Parallel global profiling of plant TOR dynamics reveals a conserved role for LARP1 in translation
Figures
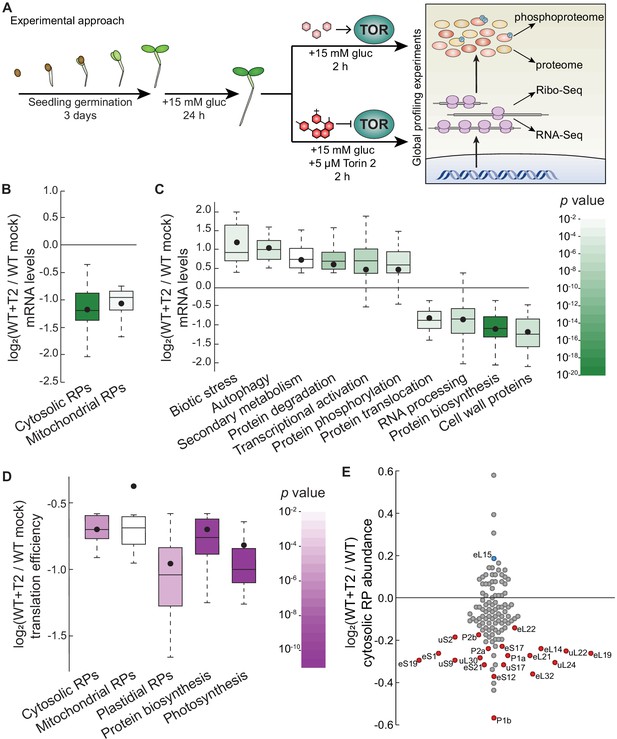
Parallel global profiling experiments demonstrate that TOR regulates ribosome biogenesis and photosynthesis at multiple levels in Arabidopsis.
(A) Arabidopsis thaliana seeds were germinated and grown to quiescence under photosynthesis-limiting conditions (Xiong et al., 2013) for three days and then supplemented with 15 mM glucose to stimulate TOR and growth for 24 hr. Seedlings were next treated with either 15 mM glucose + 5.0 μM Torin2 to attenuate TOR activity or only 15 mM glucose to promote TOR activity for 2 hr. Seedlings were then snap-frozen in liquid nitrogen. RNA or protein was extracted as described in the methods for global, unbiased profiling of the seedling Torin2-sensitive transcriptome, translatome, proteome, and phosphoproteome. (B) Inhibiting the TOR pathway affected the accumulation of transcripts in multiple categories, as determined by MapMan analysis. One of the most broadly affected categories was protein biosynthesis, including significantly lower levels of mRNAs that encode cytosolic and mitochondrial ribosomes after Torin2 treatment. Here and below, the observed fold-change in mRNA levels for each category is shown in box-whisker plots drawn with Tukey’s method; the middle line represents the median and the dot represents the mean of the fold-changes. p values for each category were determined by the Mann–Whitney U test using MapMan gene ontologies and corrected for false positives with the stringent Benjamini-Yekutieli method. (C) In addition to RP genes, categories that were affected by Torin2 treatment included repression of genes involved in protein translocation and RNA processing and induction of genes involved in protein degradation and stress responses. (D) The TE of transcripts involved in protein biosynthesis and photosynthesis were repressed in Torin two treatment. TE was determined by dividing Ribo-Seq FPKM by RNA-Seq FPKM for each gene. (E) Proteomic analysis showed that cytosolic RP levels are reduced after Torin2 treatment. The scatterplot shows all detected RPs as dots; color-coded and labeled dots represent RPs with statistically-significant changes in abundance (p<0.05, Mann–Whitney U test).
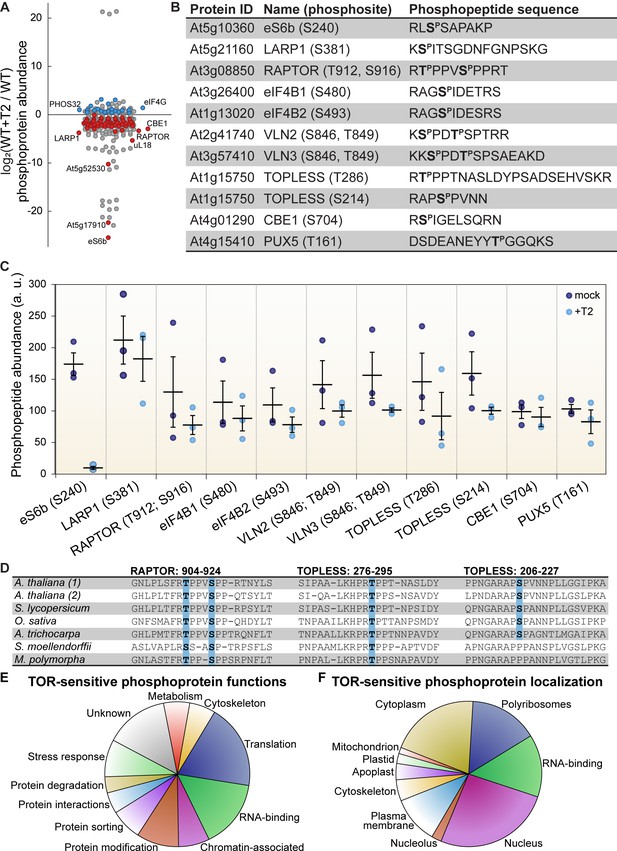
TOR regulates the phosphorylation of critical proteins involved in translation, cellular dynamics, and signal transduction in Arabidopsis seedlings.
(A) Phosphoprotein levels decreased for the majority (80%) of the 85 proteins that were significantly differentially phosphorylated after Torin2 treatment. The scatterplot displays the difference in phosphoprotein abundance in Torin2-treated seedlings for every detected phosphoprotein. Statistically-significant differences are indicated by colored dots (red for decreased abundance, blue for increased abundance; p<0.05, Mann–Whitney U test). (B) The protein ID, name, phosphosite(s), and the sequence of individual detected phosphopeptides from select proteins are shown. (C) The abundance of phosphopeptides shown in (B) in response to Torin2 are represented by dots in this scatterplot (mock-treated, dark blue; Torin2-treated, light blue). Black lines represent the mean and standard error. In each case, the difference was statistically-significant (p<0.05, t test). (D) Alignment of RAPTOR and TOPLESS protein sequences surrounding the phosphosites that were sensitive to Torin2 treatment. A. thaliana (1) shows RAPTOR1 and TOPLESS sequences; A. thaliana (2) shows the sequences of their closely-related paralogs, RAPTOR2 and TOPLESS-RELATED 1. Sequences from representative land plant species are shown in phylogenetic order: A. thaliana (thale cress, a rosid) Solanum lycopersicum (tomato, an asterid), Oryza sativa (rice, a monocot), Amborella trichocarpa (representing a basal lineage of angiosperms), Selaginella moellendorffii (spikemoss, a lycophyte), and Marchantia polymorph (common liverwort, representing a basal lineage of land plants). Phosphosites are highlighted in blue. (E) Categorical analysis of the molecular functions of Torin2-sensitive phosphoproteins is shown. (F) Categorical analysis of the subcellular localization of Torin2-sensitive phosphoproteins is shown.
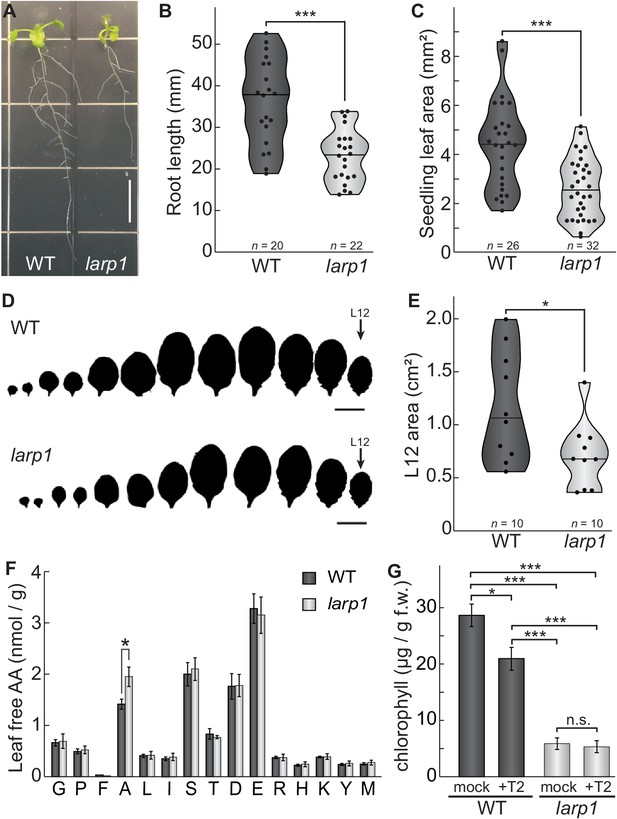
LARP1 is required for normal plant growth and physiology.
(A) Arabidopsis larp1 seedlings grown for 15 days in half-strength MS agar plates were smaller than WT seedlings (white scale bar, 1 cm), with shorter roots (B) and smaller leaf area (measuring the area of the first two true leaves) (C) in larp1 than WT seedlings. Results are represented with violin plots; the line shows the median and the dots represent each measurement (***p<0.001, t test). (D) Leaf outlines of 4-week-old WT and larp1 plants (Scale bar = 1 cm) showed that recently-emerged larp1 leaves (L12) are significantly smaller than WT, as shown with violin plots in (E). Results are represented with violin plots; the line shows the median and the dots represent each measurement (*p<0.05). (F) Alanine levels were higher in larp1 leaves than in WT. Free amino acid levels are depicted in a bar graph showing average levels in WT (dark gray) and larp1 (light gray) from four samples, with error bars representing standard error (*p<0.05). (G) Total chlorophyll levels were reduced in WT seedlings (dark gray) when treated with 5.0 μm Torin2 and were significantly lower in the larp1 background (light gray) regardless of treatment (n > 4). The bar graph shows mean total chlorophyll levels and standard error (***p<0.001, **p<0.01, *p<0.05, n.s. = not significant).
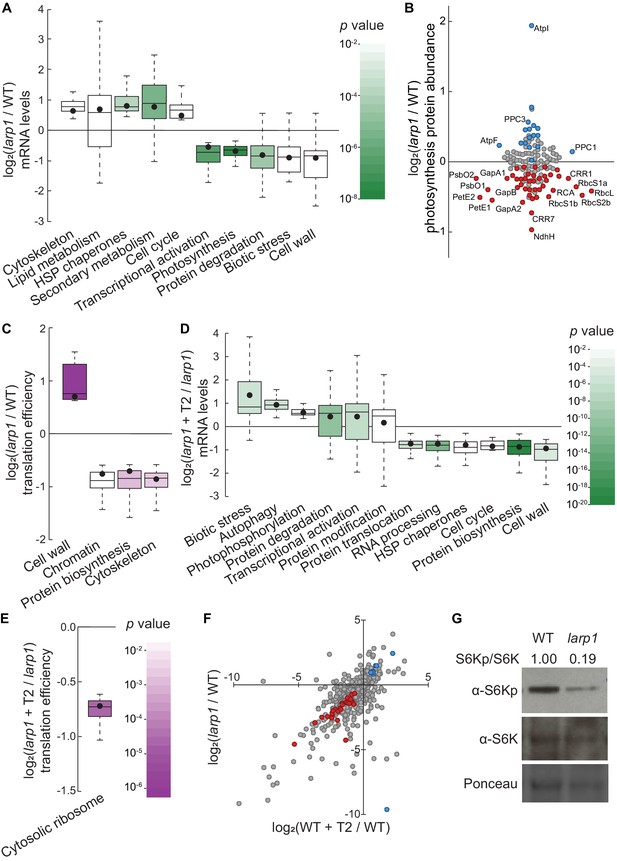
LARP1 promotes TOR signaling and activity in growing Arabidopsis seedlings.
(A) Multiple categories of transcripts accumulated to significantly different levels in larp1 compared to wild-type. Most significantly, photosynthesis-associated nuclear gene expression was strongly repressed in larp1 mutants. Here and below, the observed fold-change in mRNA levels for each category is shown in box-whisker plots drawn with Tukey’s method; the middle line represents the median and the dot represents the mean of the fold-changes. p values for each category were determined by the Mann–Whitney U test using MapMan gene ontologies and corrected for false positives with the stringent Benjamini-Yekutieli method. (B) Correlating with lower chlorophyll levels (Figure 3G), global quantitative proteomics revealed that most photosynthesis-related protein levels were lower in larp1 mutants. The color-coded dots represent proteins that were detected at significantly higher (blue) or lower (red) levels in larp1 compared to WT, with representative proteins labeled. (C) Many transcripts show differences in TE in larp1 compared to wild-type, as depicted in box-whisker plots (drawn as in A) showing significantly-affected MapMan categories with p values colored as in panel E. (D) Torin2 treatment in the larp1 background affects the mRNA levels of multiple functional categories, mostly similar to the effects of Torin2 on mRNA levels in wild-type plants (Figure 1C), including repression of protein biosynthesis, cell-cycle progression, and protein sorting, alongside induction of stress responses and protein degradation pathways. (E) The TE of cytosolic ribosomal mRNAs is significantly repressed by Torin2 treatment in larp1 mutants. (F) TOR activity is reduced in larp1 mutants. Observed differences in phosphoprotein abundance in mock-treated larp1 compared to wild-type (y axis) are mapped against Torin2-treated WT versus to mock-treated WT (x axis); phosphoproteins that accumulated to significantly different levels (p<0.05) in both comparisons are highlighted in red and blue. The strong positive correlation shown suggests that the TOR signaling network is relatively inactive in larp1 mutants than in WT. (G) Phosphorylation of the direct TOR substrate, S6K-pT449, is drastically reduced in larp1 mutants compared to WT, confirming the results shown in panel F. The densitometry ratio between the levels of S6K-pT449 and total S6K are shown above. This experiment was repeated three times with consistent results.
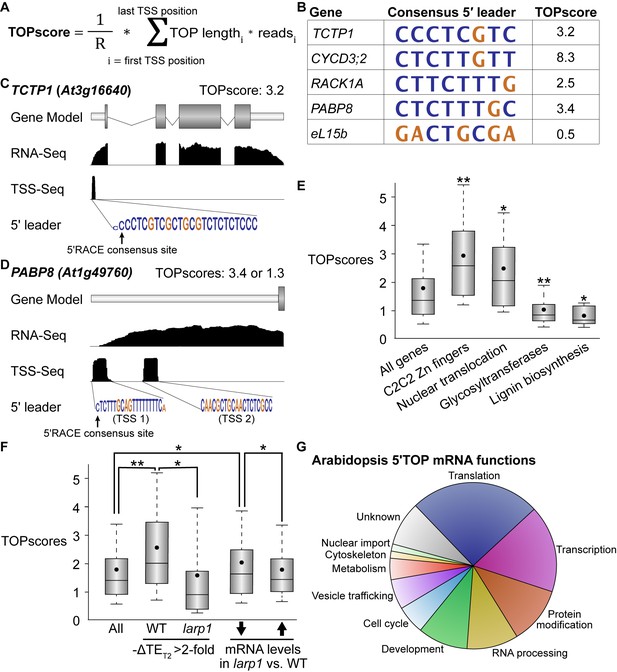
TOR-LARP1 signaling specifically controls 5′TOP mRNA translation.
(A) TOPscores were calculated by scoring the transcription start site (TSS) reads for a given gene. Briefly, for every nucleotide in the annotated 5′ leader of a gene, the number of TSS-Seq reads was multiplied by the length of the pyrimidine tract (TOP) starting at that nucleotide. The sum of these weighted reads was then divided by the total number of reads throughout the 5′ leader (R). (B) Representative examples of 5′TOP sequences in Arabidopsis. Each of these TSSs was validated with 5′RACE. TCTP1, CycD3;2, RACK1, and PABP8 are all encoded by 5′TOP mRNAs in Arabidopsis; as a counter-example, ribosomal protein eL15b is not encoded by a 5′TOP mRNA. Nucleotides are color-coded for pyrimidines (blue) or purines (gold). (C,D) TCTP1 and PABP8 are 5′TOP mRNAs in Arabidopsis. Gene models show the 5′ leader (narrow white rectangle), open reading frame (wide gray rectangles), introns (thin lines), and 3′ untranslated region. RNA-Seq and TSS-Seq read coverages are shown. The sequences of TSS-Seq peaks in the 5′ leader are shown as in (B). (E) Categorical analysis of TOPscores by MapMan revealed four functional categories with significantly different TOPscores compared to the set of all genes (p<0.05 after Benjamini-Yekutieli correction). Box-whisker plots show the distribution of TOPscores for each category. TOPscores in each category were compared using Mann–Whitney U tests; *p<0.05, **p<0.01. (F) In wild-type seedlings, TOPscores for transcripts that were translationally repressed by Torin2 treatment are significantly higher than the distribution of TOPscores transcriptome-wide and significantly higher than the TOPscores for transcripts that were translationally repressed by Torin2 treatment in larp1 mutants (Mann–Whitney U, *p<0.05, **p<0.01). There was no statistically- significant difference in TOPscore distributions between the whole transcriptome and the set of transcripts that were translationally repressed in larp1. mRNAs with significantly lower steady-state levels in larp1 mutants compared to WT also had slightly but statistically significantly higher TOPscores than the distribution of all TOPscores and the TOPscores of mRNAs with significantly higher steady-state levels in larp1 mutants compared to WT. (G) High-confidence 5′TOP mRNAs in Arabidopsis participate in diverse biological functions, including RNA metabolism, protein metabolism, cell-cycle regulation, and subcellular trafficking.
-
Figure 5—source data 1
TOR-LARP1-5′TOP signaling in Arabidopsis seedlings regulates translation of mRNAs that encode deeply conserved eukaryotic proteins, plant lineage-specific proteins, and diverse proteins involved in ribosome biogenesis.
(A) Several mRNAs are translationally regulated by TOR-LARP1-5′TOP signaling in both humans (Philippe et al., 2020) and Arabidopsis. (B) Some 5′TOP mRNAs in Arabidopsis encode proteins that evolved in the plant lineage, including genes involved in chloroplast physiology and developmental patterning. (C) Although the translation of only a few RP transcripts is directly controlled by TOR-LARP1-5′TOP signaling in Arabidopsis seedlings, multiple other steps of ribosome biogenesis are subject to TOR-LARP1-5′TOP regulation.
- https://cdn.elifesciences.org/articles/58795/elife-58795-fig5-data1-v2.xlsx
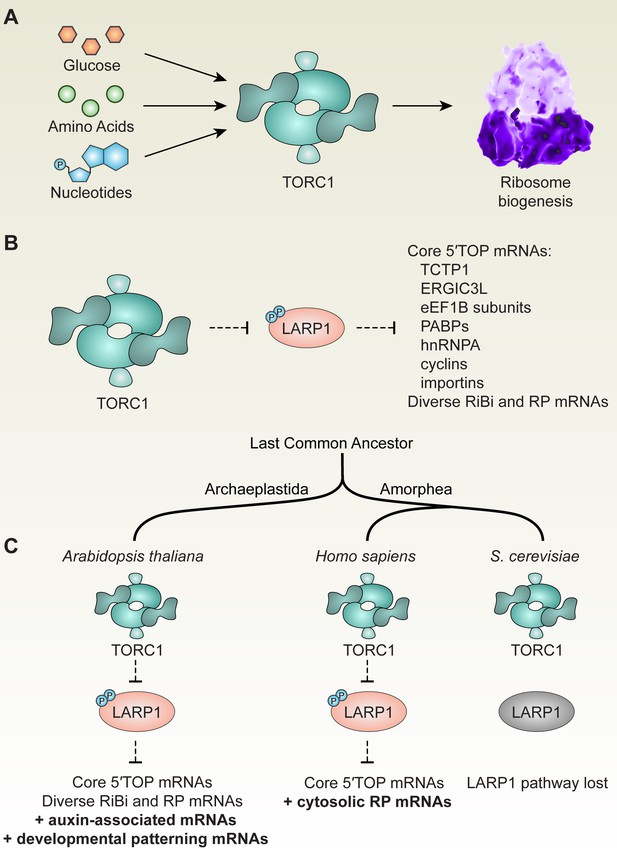
TOR-LARP1-5′TOP signaling regulates ribosome biogenesis through both conserved and evolving mechanisms in eukaryotes.
(A) Across eukaryotic lineages, TORC1 coordinates metabolism with ribosome biogenesis. When glucose (or ATP), amino acids, and nucleotides are sufficiently available, TOR promotes ribosome biogenesis at multiple levels, including transcriptional, translational, and post-translational controls. (B) Comparative global profiling in Arabidopsis and humans presented here revealed a set of ‘core’ 5′TOP mRNAs that are regulated by the TOR-LARP1-5′TOP signaling axis. (C) We propose that TOR-LARP1-5′TOP signaling evolved an early role in regulating ribosome biogenesis in the last common ancestor of plants and animals by controlling translation of ribosome biogenesis (RiBi)-associated mRNAs. Subsequently, plants exapted this signaling axis to regulate the expression of other mRNAs that encode, for example, proteins involved in hormone signaling and developmental patterning. Animal ancestors adapted the TOR-LARP1-5′TOP pathway to directly control expression of ribosomal protein mRNAs themselves, rather than diverse upstream RiBi mRNAs. Other lineages, including a recent ancestor of Saccharomyces cerevisiae, lost the LARP1 pathway, and presumably coordinate ribosome biogenesis downstream of TOR through other mechanisms.
Additional files
-
Supplementary file 1
Molecular dynamics of gene expression in Torin2-treated Arabidopsis seedlings.
Table presents all global profiling data from this study, including RNA-Seq, Ribo-Seq, proteomes, and phosphoproteomes of WT and larp1 seedlings treated with glucose (mock) or glucose and Torin2.
- https://cdn.elifesciences.org/articles/58795/elife-58795-supp1-v2.xlsx
-
Supplementary file 2
Transcriptome level changes in WT+Torin2 treated seedlings compared to WT.
- https://cdn.elifesciences.org/articles/58795/elife-58795-supp2-v2.xlsx
-
Supplementary file 3
Translational efficiency level changes in WT+Torin2 treated seedlings compared to WT.
Table presents the fold-change in translation efficiency levels of the genes from the different categories, as determined by Mapman analysis. These data are represented as a box-whisker plot in Figure 1D.
- https://cdn.elifesciences.org/articles/58795/elife-58795-supp3-v2.xlsx
-
Supplementary file 4
Proteomic analysis of cytosolic RP abundance changes and phosphoprotein abundance changes in WT+Torin2 treated seedlings compared to WT.
- https://cdn.elifesciences.org/articles/58795/elife-58795-supp4-v2.xlsx
-
Supplementary file 5
Transcriptome level changes in larp1 seedlings compared to WT.
Table presents the fold-change of mRNA levels of the genes from the different categories, as determined by Mapman analysis. These data are represented as a box-whisker plot in Figure 4A.
- https://cdn.elifesciences.org/articles/58795/elife-58795-supp5-v2.xlsx
-
Supplementary file 6
Proteomic analysis of photosynthesis protein abundance changes in larp1 seedlings compared to WT and correlation analysis of phosphoprotein abundance changes in WT+Torin2 treated seedlings compared to WT and larp1 seedlings compared to WT.
Table presents the fold-change of photosynthesis protein abundance of the genes with statistically-significant changes, the fold-change of phosphoprotein abundance with statistically-significant changes in WT+T2 compared to WT, and the fold-change of phosphoprotein abundance with statistically-significant changes in larp1 compared to WT. These data are represented as scatterplots in Figure 4B and Figure 4F.
- https://cdn.elifesciences.org/articles/58795/elife-58795-supp6-v2.xlsx
-
Supplementary file 7
Translational efficiency level changes in larp1 seedlings compared to WT.
Table presents the fold-change in translation efficiency levels of the genes from the different categories, as determined by Mapman analysis. These data are represented as a box-whisker plot in Figure 4C.
- https://cdn.elifesciences.org/articles/58795/elife-58795-supp7-v2.xlsx
-
Supplementary file 8
Transcriptome level changes in larp1+Torin2 treated seedlings compared to larp1.
Table presents the fold-change of mRNA levels of the genes from the different categories, as determined by Mapman analysis. These data are represented as a box-whisker plot in Figure 4D.
- https://cdn.elifesciences.org/articles/58795/elife-58795-supp8-v2.xlsx
-
Supplementary file 9
Translational efficiency level changes in larp1+Torin2 treated seedlings compared to larp1.
Table presents the fold-change in translation efficiency levels of the genes from the different categories, as determined by Mapman analysis. These data are represented as a box-whisker plot in Figure 4E.
- https://cdn.elifesciences.org/articles/58795/elife-58795-supp9-v2.xlsx
-
Supplementary file 10
Arabidopsis 5′TOP mRNAs.
Table presents strong candidate 5′TOP mRNAs in Arabidopsis, showing their TOPscores and fold-changes in translation efficiency in response to Torin2 in WT and larp1 seedlings.
- https://cdn.elifesciences.org/articles/58795/elife-58795-supp10-v2.xlsx
-
Transparent reporting form
- https://cdn.elifesciences.org/articles/58795/elife-58795-transrepform-v2.docx