Rapid stimulus-driven modulation of slow ocular position drifts
Figures
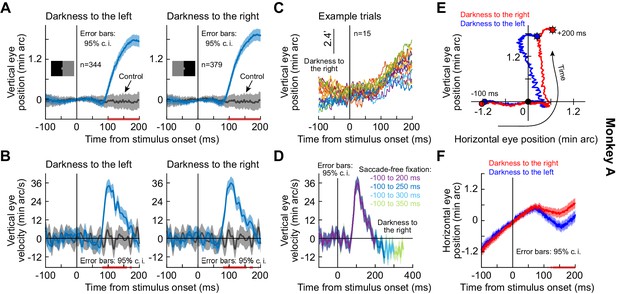
Short-latency, stimulus-driven ocular position drift response.
(A) Vertical eye position (plus 95% confidence intervals) after split view stimulus onset. Compared to the no-stimulus condition (gray), the eye drifted upwards with short latency after stimulus onset. This happened for both right and left darkness stimuli (the two panels). We vertically aligned all starting eye positions at time 0 before averaging trials, to highlight the systematic change in eye position shortly after stimulus onset. (B) Same data as in (A) but for vertical eye velocity. A clear velocity pulse (much slower than that of microsaccades/saccades) is visible. (C) Individual trials demonstrating the drift response. The vertical position of each curve was jittered across the shown trials to facilitate visibility. (D) When we included progressively longer intervals of saccade-free fixation, we confirmed that the drift response (e.g. B) is transient. (E, F) Relative to starting eye position at stimulus onset, the drift response was predominantly vertical, but the horizontal component of eye position was also stimulus-dependent. Error bars in (F) denote 95% confidence intervals. The trajectories in (E) are two-dimensional plots of the average curves in (A), (F). All pink lines on x-axes indicate intervals in which the 95% confidence intervals of the two compared curves did not overlap. Also see Figure 1—figure supplements 1 and 2.
-
Figure 1—source data 1
Excel table with the source data for this figure.
- https://cdn.elifesciences.org/articles/57595/elife-57595-fig1-data1-v2.xlsx
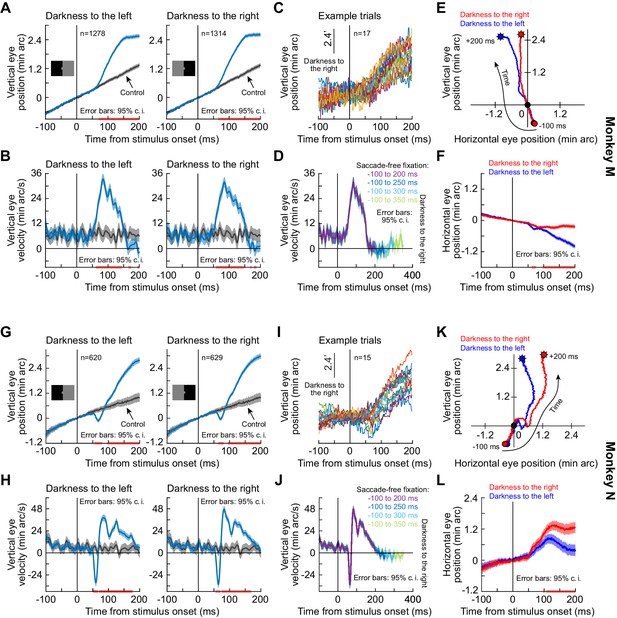
Short-latency, stimulus-driven ocular position drift response in two additional monkeys besides the monkey shown in Figure 1.
This figure is formatted identically to Figure 1. It shows very similar results from two additional monkeys, M and N. (A–F) Monkey M results. (G–L) Monkey N results. Error bars are defined in all relevant panels. The pink horizontal lines on various x-axes denote intervals in which the 95% confidence intervals around the two compared curves did not overlap.
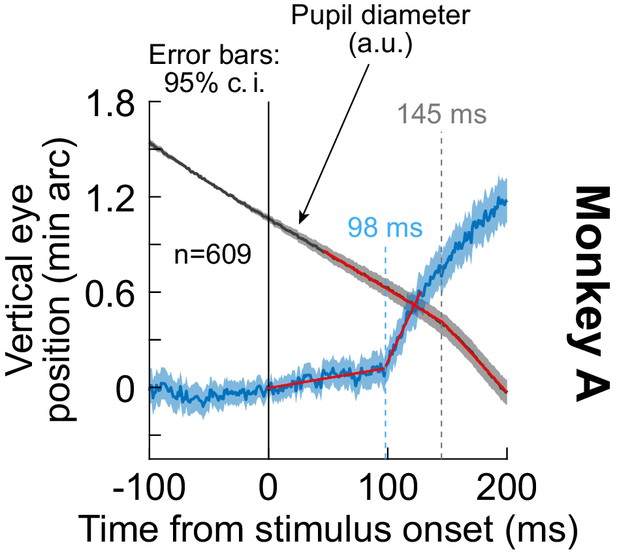
The ocular position drift response occurred earlier than pupil diameter constriction occurring after stimulus onset.
We measured pupil diameter in monkey A when a stimulus transient (high-contrast, full-screen vertical grating) was presented. The blue curve shows the ocular position drift response, replicating the results of Figure 1, Figure 1—figure supplement 1 in new experiments. This response was measured with a scleral search coil in the left eye. The gray curve shows pupil diameter from the same set of trials, measured with a video-based eye tracker aimed at the right eye (the ocular position drift response was unresolvable in the right eye with the video-based eye tracker; Methods). To highlight the change in pupil diameter after stimulus onset within each trial, we averaged pupil diameter in the final 100 ms before stimulus onset, and we then subtracted this average from all pupil measurements within the trial. This aligned the pupil measurements across all trials in the y-axis dimension, isolating the relative changes in pupil diameter occurring after stimulus onset (Methods). This is a similar approach to our procedures for ocular position drift responses (e.g. Figure 1 and the blue curve in the current figure). As can be seen, the inflection point of pupil diameter (indicated by the gray dashed vertical line at 145 ms), demonstrating stimulus-driven pupillary constriction, came significantly later than the onset of the ocular position drift response (indicated by the light blue dashed vertical line at 98 ms). Each inflection point was obtained by fitting a piece-wise linear model to each curve: a linear fit in an early interval before the onset of the ocular position drift response or pupil constriction, and a second linear fit in a later interval after the onset of the ocular position drift response or pupil constriction. This is similar to methods used earlier for estimating smooth pursuit onset latencies (Krauzlis and Miles, 1996). The two linear fits per curve are shown in red. Error bars denote 95% confidence intervals across trials.
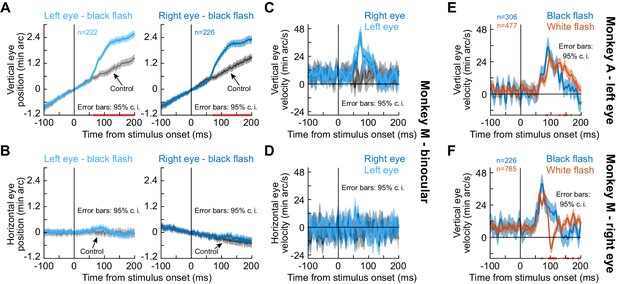
The drift response is a binocular eye movement.
(A) Vertical eye position from monkey M during simultaneous measurements of the right and left eyes. The upward drift response (for a brief ~8 ms black flash covering the whole display) occurred binocularly. Note that this response superseded the monkey’s stereotypical baseline slow upward drift, which was much slower (each monkey had a stereotypical baseline drift direction; Figure 1, Figure 1—figure supplement 1). (B) Horizontal eye position showed no evidence of convergence. (C, D) Same data with vertical and horizontal eye velocity, respectively. The drift response (C) was not associated with a convergence response (D). The lack of horizontal pink lines on the x-axes of (C), (D) confirm that both eyes moved similarly. (E) In monkey A, we confirmed that the same paradigm (brief black flash) was still sufficient (bluish curve) to cause an upward drift response. When we switched the flash to white (reddish curve), the drift response still occurred. (F) Monkey M also showed a drift response for a white flash. Error bars in all panels denote 95% confidence intervals, and pink lines on the x-axes show periods in which the 95% confidence intervals of the two compared curves did not overlap.
-
Figure 2—source data 1
Excel table with the source data for this figure.
- https://cdn.elifesciences.org/articles/57595/elife-57595-fig2-data1-v2.xlsx
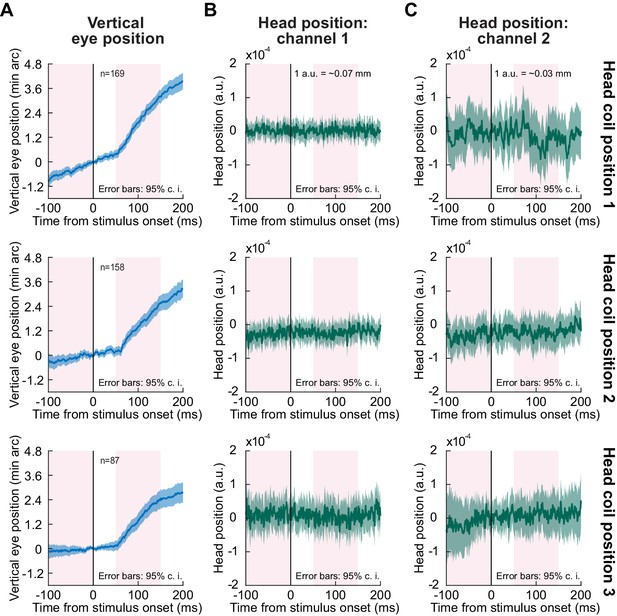
The drift response is independent of head movements.
(A) Each row shows vertical eye position in a format identical to that shown in earlier figures (e.g. Figures 1 and 2). The same drift response was replicated in these three new sets of experiments of the black flash condition. (B, C) Simultaneous measurements of head position for the same sets of trials shown in (A). In each row, we placed a head coil at a different position on the monkey’s head to explore different axes of potential head movements (Methods). Each coil provided two measurements (B, C) of induced electrical current from two orthogonal magnetic fields used to measure position (these were the same two fields used to induce electrical current in the eye coil to allow measuring horizontal and vertical eye position). There was no head movement concomitant with the drift response in any of the channels. The pink intervals illustrate the measurement intervals that we used for statistical assessment of whether there was a response after stimulus onset relative to before (see text); the calibration values (in mm) were obtained using an approximate calibration of head position measurement with one of the head coil positions (Methods). Error bars denote 95% confidence intervals.
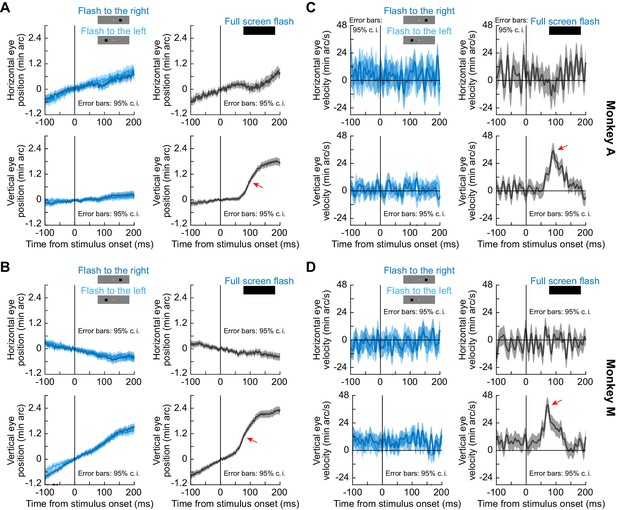
Small localized stimulus onsets had minimal effects on ocular position drifts when compared to larger flashes.
(A, B) Horizontal (top row in each panel) and vertical (bottom row in each panel) eye position in each of two monkeys (A and M) under different conditions. The left column in each panel shows data when small localized flashes were presented in the black fixation flash paradigm (Methods; similar results were obtained with the white fixation flash paradigm). The right column shows the full-screen flash results of Figure 2 for comparison. All other conventions are like in Figure 2. As can be seen, the upward drift response with full-screen flashes was much bigger than any responses to small localized stimuli. Consistent with this, there was no single contiguous interval longer than 8 ms in duration for which horizontal or vertical eye position differed between whether a localized flash was presented to the right or to the left of fixation (pink intervals on the x-axis of the bottom left panel of B), denoting when the 95% confidence intervals did not overlap with each other between the two compared curves). (C, D) Eye velocity measurements for the same data as in (A), (B). There were no time intervals during which the right and left flash eye velocity curves differed from each other (that is, there were no pink intervals on x-axes denoting a lack of overlap between 95% confidence intervals). We also confirmed that in the interval of 50–140 ms after stimulus onset, peak vertical eye velocity was not different between rightward and leftward localized flashes (p=0.64, Z = 0.47 for monkey A and p=0.92, Z = −0.10 for monkey M; Wilcoxon rank sum test). A similar conclusion was also reached for peak horizontal eye velocity (p=0.5, Z = −0.68 for monkey A and p=0.52, Z = −0.65 for monkey M). Error bars in all panels denote 95% confidence intervals.
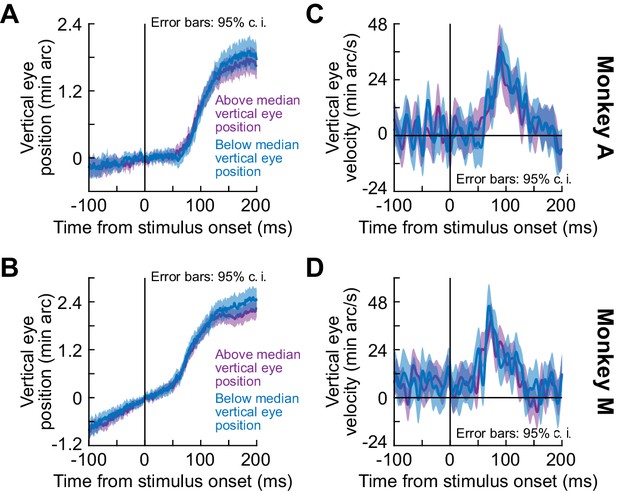
Independence of the ocular position drift response from starting eye position.
For each trial of the black fixation flash paradigm (full-screen flashes), we measured average eye position in the final 50 ms before stimulus onset. Across trials within a given session, this resulted in a population of starting eye positions. We split the trials within a session based on the median value of starting vertical eye position. We then measured the ocular position drift response for all sessions when only taking the ‘above’ median group of the sessions (purple) and again when taking only the ‘below’ median group of all sessions (bluish). (A, B) Position measurements in two monkeys. We vertically aligned traces (based on starting position at stimulus onset) in order to highlight the relative change in eye position associated with the ocular position drift response (similar to how we analyzed data in all other figures; e.g. Figure 1). (C, D) Eye velocity measurements. In both eye position and eye velocity, the ocular drift response measurements were statistically very similar; there were no time intervals in which the 95% confidence intervals between the ‘above’ and ‘below’ median groups did not overlap with each other. We also confirmed that in the interval of 50–140 ms after stimulus onset, peak vertical eye velocity was not different between the two groups (p=0.63, Z = 0.48 for monkey A and p=0.46, Z = 0.74 for monkey M; Wilcoxon rank sum test). All other conventions are similar to our other analyses of the ocular position drift response (e.g. Figure 1). Similar results were obtained with the split view stimulus and white fixation flash paradigms (e.g. p=0.32, Z = −1.01, and p=0.62, Z = −0.5 for monkeys A and M, respectively, in the white fixation flash paradigm; similarly, p>0.15, Z = −1.46,–0.82, and p>0.2, Z = −0.55, 1.27, and p>0.85, Z = 0.01, 0.19 for monkeys A, M, and N, respectively, in the split view stimulus paradigm; Z-values are reported for right and left darkness for each monkey in the split view stimulus paradigm).
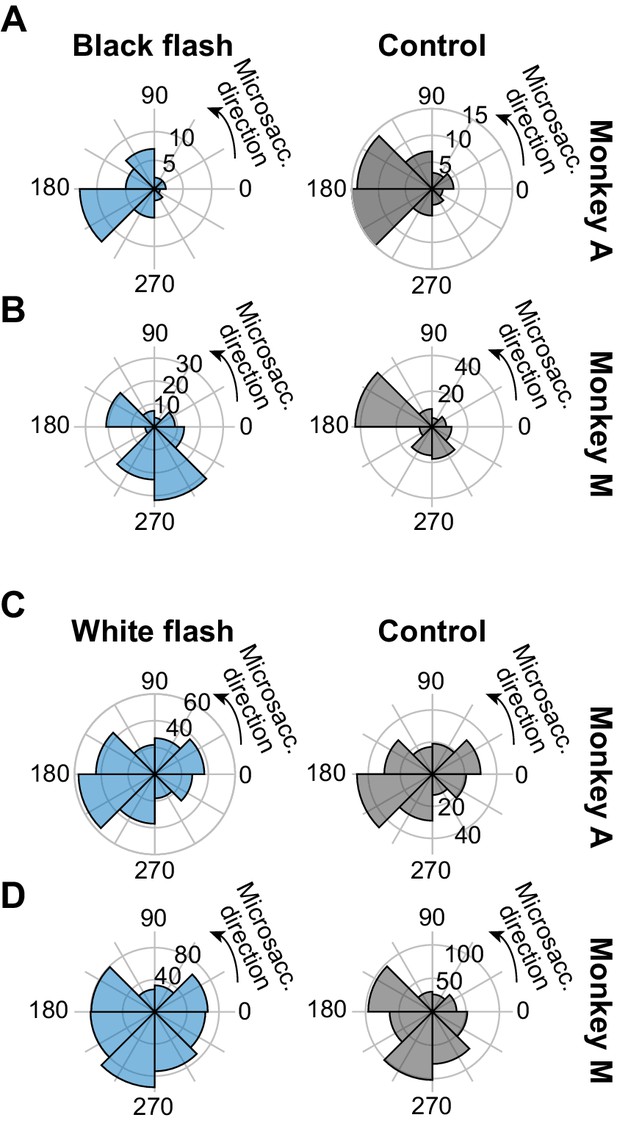
The drift response was not systematically related to the direction of the first microsaccade to occur after it.
We searched for the first microsaccade to occur after our standard designated saccade-free fixation interval of −100 ms to 200 ms relative to full-screen flash onset. Across trials, we then plotted the direction distribution of these first microsaccades. Each row shows the stimulus onset condition (left column) and the corresponding control condition without any full-screen stimulus flash (right column). (A, B) Black fixation flash paradigm. (C, D) White fixation flash paradigm. Similar results were obtained with the split view stimulus paradigm. In all cases, each monkey showed a stereotypical distribution of microsaccade directions in the control condition without any stimulus onset (e.g. predominantly leftward in the monkey A control data; right column). This distribution was largely unaltered after the upward ocular position drift response, despite some idiosyncratic variations in the ranges of microsaccade directions that occurred. This is expected: given how small the ocular position drift response is (e.g. Figure 1), its amplitude is much smaller than the amplitude of microsaccades, and it is therefore unlikely to trigger a corrective downward microsaccade.
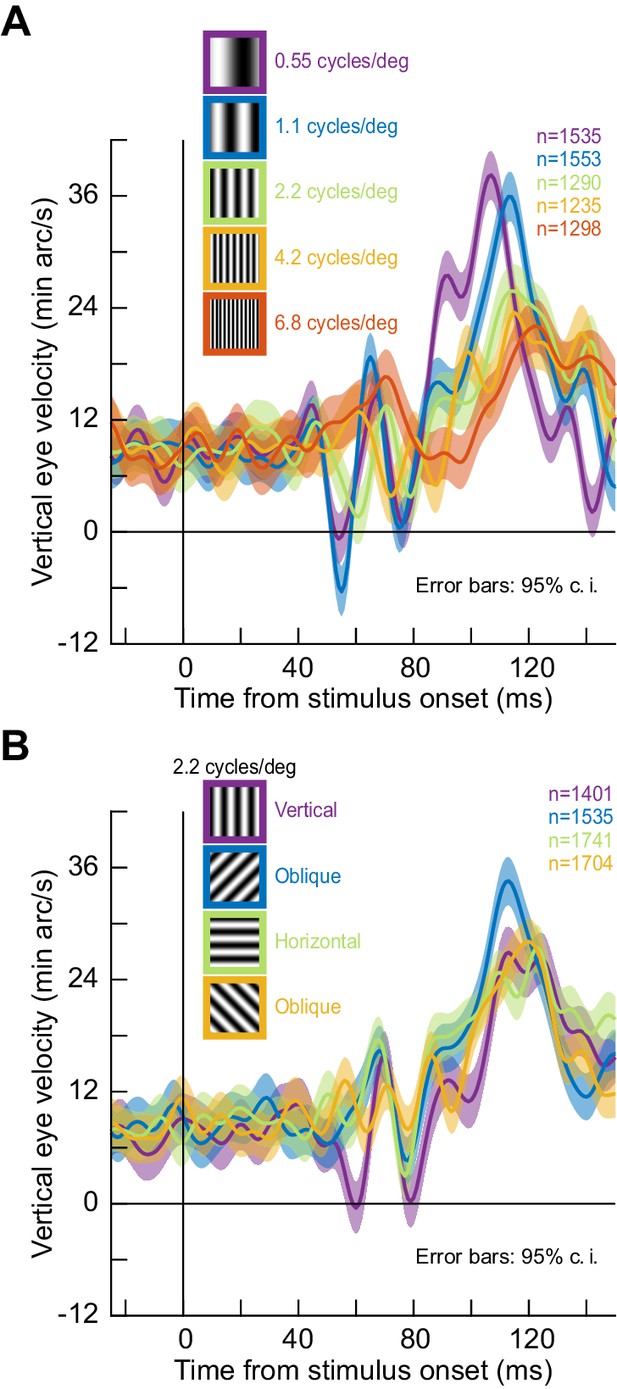
Stimulus tuning of the ocular position drift response.
(A) We replaced the full-screen flash of Figure 2 with a vertical grating of different spatial frequencies (Methods). The upward velocity pulse depended on spatial frequency; it occurred earlier, and had stronger amplitude, for low spatial frequencies. This is reminiscent of visual neural activity in the superior colliculus, an important oculomotor control circuit (Chen et al., 2018). (B) Similarly, when we fixed spatial frequency and altered grating orientation, the drift response was also modulated. Ocular position drifts are therefore sensitive to stimulus manipulations. Error bars denote 95% confidence intervals.
-
Figure 3—source data 1
Excel table with the source data for this figure.
- https://cdn.elifesciences.org/articles/57595/elife-57595-fig3-data1-v2.xlsx
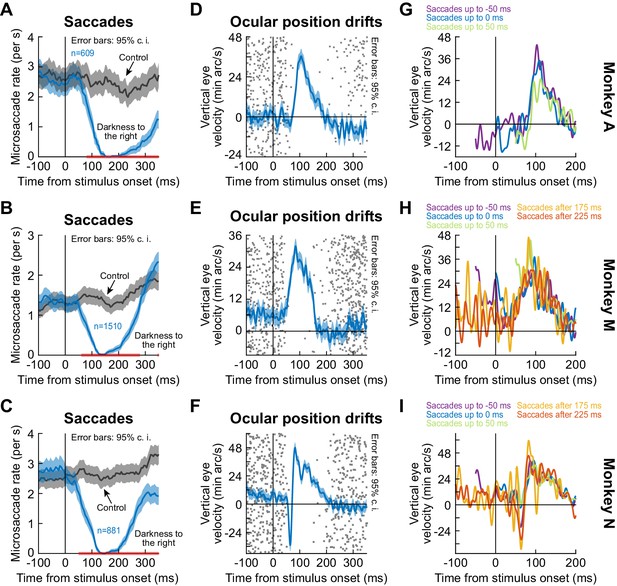
The drift response starts when saccades are inhibited.
(A–C) Our monkeys exhibited known saccadic inhibition (Buonocore et al., 2017a; Buonocore and McIntosh, 2008; Edelman and Xu, 2009; Hafed and Ignashchenkova, 2013; Hafed et al., 2011; Reingold and Stampe, 2002) after stimulus onset (split view condition shown; Figure 4—figure supplement 1 shows the fixation flash condition). Error bars denote 95% confidence intervals. (D–F) In the same sessions, saccade-free fixation epochs (no saccades from −100 to 350 ms) showed the ocular position drift response (error bars denote 95% confidence intervals). The timing of this drift response was linked to the timing of saccadic inhibition (individual dots show individual trial rasters of microsaccade onset times from A–C). The drift response started when microsaccades were inhibited. (G–I) When we combined (A–C) and (D–F), we found that even when microsaccades occurred near the time of drift response (i.e. before or after with shorter saccade-free epochs than in D–F), the drift response could still occur. In (G), two curves are missing because they had <10 trials due to the prolonged saccadic inhibition profile in (A). The different colors show traces in which there were microsaccades within a given time range relative to stimulus onset. For example, ‘Saccades up to −50 ms’ means that the curve shows average smooth eye velocity from −50 ms onwards, since earlier times had saccadic velocities in them. Similarly, the curve for ‘Saccades after 175 ms’ would mean that average eye velocity is shown up to 175 ms, since later times relative to stimulus onset had saccadic velocities in them. Error bars in (D–F) denote 95% confidence intervals. Also see Figure 4—figure supplement 1 and Figure 2—figure supplements 3 and 4.
-
Figure 4—source data 1
Excel table with the source data for this figure.
- https://cdn.elifesciences.org/articles/57595/elife-57595-fig4-data1-v2.xlsx
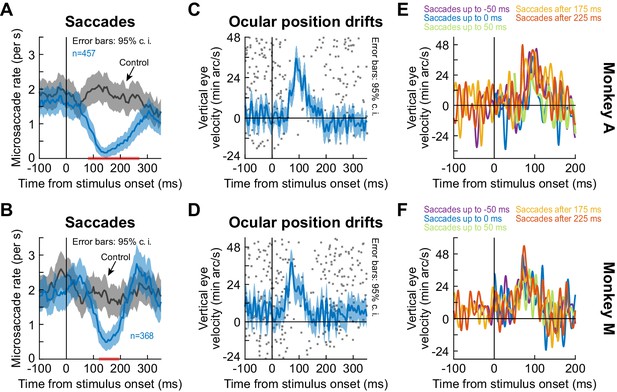
The ocular position drift response occurred during saccadic inhibition, and it also occurred even with nearby saccades.
This figure is formatted identically to Figure 4. It shows similar results, but this time from the black fixation flash paradigm using full-screen flashes (the white fixation flash paradigm produced similar observations). (A, B) Microsaccade rate after full-screen flash onset in each monkey. The gray curve shows baseline microsaccade rate with no stimulus transient. (C, D) Ocular position drift response in each monkey during saccade-free fixation. To illustrate the timing of the drift response to saccadic inhibition, the dots indicate trial-by-trial rasters of microsaccade onset times during fixation. The drift response occurred when microsaccades were inhibited. (E, F) In each monkey, we inspected the ocular drift response when microsaccades still occurred near stimulus onset (different colors denote different microsaccade times as per the legends). The drift response still occurred, as in Figure 4 with another paradigm and the inclusion of a third monkey. Error bars are defined in the respective panels. All other conventions are similar to Figure 4.