A Sec14-like phosphatidylinositol transfer protein paralog defines a novel class of heme-binding proteins
Figures
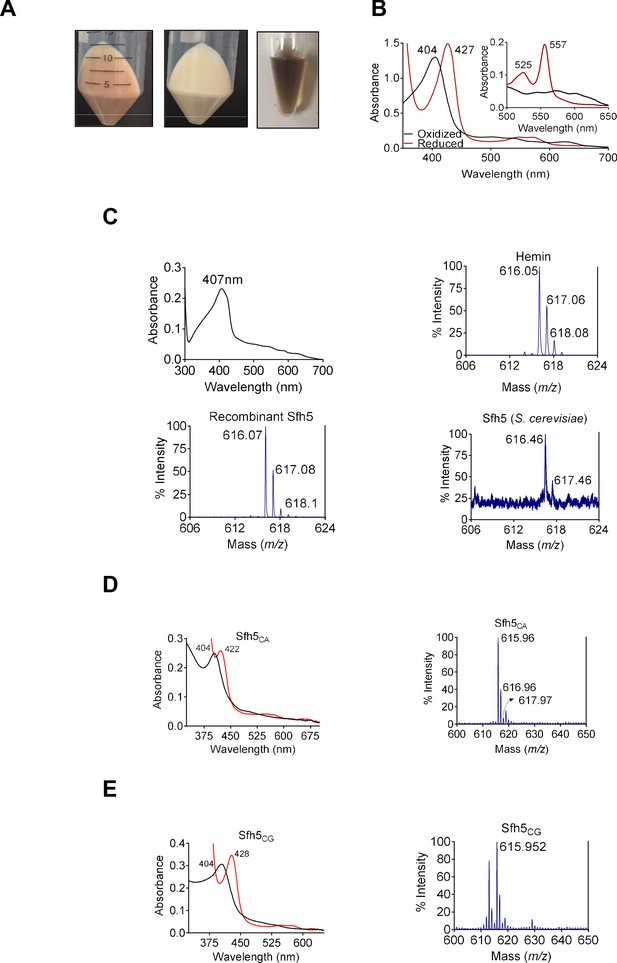
Sfh5 is a heme-binding protein.
(A) Cell pellets of E. coli BL21(DE3) culture expressing Sfh5 are reddish brown color (left panel) relative to cell pellets of BL21(DE3) expressing Sec14 (middle panel). Panel at right shows an image of a purified recombinant Sfh5 (500 µM) solution which is distinguished by a deep reddish brown color. (B) UV-vis absorption spectrum of purified Sfh5 is traced in black. Spectra in red identify the spectral profile of Sfh5 after reduction with dithionite. Inset shows the 500 to 650 nm region of the UV-vis spectrum from pyridine hemochromagen assays. The indicated β and α bands at 525 and 557 nm, respectively, diagnose a noncovalently-bound heme b. (C) Top left: UV-vis absorption spectrum of a clarified cell lysate prepared from E. coli expressing Sfh5 absent an N’ terminal 8xHis tag. The spectra were calibrated against a cell lysate prepared in parallel from mock-expressing E. coli cells (vector-only). Top left: The UV-vis absorption spectrum of clarified cell lysate prepared from E. coli BL21 (DE3) cells expressing tag-less Sfh5. Mock lysate prepared from E. coli BL21 (DE3) cells was used as blank to calibrate the spectrophotometer. Both E. coli cultures were induced with 100 µM IPTG and incubated overnight at 16 °C prior to harvesting and lysing cells. Top right: MALDI-TOF mass spectrum signature of hemin (positive standard), Bottom left and bottom right panels show the heme signatures of purified recombinant Sfh5 and Sfh5 purified directly from S. cerevisiae, respectively. Peaks at 616 ± 2 Da constitute a signature for protoporphyrin IX. Total spectral counts within the indicated m/z ranges were as follows: hemin, 30821; recombinant Sfh5, 8038; S. cerevisiae Sfh5, 672. (D, E) UV-vis absorption (left panels) and MALDI-TOF mass spectra (right panels) of purified recombinant Sfh5 sourced from C. albicans (Sfh5CA) and C. glabrata (Sfh5CG). Spectral profiles of dithionite-treated versions of these proteins were red-shifted to 422 and 428 nm for Sfh5CA and Sfh5CG, respectively. Total counts within the indicated m/z ranges were 1404 and 1739 for Sfh5CA and Sfh5CG, respectively.
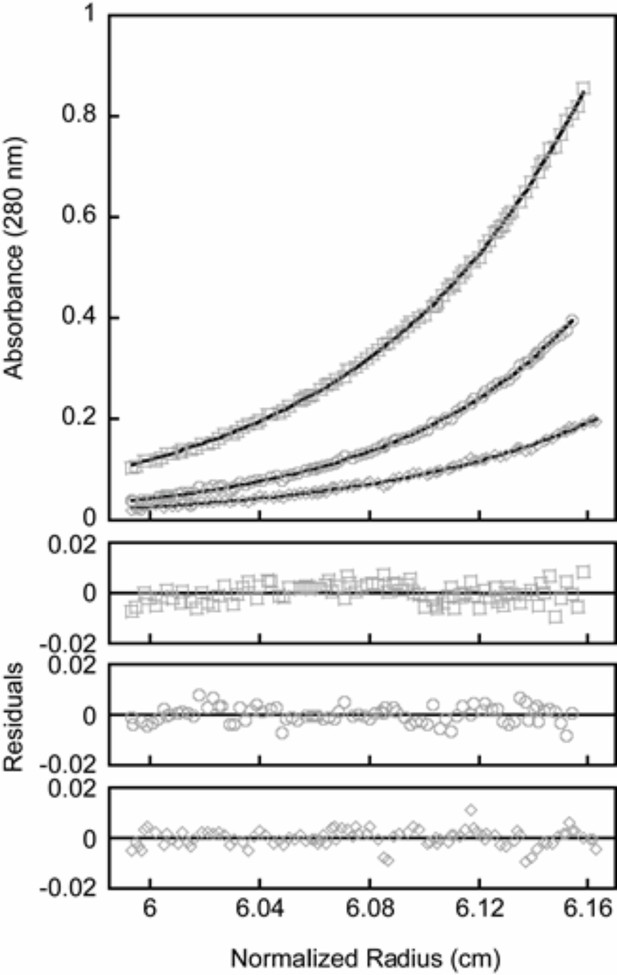
Sfh5 oligomerization and the unit cell.
Representative analytical ultracentrifugation equilibrium data (gray symbols) and the corresponding global single ideal species fit (solid black lines) are shown for starting concentrations of 10 µM (squares), 5 µM (circles) and 2.5 µM (diamonds) Sfh5 at 15,000 rpm. The residuals of the fit for each concentration are also shown. The estimated molecular mass of the global fit (70,826 Da) corresponds to a dimeric assembly (MWobs/MWcalc = 1.99). When centrifuged at 20,000 RPM, Sfh5 begins to aggregate at the bottom of the cell, and the dimeric single ideal species fit no longer adequately fits the data.
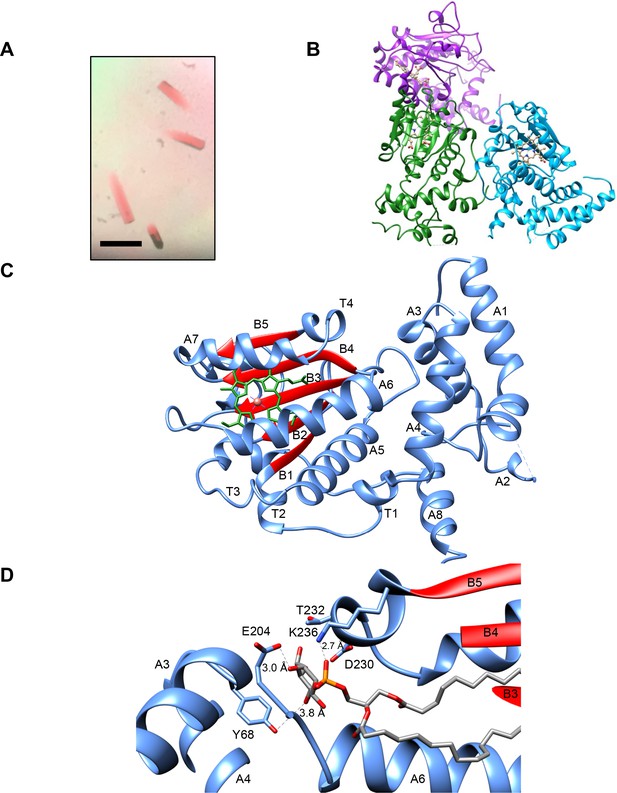
Sfh5 crystal structure.
(A) Recombinant Sfh5 forms reddish brown rod-shaped crystals. Scale bar, 50 µm. (B) The asymmetric unit consists of three Sfh5 molecules and ribbon representations are colored by molecule. The bound heme is shown in ball and stick with carbon atoms colored in brown, oxygen – in red, and nitrogen in blue. (C) Ribbon diagram of the Sfh5 showing α-helices, loops and 310 turns in blue, and β-strands in red. A single non-covalently-bound heme b molecule is rendered in green with the brown sphere representing the heme iron. (D) The PtdIns binding substructure of Sec14-like PITPs is conserved in Sfh5. A PtdIns molecule was modeled into the closed Sfh5 structure by overlaying Sfh1::PtdIns crystal structure (PDB ID: 3B7N) onto the Sfh5 crystal structure. This binding model emphasizes the conserved interactions (dashed lines) between Sec14 family PtdIns-binding barcode residues of Sfh5 and elements of the PtdIns headgroup. The corresponding distances between PtdIns headgroup structural elements and side-chains of the barcode residues in this dock model are shown.
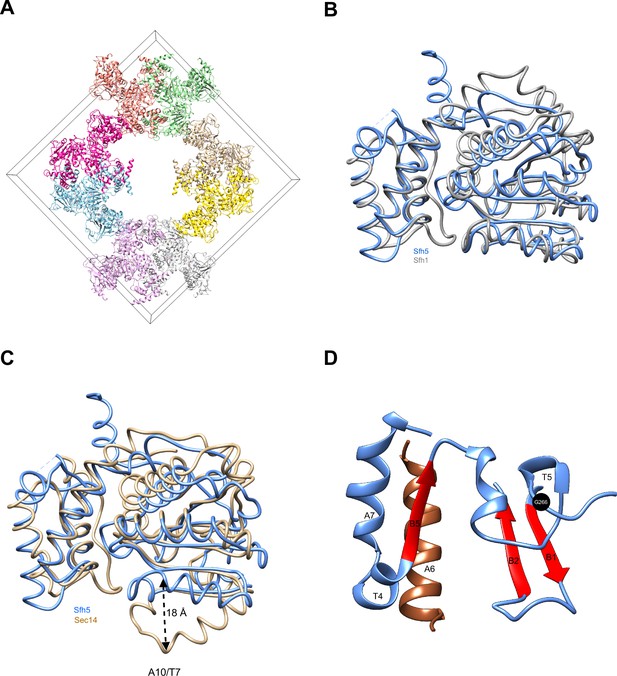
Structural features of Sfh5.
(A) Sfh5 crystal packing in the unit cell is shown. Each asymmetric unit within the unit cell is differentially colored for purpose of illustration. (B) Superposition of the Sfh5 (blue) and Sfh1 (silver) α-carbon backbones (rmsd 6.26 Å). Sfh1 represents the Sec14-like paralog most identical to Sec14 and it crystallizes in the closed conformation as does Sfh5. Bound PtdIns (Sfh1) and heme (Sfh5; pdb 3B7N) are omitted from the overlay. (C) Superposition of the Sfh5 (blue) and Sec14 (gold; pdb 1AUA) α-carbon backbones (rmsd 6.63 Å). Sec14 crystallizes as the open conformer. The displacement of the Sec14 gating helix relative to that of Sfh5 is highlighted and reflects the difference in atomic distance between reference residues K192 and F228 for Sfh5 and the Sec14 cognates R195 and F231. Bound β-octylglucoside (Sec14) and heme (Sfh5) are omitted from the overlay. (D) The Sfh5 G-module is a conformational switch element that consists of a series of short 310 helices organized into two distinct substructures, the β1-loop-β2 and the extended T5 loop linked to β5 (Ryan et al., 2007; Schaaf et al., 2008). Hydrogen bonds between these two elements contributes to opening and closing of the chamber gating helix. The close proximity of helices A7 (in blue) and A6 (in copper) indicate a ‘closed’ version of the protein. G260, analogous to residue G266 of the Sec14 G-module is shown as black sphere.
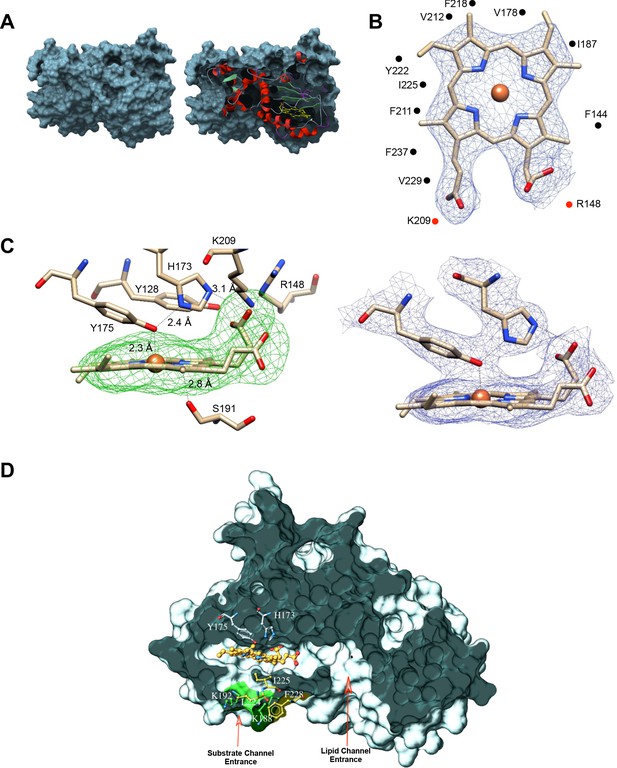
Coordination environment of Sfh5-bound heme.
(A) Left panel shows the surface rendering of Sfh5 in grey. Right panel shows surface view of Sfh5 clipped to expose the buried heme rendered in yellow ball and stick with protein secondary structure elements depicted in cartoon ribbon. (B) Heme bound to chain B of the Sfh5 crystal structure. The 2Fo-Fc electron density map for heme contoured at 1.5 σ is displayed as blue mesh. Residues engaged in van der Waals contacts with heme are shown as gray spheres. The side-chains of residues interacting with the propionyl groups of heme (K209 and R148) are presented as red dots. (C) Left panel: Sfh5 residues that bind heme are shown including the Fe-coordinating residues Y175 and H173. Dashed lines identify the inter-atomic distances between the indicated Sfh5 residues and cognate components of heme. Polder omit electron density map for heme is shown as green mesh (Liebschner et al., 2017), contoured at 2.5 σ. Right panel: Lateral view of the 2Fo-Fc map showing electron density associated with residues coordinating the heme iron center (contoured at 1.5σ). (D) Surface view of Sfh5 clipped to display the ligand-binding cavity. Bound heme (shown in ball and stick and colored by the element with carbons in gold) resides deep inside the cavity. Heme-coordinating residues H173 and Y175 are displayed in ball and stick mode and colored by element with carbons in light gray. Indicated access to the vacant heme coordination site from solvent is potentially controlled by conformational transitions of the K188 and K192 side chains (labeled as substrate channel, entrance displayed in ball and stick render and colored by element with carbons in green), and the side-chains of residues L224, I225 and F228 (colored by element with carbons in yellow). The surface contributed by the side chains of residues K188 and K192 is colored in green. Access channel for lipid to the internal cavity of other Sec14-like PITPs is also indicated.
-
Figure 3—source data 1
Fungal Sfh5 orthologs.
The S. cerevisiae Sfh5 primary sequence was used as query in a Uniprot BLAST search to identify orthologs across the fungal kingdom. Initial sequence alignments were performed using ClustalW and followed by a Sfh5 structure guided alignment of the sequences using ESCRIPT. Sequences from C. glabrata, C. albicans, C. tropicalis, C. auris, A. nidulans, A. oryzae, N. crassa, C. neoformans and Y. lipolytica were aligned. Conserved residues are highlighted in red. At bottom are identified the Sfh5 residues that: (i) correspond to the PtdIns-binding barcode common to Sec14-like PITPs (▲), (ii) that are engaged in van der Waals interactions with heme or π-π stacking interactions with the pyrrole groups of the porphyrin ring (●), (iii) engage in electrostatic or H-bond interactions with heme -- that is. the heme-binding barcode (♦) and (iv) conserved residues Lys188 and Lys192 that are poised to gate access to the empty pocket distal to the heme iron (arrows). Secondary structure elements as determined from the Sfh5 crystal structure are identified at top.
- https://cdn.elifesciences.org/articles/57081/elife-57081-fig3-data1-v2.pdf
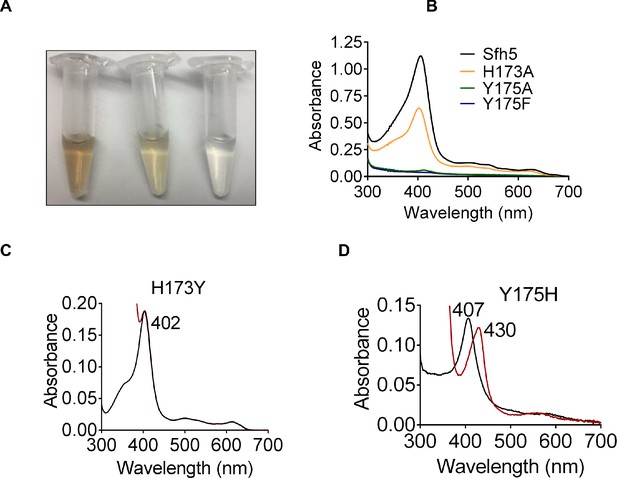
Roles of Sfh5 residues Y175 and H173 in heme binding and Fe-center chemistry.
(A) The color intensities of solutions (30 μM) of purified recombinant Sfh5 (left), Sfh5H173A (middle) and Sfh5Y175F (right) are compared. (B) UV-vis absorption spectra of Sfh5, Sfh5H173A, Sfh5Y175A and Sfh5Y175F are shown. The protein concentrations were fixed at 30 μM. (C, D) UV-vis spectra of Sfh5H173Y and Sfh5Y175H are shown, respectively. Spectra obtained after reduction of sample with dithionite are in red. Sfh5H173Y did not undergo a spectral shift upon reduction.
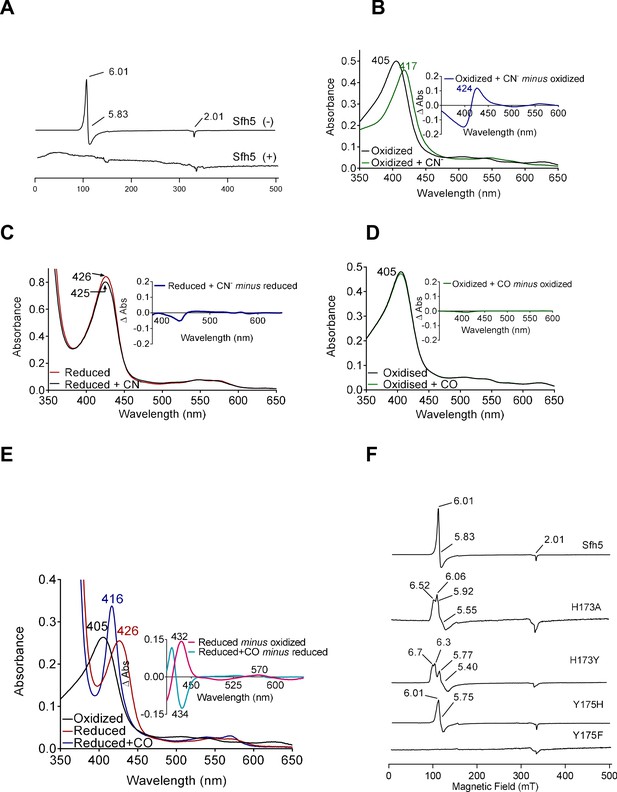
Electronic properties of the Sfh5 Fe-center.
(A) EPR spectra of purified Sfh5 before (-) and after dithionite treatment (+). (B) UV-visible absorption spectrum of purified (oxidized) Sfh5 without and with treatment with potassium ferricyanide as source of cyanide ion (CN-) (Blumenthal and Kassner, 1980). Inset shows the difference spectrum between the two conditions and highlights a significant red shift in the Soret maximum induced by CN-. (C) Purified Sfh5 was first reduced with excess dithionite and spectra were taken before and after incubation in the presence of CN-. Inset indicates the difference spectrum between the two conditions and shows no appreciable shift in the Soret maximum. (D) UV-vis spectra before and after incubation of purified Sfh5 with carbon monoxide (CO) gas are shown. Inset indicates the difference spectrum between the two conditions and shows no shift in the Soret maximum in the presence of CO. (E) UV-vis spectra of purified Sfh5 reduced with dithionite and incubated in the presence of CO. Inset, difference spectra as indicated. (F) EPR spectra of purified Sfh5, Sfh5H173A, Sfh5H173Y, Sfh5Y175H, and Sfh5Y175F are shown. Protein samples were normalized by concentration (150 μM).
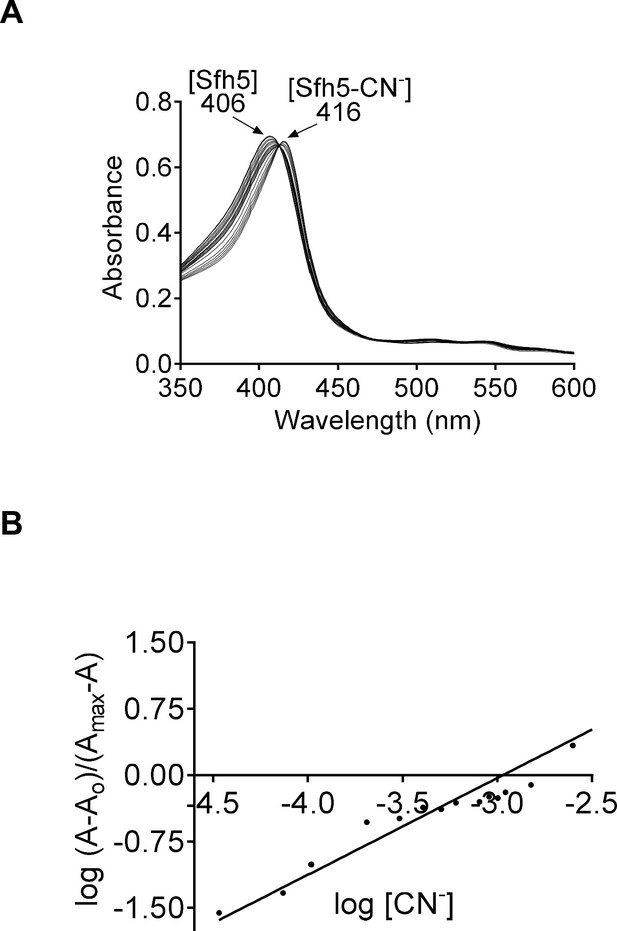
Accessibility of the open axial binding site of Sfh5 by CN-.
(A) Sfh5 (10 μM solution, pH 7.0) was titrated with increasing concentrations of freshly prepared KCN solution and the UV-vis spectra were collected. The Soret maxima peak shifted from 406 to 416 nm as concentration of KCN increased. Isosbestic point at 413 nm was observed. (B) Stoichiometry was determined from a plot of versus , where A0 is the starting absorbance and Amax is the maximum absorbance. A simple one-ligand binding event was assumed expressed as: . A straight line with a slope of 1.094 ± 0.09 was calculated, consistent with a ‘one ligand binding’ model.
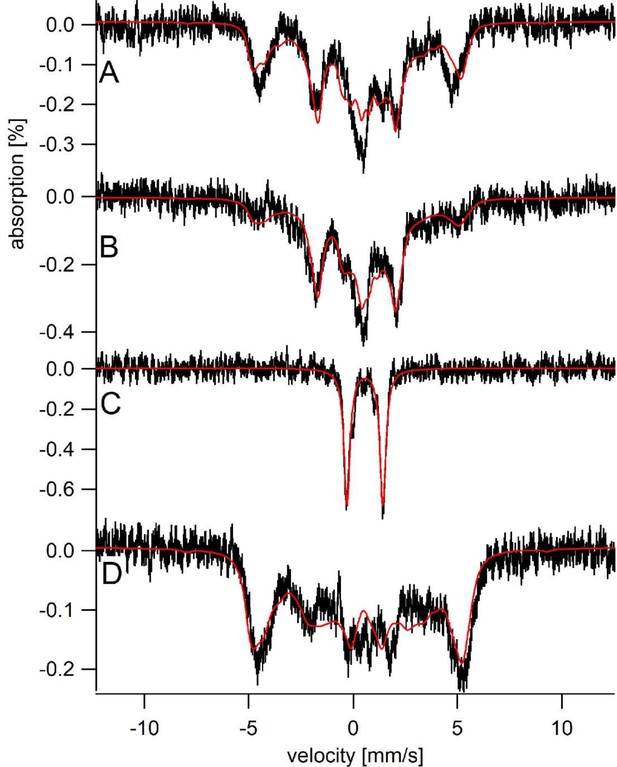
Sfh5 heme center exhibits two magnetic states.
Mössbauer spectra were collected at low-temperature (5 K) and field (0.05 T). (A) Spectrum of Sfh5 sample prepared at pH 7. The red line is a composite simulation with 63% FeIIILA site and 37% FeIIISA site. (B) Spectrum of an independent sample of Sfh5 prepared at pH 8. (C) Spectrum of the sample of Sfh5 in (B) reduced with sodium dithionite. The simulation in B was used to remove 60% of the overall spectral intensity in C. (D) Spectrum of Sfh5H173A100% FeIIILA site. In all spectra, the field was applied parallel to the gamma radiation.
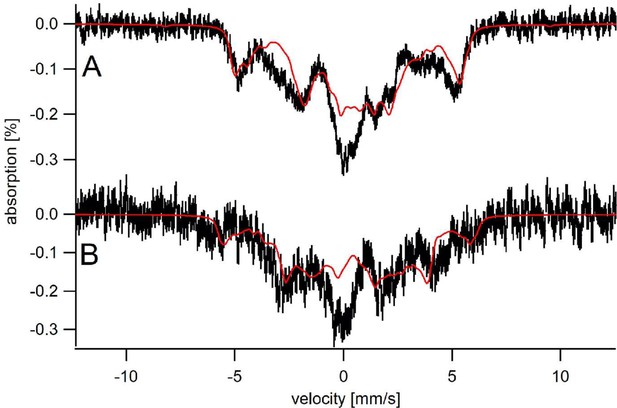
High-field spectrum of 57Fe-enriched Sfh5.
The 4.2 K Mössbauer spectra of Sfh5 at 0 T (A) and 6.0 T (B) applied fields. Red lines are simulations assuming those applied fields at 50% FeIIILA site and 35% FeIIISA site. The simulations did not include 15% of the overall spectral intensity. The field was applied perpendicular to the gamma radiation.
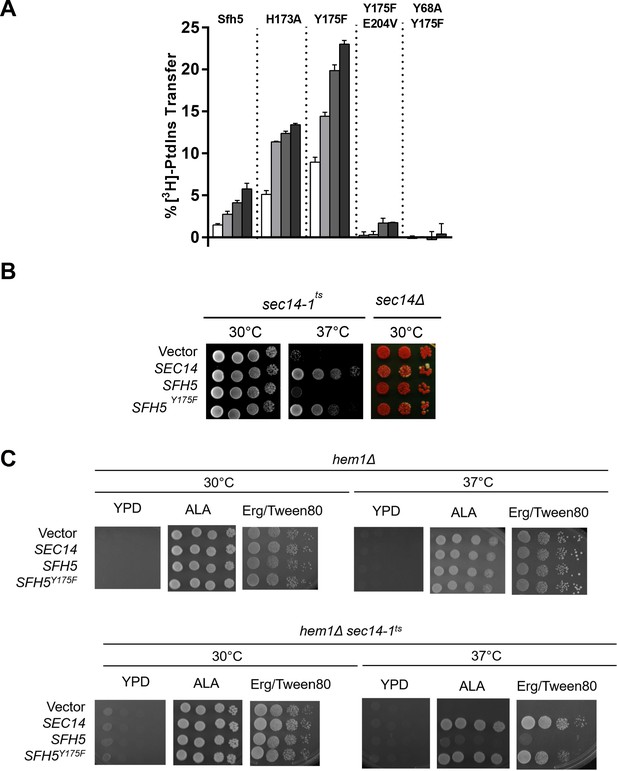
Incompatibility of high affinity Sfh5 heme-binding with PtdIns binding.
(A) In vitro [3H]-PtdIns transfer assays were performed with purified recombinant Sfh5, Sfh5H173A and Sfh5Y175F in a titration series where protein inputs were increased in steps of two-fold (5, 10, 20, 40 µg). The total radiolabeled input for each assay varied between 8377 to 12,070 cpm, and background ranged between 150–575 cpm. The transfer values represent the mean of triplicate assay determinations from at least two independent experiments. (B) Resuscitation of PITP activity in heme-deficient Sfh5 mutants in vivo. Left panel: A sec14-1ts strain was transformed with episomal YEp(URA3) plasmids that drive constitutive ectopic expression of SEC14, SFH5 or sfh5Y175F from the powerful PMA1 promoter. The transformants were spotted in 10-fold dilution series onto YPD plates and incubated at the indicated temperatures for 48 hr before imaging. Growth at 37°C reports rescue of growth defects associated with the sec14-1ts allele at this normally restrictive temperature. Right panel: A sec14Δ ade2 ade3/YEp(SEC14, LEU2, ADE3) strain was transformed with the indicated YEp(URA3) expression plasmids described in (B). Transformants were again spotted in dilution series onto YPD plate as above. Under those conditions, all nutrient selections are relieved and loss of the normally essential YEp(SEC14, LEU2, ADE3) plasmid (which has the dual properties of covering the lethal sec14Δ allele and also the ade3 allele which coverts colony color of ade2 cells from red to white) can be monitored. Appearance of white colonies that are phenotypically Leu auxotrophs signifies loss of the parental YEp(SEC14, LEU2, ADE3) plasmid on the basis of the YEp(URA3) plasmid driving expression of a functional PITP. Uniformly red colonies report the YEp(URA3) expression plasmid does not drive production of a functional PITP with the capacity to provide Sec14-like functions to Sec14-deficient cells. YEp(SEC14) serves as positive control in these experiments. (C) PITP activity is not resuscitated in Sfh5 expressed in yeast cells devoid of heme. Isogenic hem1Δ and hem1Δ sec14-1ts strains deficient in α-aminolevulenic acid (ALA) synthesis were transformed with YEp(URA3) plasmid as mock control or for expression of SEC14, SFH5 or sfh5Y175F as indicated. Transformants were selected on a minimal media plate without uracil and supplemented with 250 µM ALA. After depleting cells for residual heme by growth in ergosterol-containing medium (added to a 20 mg/l final concentration from a 0.2% stock solution in 1:1 ethanol: Tween 80), cells were spotted onto YPD solid medium or onto YPD solid medium supplemented with ALA (250 µM) to rescue the hem1Δ deficiency, or onto YPD solid medium supplemented with 50 µM ergosterol/Tween 80 (Erg/Tween80) to rescue the heme deficiency without permitting heme synthesis. The cells were incubated for 72 hr at the indicated temperatures prior to imaging. The hem1Δ control cells grow under all conditions that either rescue heme synthesis (+ALA) or provide exogenous ergosterol whose synthesis is the sole essential heme-dependent activity in cells cultured under these conditions. Growth of the hem1Δ sec14-1ts mutant at the restrictive temperature of 37C was not rescued by enhanced expression of WT Sfh5 under heme-less conditions (i.e. on YPD supplemented with Erg/Tween80). Expression of SEC14 or the sfh5Y175F heme-binding mutant served as additional positive controls.
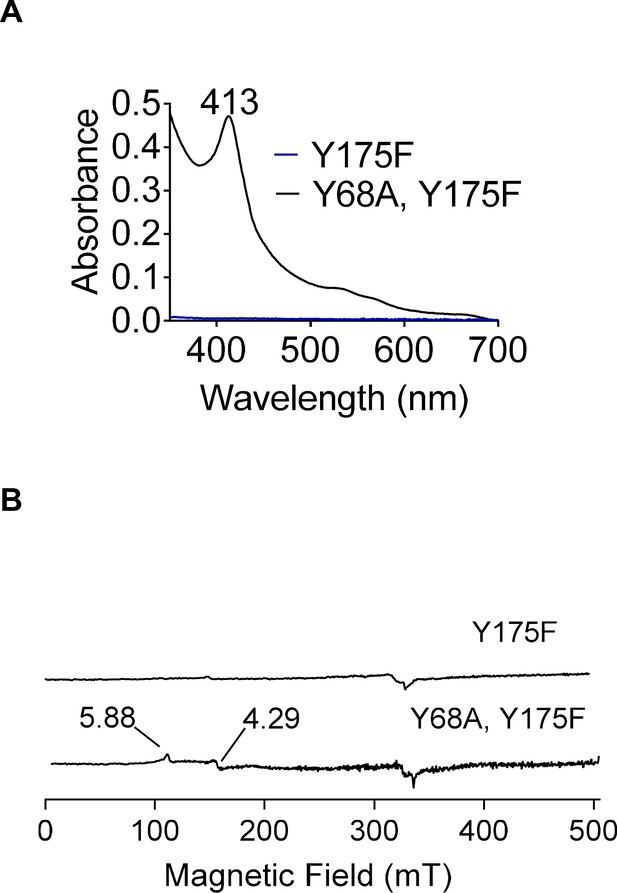
Partial restoration of heme binding to Sfh5Y175F by alteration of a residue of the PtdIns-binding barcode.
(A) UV-vis absorption spectra of Sfh5Y175F and Sfh5Y68A,Y175F (225 µM solutions) are shown. A clear Soret maximum at 413 nm for Sfh5Y68A,Y175F is evident. (B) EPR spectra of Sfh5Y175F and Sfh5Y68A,Y175F proteins are shown (225 µM solutions). A discernible peak at g = 5.88 (reporter of high-spin Fe3+ heme) is recorded for Sfh5Y68A,Y175F but not for the heme-less Sfh5Y175F parental control. Signal for a minor non-heme iron species was also observed (g = 4.29).
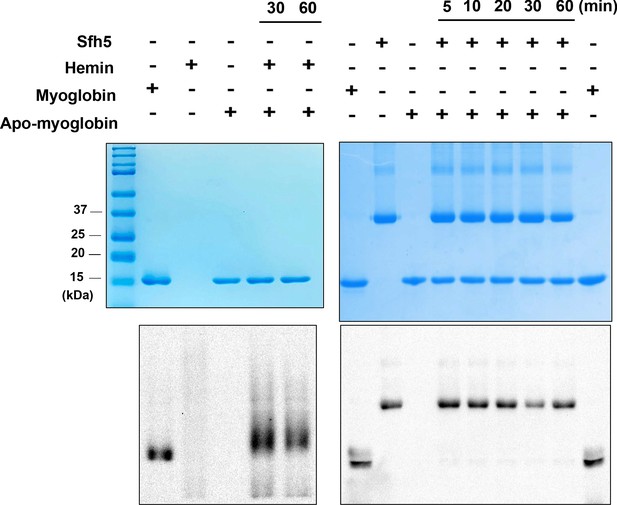
Sfh5 does not donate bound heme to an efficient heme scavenger.
Top panels: Coomassie-stained SDS-PAGE gel images of Sfh5, myoglobin and apo-myoglobin proteins run individually and mixtures of hemin/apo-myoglobin and Sfh5/apo-myoglobin (as indicated) that were pre-incubated for the indicated times. The proteins migrate with the expected masses of 35.7 kDa for the Sfh5 monomer and 18 kDa for myoglobin/apo-myoglobin. Bottom panels show corresponding chemiluminescent images of the nitrocellulose membranes onto which the proteins from duplicate SDS-PAGE gels were transferred and probed by visualizing pseudo-peroxidase activity in situ. Whereas apo-myoglobin avidly scavenged hemin from the medium, no measurable transfer of heme from Sfh5 to apo-myoglobin was detected.
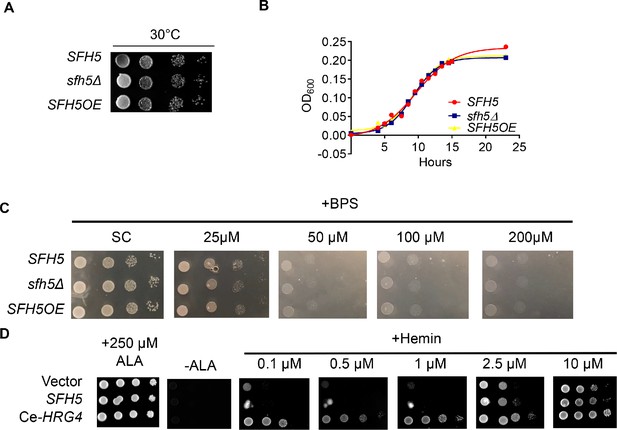
Sfh5 deficient cells are not compromised for major heme-requiring functions.
(A) Cultures of of isogenic wild-type, sfh5Δ and Sfh5 over-expressing (OE; Sfh5 expression driven by the PMA1 promoter in the context of a genomic replacement cassette) were spotted on yeast peptone (YP) agar plates supplemented with glycerol as carbon source and incubated for 60 hr at 30°C. (B) Growth-curves for the strains described in (A) in YP-glycerol liquid media. (C) Wild-type Sfh5, sfh5Δ and SFH5OE strains grown in YPD media then harvested and washed in minimal medium plus glucose. All strains were sub-cultured in the same minimal medium for 12 hr, followed by spotting of 10-fold serial dilutions of innoculum on synthetic defined media agar plates supplemented with glucose and varied concentrations of bathophenanthroline disulfonic acid (BPS) as indicated. Plates without BPS served as control. All plates were incubated at 30°C for 36 hr and imaged. (D) A hem1Δ strain was transformed with YEp(URA3) plasmid driving galactose-inducible expression of either SFH5 or C. elegans HRG4 genes. The backbone vector plasmid served as control. After depletion of residual heme, ten-fold dilutions of cells were spotted onto minimal media plates supplemented with either α-aminolevulenic acid (ALA) or hemin as indicated. The plates were imaged after incubation at 37°C for 72 hr.
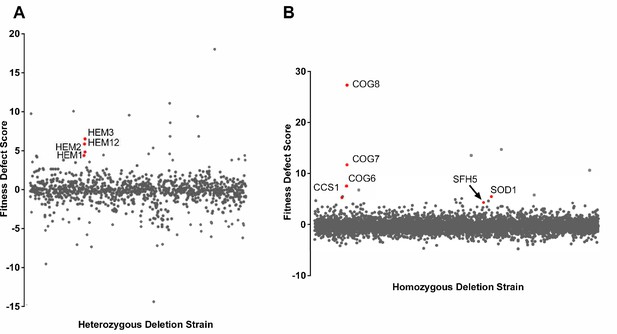
Specific sensitivity of Sfh5 deficient cells to oltipraz.
(A) Chemogenomic profiles of the diploid yeast heterozygous deletion collection challenged with oltipraz as expressed as a log2-scaled fitness defect (FD; Lee et al., 2014). Fitness or growth defects associated with haplo-insufficiency profiling (HIP) are represented on the y-axis, and the x-axis represents statistically significant hits (p<0.05). Note the enrichment of hits directly associated with heme biosynthesis (red dots). These data are adapted from the following searchable database (http://chemogenomics.pharmacy.ubc.ca/HIPHOP/). (B) Chemogenomic profiles of the diploid yeast homozygous deletion collection challenged with oltipraz (Lee et al., 2014). The sfh5Δ homozygote is identified. Significant hits related to deficiencies in redox enzymes and Golgi trafficking components of the COG complex are also highlighted in red.
Tables
X-ray data collection and refinement statistics.
Parentheses indicate highest shell.
Data Collection | Phasing data set | Refinement data set |
---|---|---|
Wavelength (Å) | 1.55 | 1.55 |
Space group | P43212 | P43212 |
a, b, c (Å) | 205.13, 205.13, 68.27 | 205.34, 205.34, 68.31 |
α, β, γ (°) | 90, 90, 90 | 90, 90, 90 |
Resolution (Å) | 41–2.7 (2.8–2.7) | 29.3–2.9 (3.06–2.9) |
Rmerge | 0.291 (1.736) | 0.157 (2.22) |
Rpim | 0.049 (0.545) | 0.064 (0.881) |
I/σI | 13.5 (0.8) | 10.2 (1.2) |
Completeness (%) | 98.5 (85.3) | 99.6 (100) |
Redundancy | 32.2 (9.1) | 6.9 (7.0) |
CC1/2% | 0.996 (0.303) | 0.996 (0.505) |
Refinement | ||
Resolution (Å) | 29.3–2.9 | |
No. of Reflections: | 61477 | |
Rwork | 0.23 | |
Rfree | 0.30 | |
No. of atoms | 7169 | |
Protein | 7040 | |
Ligand | 129 | |
B-factors (Å2) | ||
Protein | 79.3 | |
Ligands | ||
R.M.S. deviations: | 57 | |
Bond lengths, rmsd (Å) | 0.01 | |
Bond angles, rmsd (°) | 1.41 | |
Ramachandran plot | ||
Ramachandran favored (%) | 86 | |
Ramachandran allowed (%) | 13 | |
Ramachandran outliers (%) | 0.5 |
Additional files
-
Supplementary file 1
Biochemical and biophysical properties of Sfh5 and mutant derivatives.
- https://cdn.elifesciences.org/articles/57081/elife-57081-supp1-v2.docx
-
Transparent reporting form
- https://cdn.elifesciences.org/articles/57081/elife-57081-transrepform-v2.docx