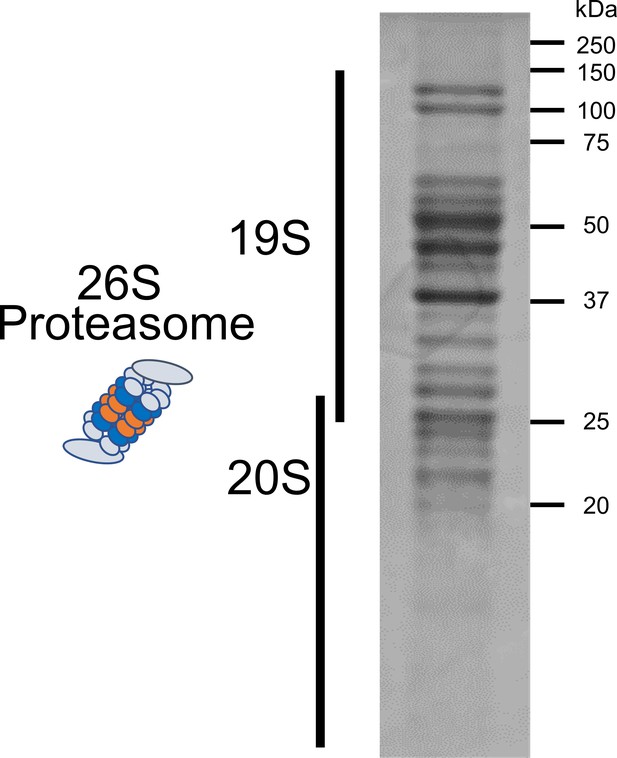
Purification of 26S proteasome.
CBB staining of 26S proteasome complex used in this study.
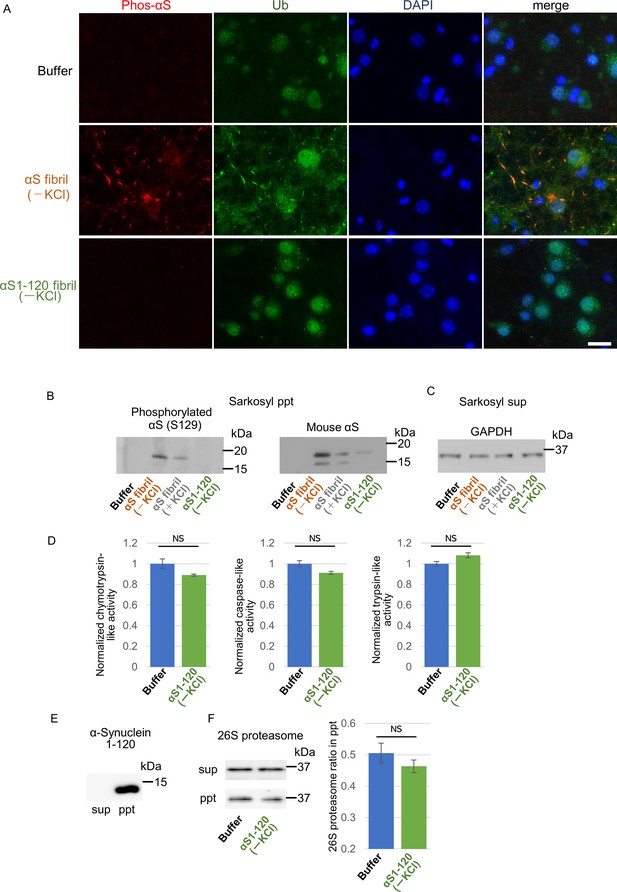
Effects of C-terminal truncated α-synuclein fibrils on proteasome in neurons and in vitro.
(A–C) Seeding activities of C-terminal truncated α-synuclein fibrils in primary mouse cortical neurons. C-terminal truncated α-synuclein fibrils (αSΔC20 (KCl-)) were transduced into primary mouse cortical cells. 14 days after fibril transduction, the accumulation of abnormal phosphorylated α-synuclein and ubiquitinated proteins are detected by immunofluorescence microscopy and western blotting. (A) Phosphorylated α-synuclein (Phos-αS), ubiquitin (Ub) and nuclei (DAPI) were stained. Scale bar, 20 µm. (B) Detection of sarkosyl insoluble phosphorylated α-synuclein (left) and endogenous mouse α-synuclein (right) by western blotting. (C) Detection of sarkosyl soluble GAPDH as a loading control. (D) Effects of the αSΔC20 (KCl-) fibrils on the 26S proteasome activity. 26S proteasome activities in the presence of the αSΔC20 (KCl-) fibrils were measured. Chymotrypsin like activity (left), caspase like activity (center) and trypsin like activity (right) are shown. (mean ± S.E.M; n = 3). (E and F) Co-precipitation of 26S proteasome with the αSΔC20 (KCl-) fibrils. 26S proteasomes were mixed with the αSΔC20 (KCl-) fibrils and centrifuged. Resulted supernatants (sup) and precipitates (ppt) were analyzed by western blotting against α-synuclein (E) and Rpn11-HA (F, left). Quantification of the Rpn11-HA in ppt fractions were shown (F, right). (mean ± S.E.M; n = 3) Analysis was performed using student t test.
-
Figure 5—source data 1
Quantification for graph in Figure 5D and F.
- https://cdn.elifesciences.org/articles/56825/elife-56825-fig5-data1-v2.xlsx
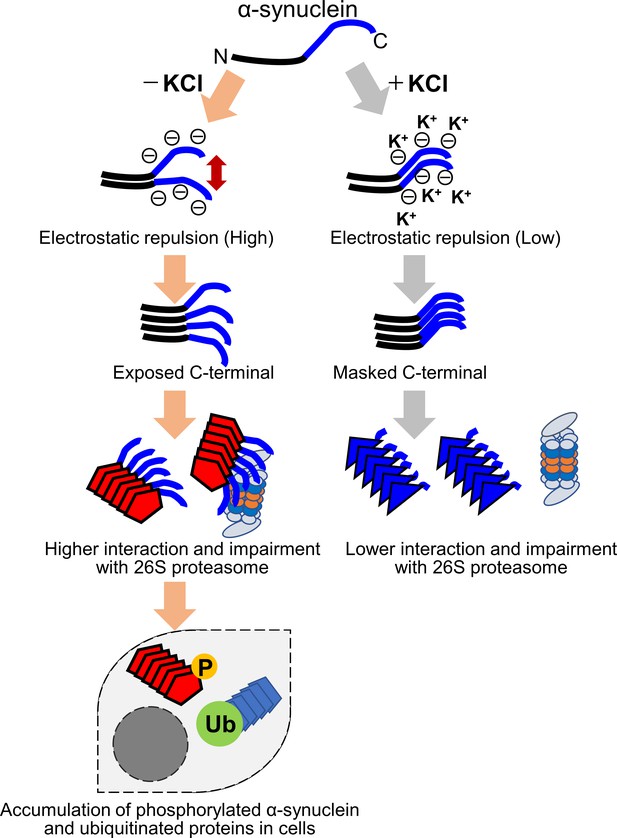
Schematic representation of α-synuclein strain formation and strain dependent interaction with 26S proteasome.
In the absence of salt, α-synuclein monomers have exposed C-terminal region with high electric repulsion. These form the α-synuclein strain with exposed C-terminal region and this type of the α-synuclein strain can interact with 26S proteasomes and inhibit their activities resulting the accumulation of phosphorylated α-synuclein aggregates and ubiquitinated proteins (left). In the presence of salt, α-synuclein monomers have packed C-terminal region with low electric repulsion. These form the α-synuclein strain with masked C-terminal region and this type of the α-synuclein strain can not interact with 26S proteasomes.
Tables
Reagent type (species) or resource | Designation | Source or reference | Identifiers | Additional information |
---|---|---|---|---|
Genetic reagent (Mus musculus) | C57BL/6J | CLEA, Japan, Inc | RRID:IMSR_JAX:000664 | WT mouse |
Genetic reagent (Saccharomyces cerevisiae) | RPN11-FLAGx3 | This paper | ||
Strain, strain background (Escherichia coli) | BL21(DE3) | Sigma-Aldrich | Cat. #: 69450 | |
Recombinant DNA reagent | pRK172-α-synuclein | PMID:8194594 | ||
Recombinant DNA reagent | pRK172-α-synucleinΔC20 | PMID:30030380 | ||
Antibody | anti-α-synuclein-pSer129 #64 (mouse monoclonal) | FUJIFILM Wako Chemicals | RRID:AB_2537218 | (1:1000) |
Antibody | anti-α-synuclein 1–10 (rabbit polyclonal) | Cosmo Bio | RRID:AB_2860557 | (1:1000) |
Antibody | anti-α-synuclein 131–140 (rabbit polyclonal) | Cosmo Bio | RRID:AB_2860558 | (1:1000) |
Antibody | anti-α-synuclein 91–99 (mouse monoclonal) | BD Bioscience | RRID:AB_398108 | (1:1000) |
Antibody | anti-α-synuclein-pSer129 (EP1646Y) (rabbit monoclonal) | Abcam | RRID:AB_869971 | (1:1000) |
Antibody | anti-α-synuclein 115–122 (LB509) (mouse monoclonal) | Santa Cruz Biotechnology | RRID:AB_785898 | (1:200) |
Antibody | anti-α-synuclein D37A6 (rabbit monoclonal) | Cell Signaling Technology | RRID:AB_1904156 | (1:1000) |
Antibody | anti-α-synuclein-pSer129 (1175) (rabbit polyclonal) | PMID:23466394 | (1:1000) | |
Antibody | anti-α-synuclein (Syn205) (mouse monoclonal) | Cell Signaling Technology | RRID:AB_490798 | (1:1000) |
Antibody | anti-ubiquitin (rabbit polyclonal) | Proteintech | RRID:AB_671515 | (1:1000) |
Antibody | anti-ubiquitin (rabbit polyclonal) | Dako | RRID:AB_2315524 | (1:10000) |
Antibody | anti-GAPDH (mouse monoclonal) | Sigma-Aldrich | RRID:AB_2107445 | (1:1000) |
Antibody | anti-FLAG M2 (mouse monoclonal) | Sigma-Aldrich | RRID:AB_262044 | (1:1000) |
Antibody | anti-GFAP (D1F4Q) (rabbit monoclonal) | Cell Signaling Technology | RRID:AB_2631098 | (1:200) |
Antibody | anti-Neurofilament-L (C28E10) (rabbit monoclonal) | Cell Signaling Technology | RRID:AB_823575 | (1:200) |
Antibody | anti-NeuN (EPR12763) (rabbit monoclonal) | abcam | RRID:AB_2532109 | (1:1000) |
Antibody | anti-tau (tauC) (rabbit polyclonal) | PMID:26374846 | (1:200) | |
Antibody | Goat anti-rabbit IgG, Alexa Fluor 488 | Thermo Fisher | RRID:AB_143165 | (1:1000) |
Antibody | Goat anti-mouse IgG, Alexa Fluor 568 | Thermo Fisher | RRID:AB_2534072 | (1:1000) |
Antibody | Goat anti-rabbit IgG, HRP | Thermo Fisher | RRID:AB_2536099 | (1:10000) |
Antibody | Goat anti-mouse IgG, HRP | Thermo Fisher | RRID:AB_2536163 | (1:10000) |
Antibody | Goat anti-rabbit IgG, biotin | Vector laboratories | RRID:AB_2313606 | (1:1000) |
Software, algorithm | EZR | PMID:23208313 | ||
Other | hematoxylin | Muto Pure Chemicals | Cat. #: 30002 | |
Other | DAPI stain | Vector laboratories | Cat #: H-1500 |