Kv1.1 channels regulate early postnatal neurogenesis in mouse hippocampus via the TrkB signaling pathway
Figures
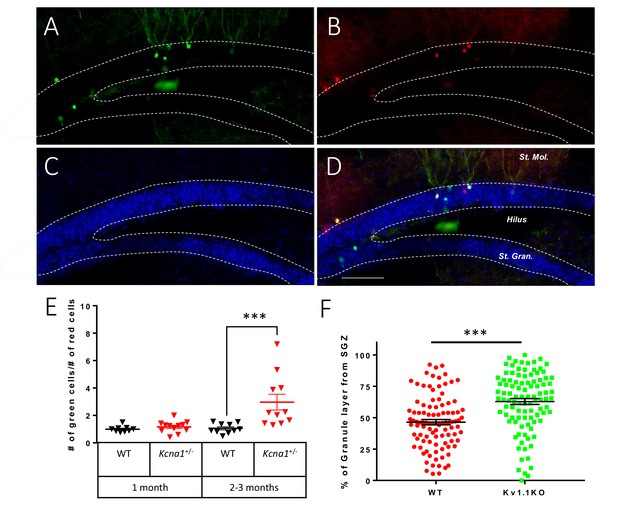
Kv1.1 channels control dentate gyrus neuron number in a cell-autonomous manner.
Mosaic analysis with double markers (MADM) of the dentate gyrus in wild-type or Kcna1+/- mice. Kv1.1KO and wild-type neurons can be respectively identified as green (GFP) (A) and red (TdTomato) (B) neurons. (C) 4′,6-diamidino-2-phenylindole (DAPI) counterstain. (D) Overlay of signals in (A) and (B), scale bar = 100 µm. (E) Statistical analysis of the red wild-type and green Kv1.1KO neurons in the dentate gyrus. The numbers of red wild-type and green Kv1.1KO neurons were comparable in the dentate gyrus of 1-month-old mice; however, compared to the numbers of red wild-type neurons, the numbers of green Kv1.1KO neurons were much higher in the dentate gyrus of 2- to 3-month-old mice (two-way ANOVA followed by Sidak's multiple comparisons test; p=0.0008, for 2- to 3-month-old mice). n = 9–12 for each group. (F) Compared to the red wild-type neurons, green Kv1.1KO neurons were more often positioned farther away from the sub-granular zone (SGZ) in 2- to 3-month-old mice, indicating that the green Kv1.1KO neurons were born in the postnatal period (p=0.0002, Mann-Whitney U-test). n = 100 for each group.
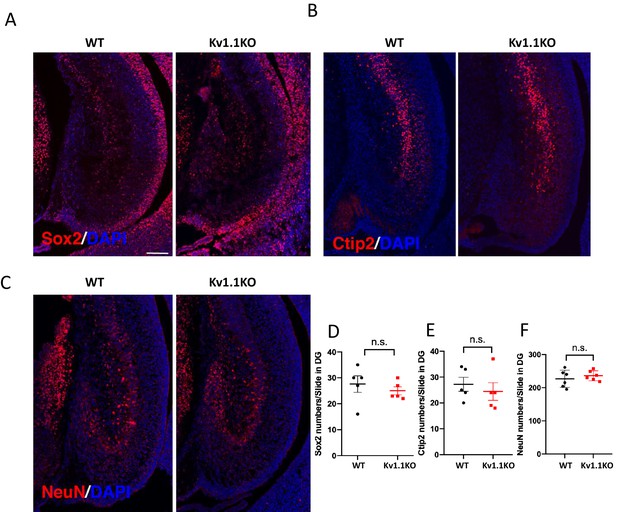
Kv1.1 channels do not affect embryonic hippocampal neurogenesis.
Immunostaining indicated that there was no difference in dentate gyrus (DG) between wild-type and Kv1.1KO mice, in terms of Sox2+ progenitors (A, D) (p=0.4807, t-test), Ctip2+ postmitotic marker (B, E) (p=0.5365, t-test), or NeuN+ neurons (C, F) (p=0.4606, t-test) at E16.5. n = 5–6 for each genotype. Scale bar = 100 µm. Data are presented as mean ± SEM. n.s., no significant difference.
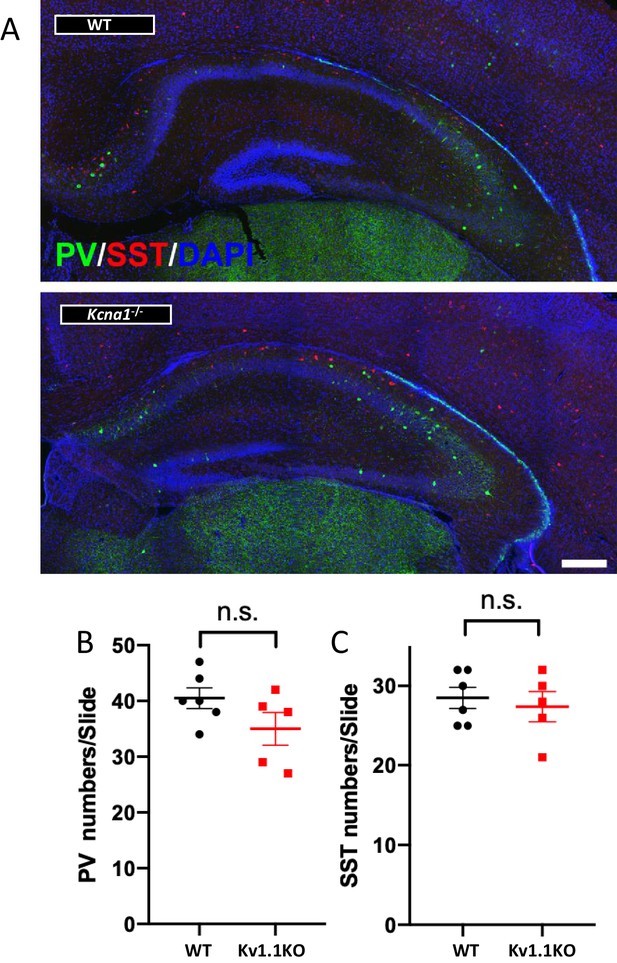
No detectable difference in interneurons of Kv1.1KO mice at P10.
(A) Immunostaining and quantification showed no significant difference in hippocampus between wild-type and Kv1.1KO mice, in terms of PV+ interneurons (B) (p=0.1363, t-test) or SST+ interneurons (C) (p=0.6372, t-test). n = 5–6 for each genotype. Scale bar = 250 µm. Data are presented as mean ± SEM. n.s., no significant difference.
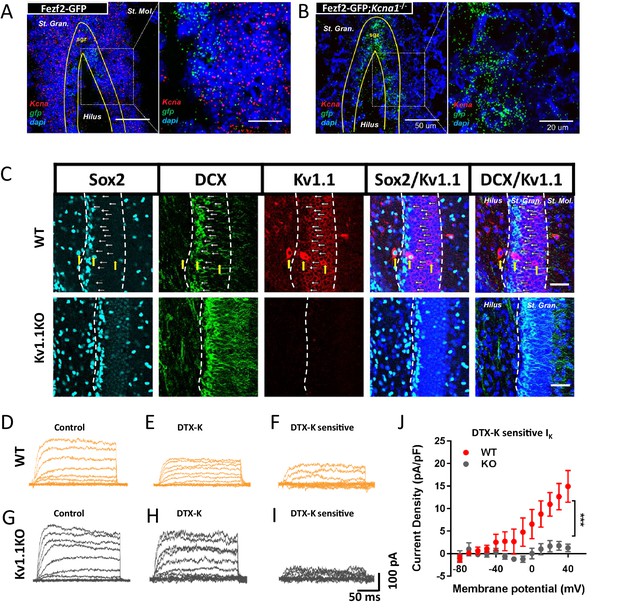
Functional expression of Kv1.1 channel in SGZ neural progenitor cells expressing Fezf2-GFP at postnatal 2 weeks.
(A) In situ hybridization results showed that Kcna1 mRNA is expressed in Fezf2-GFP-positive neural progenitor cells. Inset is displayed at a higher magnification. (B) Kcna1 mRNA was not detected in the Kv1.1KO mouse, which served as a negative control. (C) Kv1.1 protein was expressed in the doublecortin (DCX)-expressing late-stage neural progenitor cells (arrows) but not Sox2-positive early-stage neural progenitor cells. Kv1.1 protein was highly expressed in the inhibitory interneurons (yellow arrows). (D–J) Pharmacological isolation of Kv1 currents in Fezf2-GFP-positive cells. Kv1.1 currents were elicited by trains of voltage steps from -80 mV to +40 mV in 10 mV increments from the holding potential of -80 mV in the absence (D and G) or presence of the Kv1-specific blocker dendrotoxin-k (DTX-K; 100 nM) (E and H). The DTX-K-sensitive currents were considered Kv1-mediated potassium currents (F, I, and J), which were much reduced in the Kv1.1KO mice. n = 4 cells from each phenotype. Scale bar = 20 μm in (C). Data are presented as mean ± SEM.
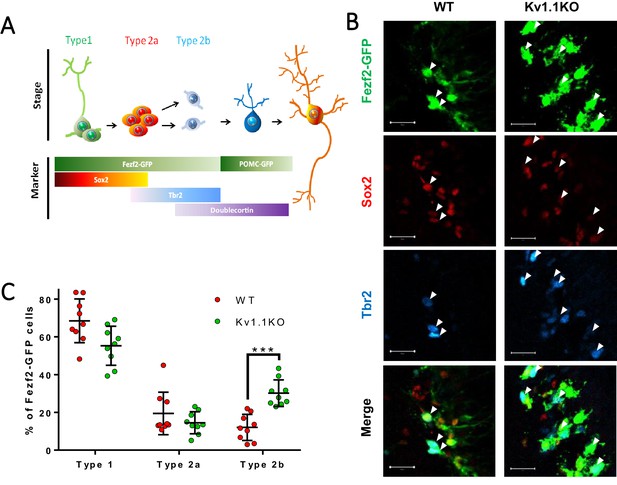
Loss of Kv1.1 channels depolarizes type 2b neural progenitor cells in SGZ at postnatal 2 weeks.
(A) Based on the expression of the cell-fate markers, the neural progenitor cells in the sub-granular zone (SGZ) can be categorized into several developmental stages (see Figure 4 for detailed images). (B) Among all the Fezf2-GFP-positive cells in the SGZ, radial glia-like type 1 neural progenitor cells expressed predominantly Sox2, type 2a neural progenitor cells expressed both Sox2 and Tbr2 (white arrows), and type 2b progenitor cells expressed Tbr2. Post-mitotic immature neurons expressed doublecortin (DCX) and could also be identified as POMC-GFP-positive cells. (C) Among these cell types, type 2b cells were significantly increased in adult Kv1.1KO dentate gyrus compared to wild type (WT), according to the numbers of Tbr2-positive Fezf2-GFP-positive cells (n = 9 for each group; two-way ANOVA followed by Sidak's multiple comparisons test; p=0.0009, for type 2b cells). Scale bar = 20 μm.
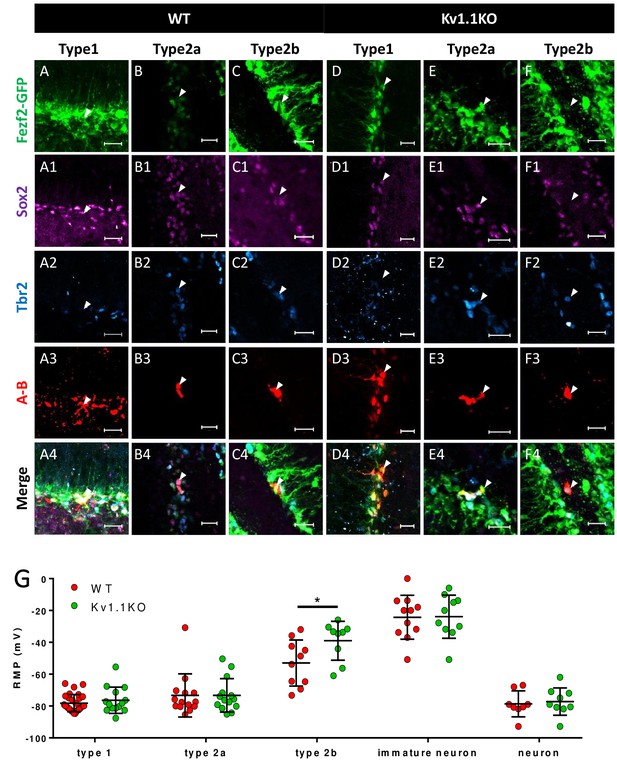
Classification of Fezf2-GFP progenitor cells in SGZ.
Based on the expression of the cell-fate markers, neural progenitor cells in the sub-granular zone (SGZ) can be categorized into several developmental stages. Among all the Fezf2-GFP-positive cells (A–F), Sox2-positive type 1 cells (A1–F1) are radial glia-like cells (A–A4, C–C4) and can give rise to Sox2+/Tbr2+ type 2a transit-amplifying progenitor cells (B–B4, D–D4). Type 2a cells further differentiate into type 2b neuroblasts that are positive for Tbr2 only (C–C4, F–F4). Type 1 (A3 and D3) and type 2a (B3 and E3) cells formed extensive syncytial connections with other cells from the same developmental stage, as indicated by the gap-junction-permeable avidin-neurobiotin (A–B) staining. Scale bar = 20 μm. (G) Type 1 and type 2a Fezf2-GFP-positive neural progenitor cells were hyperpolarized; by contrast, type 2b cells lacking Kv1.1 channels were significantly more depolarized than the wild-type (WT) cells (n = 14, 14, 9, 10, 9 (WT) and 28, 14, 10, 11, 8 (Kv1.1KO) for type 1 cells (Fezf2-GFP+/Sox2+), type 2a cells (Fezf2-GFP+/Sox2+/Tbr2+), type 2b cells (Fezf2-GFP+/Tbr2+), immature neurons (POMC-GFP+), and label-free mature neurons; (two-way ANOVA followed by Sidak's multiple comparisons test; p=0.02, for type 2b cells)).
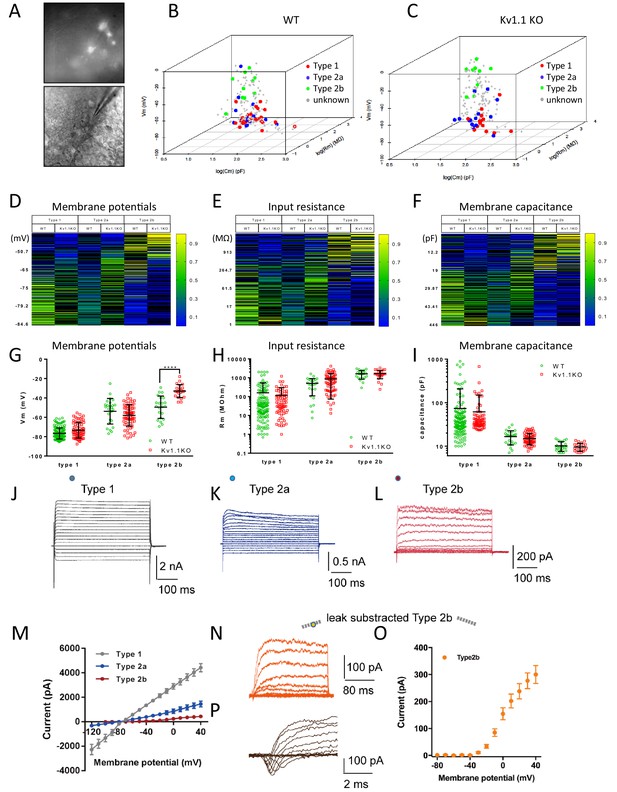
Multinomial logistic regression for cell-type prediction.
(A) Acute brain slice recording from the DG of an Fezf2-GFP mouse. (B and C) A multinomial logistic regression model was constructed using the biophysical characteristics of cells that were identified previously (same cohort of cells from Figure 3D) as the training dataset. Two individual regression models were generated for wild-type (B) and Kv1.1KO (C) cells. The cell types of unknown cells were later classified according to the resting membrane potential, input resistance, and membrane capacitance. Heatmaps show the estimated probabilities for each cell type against membrane potential (D), input resistance (E), and membrane capacitance (D). The cell types were determined based on maximum likelihood. (G) Resting membrane potentials of wild-type and Kv1.1KO progenitor cells. The predicted type 2b cells lacking Kv1.1 channels were significantly more depolarized (two-way ANOVA, followed by Sidak's multiple comparisons test; p<0.0001, for type 2b cells). Comparable input resistances (H) and membrane capacitances (I) were observed between wild-type and Kv1.1KO cells. n = 144, 25, and 22 for type 1, 2a, and 2b cells, respectively, in the wild-type group; n = 71, 70, and 22 for type 1, 2a, and 2b cells, respectively, in the Kv1.1KO group. (J–L) Voltage responses from type 1 (J), type 2a (K), type 2b (L) to series voltage from -120 mV to +40 mV or -80 mV to +40 mV, with a holding potential of -80 mV. (M) Current–voltage curves in (J–L) (the circles indicate the positions of measurements; type 1 (7 cells), type 2a (7 cells), type 2b (10 cells)). (N, O) Voltage response from -80 mV to +40 mV, with a holding potential of -80 mV and leak subtraction, shown for type 2b (N); current–voltage curve of type 2b (n = 17 cells) with leak subtraction (O). (P) A representative transient inward current (sodium current) was observed in 9 out of 25 type 2b cells. Data are presented as mean ± SD in (G), (H), and (I); mean ± SEM in (M, O).
-
Figure 5—source data 1
multiple logistic regression datasets for biophysical analysis of the neural progenitor cells in postnatal mouse hippocampus.
- https://cdn.elifesciences.org/articles/58779/elife-58779-fig5-data1-v3.xlsx
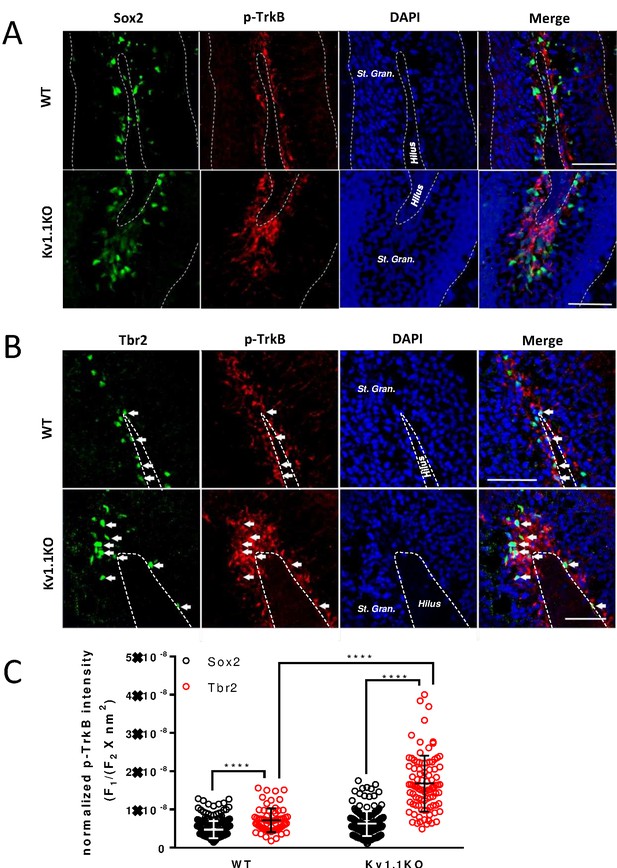
TrkB is mostly active in Tbr2-positive neural progenitor cells.
Phospho-TrkB (Tyr816) signal represents a surrogate measure for TrkB activity. Phospho-TrkB-positive cells rarely colocalized with the Sox2-positive, presumably type 1 and type 2b neural progenitor cells (A); by contrast, phospho-TrkB-positive cells colocalized with the majority of Tbr2-positive, presumably type 2b cells. (B) More Tbr2+/phospho-TrkB+ neural progenitor cells could be found in the sub-granular zone (SGZ) of Kv1.1KO mice. Scale bar = 100 μm (A) and 50 μm (B). (C) Quantification analysis of phospho-TrkB levels in Sox2+ or Tbr2+ cells. The phospho-TrkB fluorescent intensity (F1) of each Sox2+ or Tbr2+ cell was measured and normalized to the Sox2+ or Tbr2+ fluorescent intensity and the total measured cell area (F2 X nm2). n = 246, 243, 69, and 92 for Sox2+/WT, Sox2+/Kv1.1KO, Tbr2+/WT, and Tbr2+/Kv1.1KO cells, respectively. **** p<0.0001.
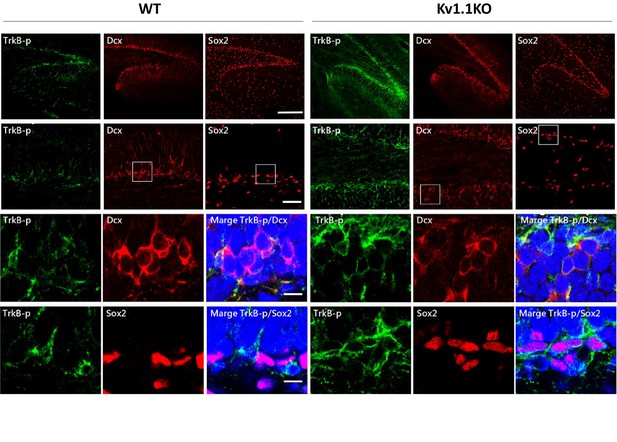
The TrkB signaling is active in DCX+ late-stage progenitor cells but not Sox2+ early-stage progenitor cells.
Phospho-TrkB-positive cells rarely colocalized with the Sox2-positive, presumably type 1 and type 2b neural progenitor cells but mostly colocalized with the DCX+ late-stage progenitor cells. Scale bar = 50 µm.
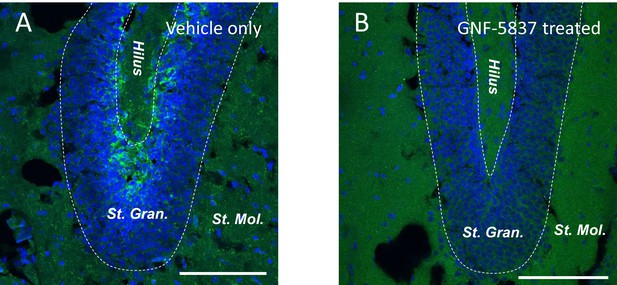
Intraperitoneal injection of GNF-5837 remarkably inhibits the TrkB signaling in SGZ.
Immunostaining of the dentate gyrus from mice treated with daily intraperitoneal vehicle-only (A) or GNF-5837 (20 mg/kg) (B) injection for 3 weeks. phospho-TrkB signal (green) can be detected in the sub-granular zone (SGZ) region in control (A) but not GNF-5837 (B). Scale bar = 100 µm.
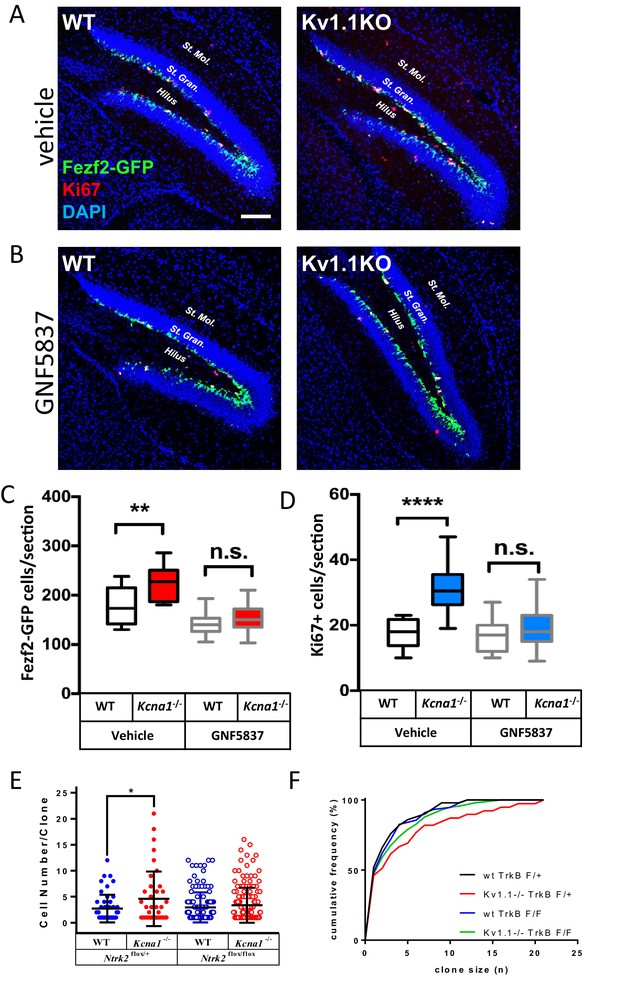
Kv1.1 channels regulate neural progenitor cell numbers via the TrkB signaling pathway.
(A, C, and D) Kv1.1KO mice have more Fezf2-GFP- (C) and Ki67-positive cells (D) than wild-type mice, with vehicle treatment. p=0.0014, one-way ANOVA followed by Tukey's test in (C); p<0.0001, one-way ANOVA followed by Tukey's test in (D). (B, C, and D) Suppression of Kv1.1-dependent neurogenesis by GNF-5837, a potent Trk inhibitor. Mice receiving daily GNF-5837 (20 mg/kg) administration had comparable numbers of Fezf2-GFP- (C) and Ki67-positive cells (D) between wild-type and Kv1.1KO mice; n = 6–8 mice from each genotype with vehicle-only or GNF-5837 treatment. (E) Clonal analysis of adult neurogenesis. Tamoxifen (0.5 mg) was administered at postnatal 3 weeks to sparsely delete Ntrk2 (a gene that encodes the TrkB receptor) in a subset of neural progenitor cells. In cells lacking only one allele of TrkB, Kv1.1KO had larger clone sizes than wild-type cells; by contrast, the Kv1.1-dependent proliferation advantage was abrogated in clones lacking both TrkB alleles. p=0.0389, comparing wild-type and Kv1.1KO on the Ntrk2flox/+ background; two-way ANOVA followed by Sidak's multiple comparisons test; n = 39–157 for each group. Scale bar = 100 μm. Data are presented as mean ± SEM. *p<0.05, **p<0.01; ***p<0.001; ****p<0.0001; n.s., no significant difference. (F) Kolmogorov-Smirnov plots showing a rightward shift of clone sizes in Kcna1-/-;Gli1creERT2/+;Ntrk2flox/+;Rosa26Tom/+ mice (red trace) compared to wild-type Gli1creERT2/+;Ntrk2flox/+;Rosa26Tom/+ mice (black trace) (p=0.0029, t-test), but this effect was absent in mice lacking TrkB receptor (p>0.05 between wild-type Gli1creERT2/+;Ntrk2flox/+;Rosa26Tom/+ (black trace) and Gli1creERT2/+;Ntrk2flox/flox;Rosa26Tom/+ (blue trace); Kcna1-/-;Gli1creERT2/+;Ntrk2flox/flox;Rosa26Tom/+ (green trace)).
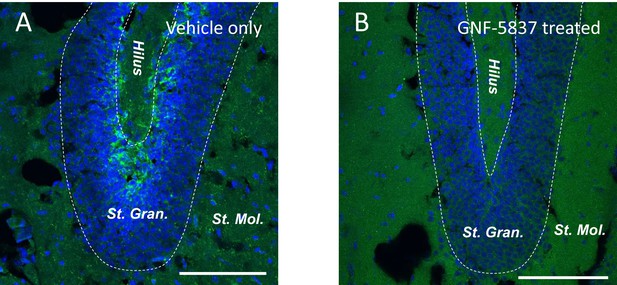
Intraperitoneal injection of GNF-5837 remarkably inhibits the TrkB signaling in SGZ.
Immunostaining of the dentate gyrus from mice treated with daily intraperitoneal vehicle-only (A) or GNF-5837 (20 mg/kg) (B) injection for 3 weeks. phospho-TrkB signal (green) can be detected in the sub-granular zone (SGZ) region in control (A) but not GNF-5837 (B). Scale bar = 100 µm.
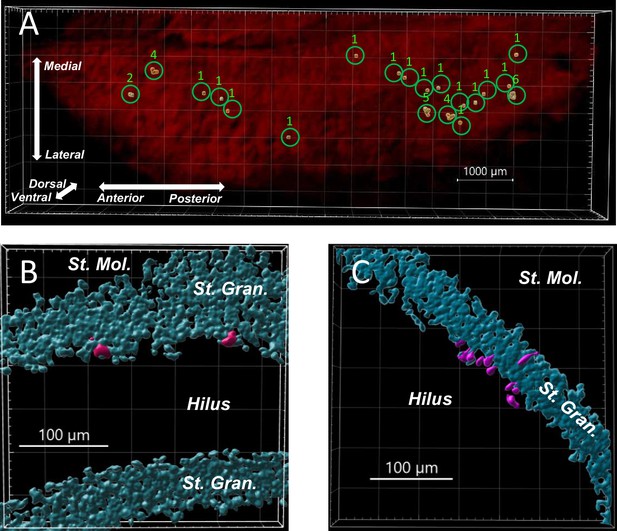
Clonal analysis of postnatal born neurons in the SGZ.
Single low-dose tamoxifen (0.5 mg/kg) was injected into a 3-week-old Gli1creERT2/+;Rosa26Tom/+ mouse and later sacrificed at 8 weeks of age. The clonal clusters were defined as the TdTomato+ cells contained within 100-μm radius of the clone center. (A) An overview of a whole-mount 3D hippocampus (red) from a Gli1creERT2/+;Ntrk2flox/flox;Rosa26Tom/+ mouse. The neural progenitor cells were identified automatically by the software, and the clone sizes were labeled as the number above the green circles. (B) A higher magnification of two single-cell clones that were separated by more than 150 µm. (C) A group of cells that are clustered within a radius of 45 µm are considered as a single clone with 10 cells. Both (B) and (C) were obtained from the hippocampus of a Kcna1-/-; Gli1creERT2/+;Ntrk2flox/+;Rosa26Tom/+ mouse.
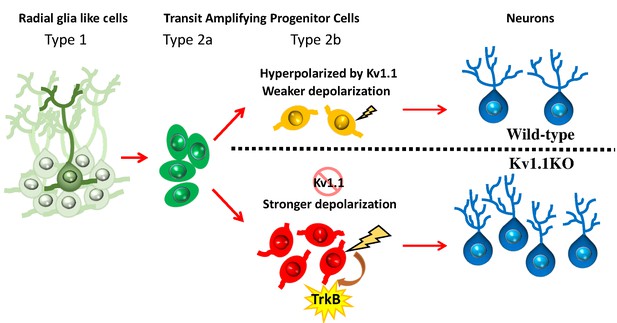
Summary of the role of Kv1.1 channels in regulating early postnatal neurogenesis in SGZ.
The membrane potentials in type 1 radial glia-like neural stem cells and type 2a transit-amplifying progenitor cells are relatively hyperpolarized. In type 2b neural progenitors, cells lacking the Kv1.1 channel are more depolarized than wild types, further stimulating the proliferation of type 2b cells via activating the TrkB signaling pathway. The mature granule cells become hyperpolarized again.
Tables
Reagent type (species) or resource | Designation | Source or reference | Identifiers | Additional information |
---|---|---|---|---|
Strain background (Mus musculus) | ICR (CD-1 or Swiss Outbred) | BioLASCO Taiwan | Outbred strain | |
Genetic reagent (Mus musculus) | Kcna1- (Kcna1tm1Tem) | PMID:9581771 | MGI: 1861959 | Dr. Bruce Tempel (University of Washington, USA) |
Genetic reagent (Mus musculus) | Rosa26GT (Gt(ROSA)26Sortm6(ACTB-EGFP*,-tdTomato)) | PMID:22479386 | MGI: 5314252 | Dr. Liqun Luo (Stanford University, USA) |
Genetic reagent (Mus musculus) | Rosa26TG (Gt(ROSA)26Sortm7(ACTB-EGFP*)) | PMID:22479386 | MGI: 5314253 | Dr. Liqun Luo (Stanford University, USA) |
Genetic reagent (Mus musculus) | POMC-GFP (Tg(Pomc-EGFP)1) | PMID:11373681 | MGI: 3776091 | Dr. Jeffery Friedman (Rockefeller University, USA) |
Genetic reagent (Mus musculus) | Fezf2-GFP (Tg(Fezf2-EGFP)CO61Gsat) | PMID:14586460 | MGI: 3847288 | Dr. Su Guo (University of California, San Francisco, USA) |
Genetic reagent (Mus musculus) | Ntrk2flox (Ntrk2tm1Lfr) | PMID:10995833 | MGI: 2384391 | Dr. Louis Reichardt (University of California, San Francisco, USA) |
Genetic reagent (Mus musculus) | Nestin-cre (Tg(Nes-cre)1Kln) | PMID:10471508 | MGI: 2176173 | Jackson Laboratory, USA |
Genetic reagent (Mus musculus) | Gli1creERT2 (Gli1tm3(cre/ERT2)Alj) | PMID:15315762 | MGI: 3053957 | Jackson Laboratory, USA |
Genetic reagent (Mus musculus) | Rosa26Tom (Gt(ROSA)26Sortm14(CAG-tdTomato)Hze) | PMID:20023653 | MGI: 3809524 | Jackson Laboratory, USA |
Antibody | Anti-GFP (chicken polyclonal) | Aves, USA | Cat#: GFP-1010 RRID:AB_2307313 | 1:400 |
Antibody | Anti-Sox2 (mouse monoclonal, IgG2b) | Millipore, USA | Cat#: MAB4343 RRID:AB_11205572 | 1:400 |
Antibody | Anti-Tbr2 (rabbit monoclonal) | Abcam, USA | Cat#: ab183991 RRID:AB_2721040 | 1:400 |
Antibody | Anti-Tbr2 (chicken polyclonal) | Millipore, USA | Cat#: AB15894 RRID:AB_10615604 | 1:400 |
Antibody | Anti-Doublecortin (rabbit monoclonal) | Cell Signaling, USA | Cat#: 14802 | 1:400 |
Antibody | Anti-phospho-TrkB (Tyr816) (rabbit polyclonal) | Millipore, USA | Cat#: ABN1381RRID:AB_2721199 | 1:100 |
Antibody | Anti-Kv1.1 (mouse polyclonal, IgG2b) | Aves, USA | Cat#: 75–105RRID:AB_2128566 | 1:200 |
Antibody | Anti-Ki67 (rabbit polyclonal) | Invitrogen, USA | Cat#: MA5-14520RRID:AB_10979488 | 1:500 |
Commercial assay or kit | Fluoromount G | SouthernBiotech, USA | Cat#: 0100–01 RRID:SCR_015961 | |
Commercial assay or kit | RNAscope Fluorescent Multiplex Kit | Advanced Cell Diagnostics, Inc, USA | Cat#: 320850 | |
Recombinant DNA reagent | Probe-Mm-GFP | Advanced Cell Diagnostics, Inc, USA | Cat#: 400281 | |
Recombinant DNA reagent | Probe-Mm-Kcna | Advanced Cell Diagnostics, Inc, USA | Cat#: 435901 | |
Chemical compound, drug | GNF-5837 | Tocris, UK | Cat#: 4559 | |
Chemical compound, drug | PEG400 (Polyethylene glycol 400) | Sigma-Aldrich, USA | Cat#: 1546445 | |
Chemical compound, drug | Cremophor EL | Sigma-Aldrich, USA | Cat#: C5135 | |
Chemical compound, drug | Dendrotoxin-K | Alomone Labs, Israel | Cat#: D-400 | |
Chemical compound, drug | Cremophor EL | Sigma-Aldrich, USA | Cat#: C5135 | |
Chemical compound, drug | Neurobiotin | Vector Laboratories, USA | Cat#: SP-1120 | 0.3% in the pipette solution |
Chemical compound, drug | Lucifer yellow-conjugated dextrans | Invitrogen, USA | Cat#: D-1825 | 0.2% in the pipette solution |
Chemical compound, drug | Neutravidin | Thermo Fisher, USA | Cat#: 31000 | 0.25% |
Software, algorithm | pClamp 10.0 | Molecular Devices, USA | RRID:SCR_011323 | |
Software, algorithm | GraphPad Prism 7.0 | Graphpad, USA | RRID:SCR_002798 | |
Software, algorithm | Imaris 9.0 | Bitplane, Switzerland | RRID:SCR_007370 | |
Software, algorithm | R Project for Statistical Computing | The R foundation | RRID:SCR_001905 |