Toxoplasma TgATG9 is critical for autophagy and long-term persistence in tissue cysts
Figures
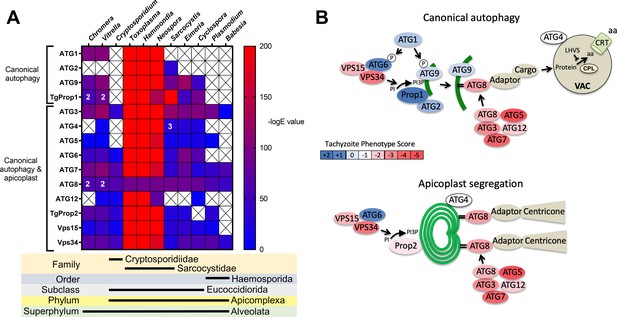
Phylogenetic conservation and adaptation of autophagy-related proteins in alveolates.
(A) Reciprocal BLAST hits to Toxoplasma gondii autophagy-related proteins detected in different alveolates. The inverse logarithm of the E value (−logE) from each hit with a corresponding T. gondii protein is plotted in a heat map to indicate the extent to which T. gondii autophagy-related proteins are conserved among other alveolates. A higher −logE value indicates a closer match to T. gondii orthologs. The number of orthologs is shown in the corresponding cell if more than one ortholog was identified. Boxes with a cross indicate no matching ortholog was identified. (B) Working models of canonical autophagy and apicoplast segregation mediated by a subset of autophagy-related proteins. Autophagy-related proteins are color-coded according to phenotype scores indicated in the scale. Autophagy proteins adapted for apicoplast maintenance tend to have negative phenotype scores in tachyzoites, based on a genome-wide CRISPR/Cas9 screen in T. gondii (Sidik et al., 2016), indicating they are essential for parasite survival in the acute stage of infection. Autophagy-related proteins that have a role in canonical autophagy and have not been adapted for apicoplast maintenance tend to have neutral or positive phenotype scores, indicating canonical autophagy is a non-essential cellular pathway in acute stage parasites.
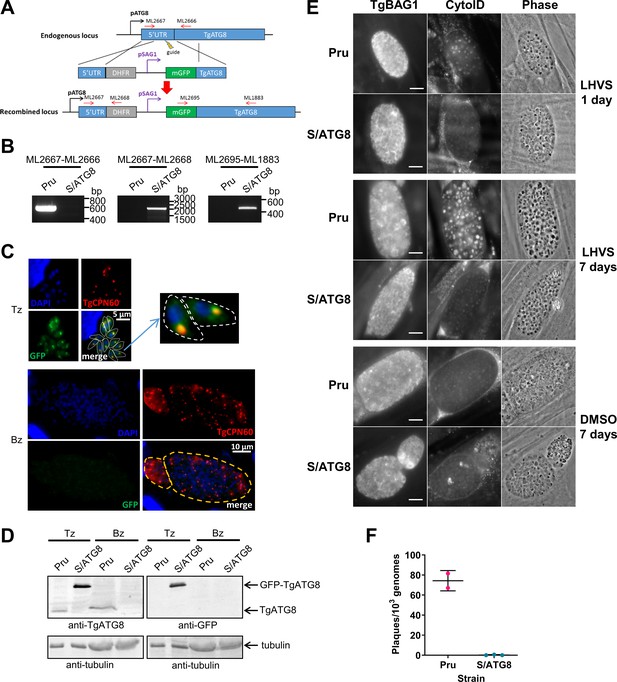
TgATG8 is required for efficient autophagosome production in Toxoplasma gondii bradyzoites.
(A) Stage-specific expression and fluorescent tagging of TgATG8 was achieved by insertion of the tachyzoite-specific TgSAG1 promoter and a GFP-coding sequence immediately upstream of the TgATG8 gene. Site-specific insertion of the DHFR-pSAG1-mGFP repair template was performed by homology-directed repair. (B) Polymerase chain reaction (PCR) analysis confirmed the repair template had been inserted at the correct locus to generate a T. gondii strain in which TgATG8 expression was driven by the TgSAG1 promoter (S/ATG8). Stage-specific expression of GFP-TgATG8 in tachyzoites (Tz) but not bradyzoites (Bz) was confirmed by immunofluorescence microscopy (C) and western blotting (D). GFP-TgATG8 signal overlapped with the apicoplast marker TgCPN60 (a native luminal apicoplast chaperone), indicating correct localization to the apicoplast. Dashed lines outline individual parasites in the tachyzoite images and the limits of cysts on the bradyzoite images. (E) Fluorescent staining of in vitro differentiated cysts with the bradyzoite specific marker TgBAG1 and the autolysosome detection reagent CytoID. Phase contrast images indicate the development of dark puncta in LHVS-treated bradyzoites. Scale bar, 10 µm. (F) Viability of Pru∆hxg and S/ATG8 bradyzoites isolated after 28 days of differentiation, as indicated by their application to fresh monolayers for plaque formation.
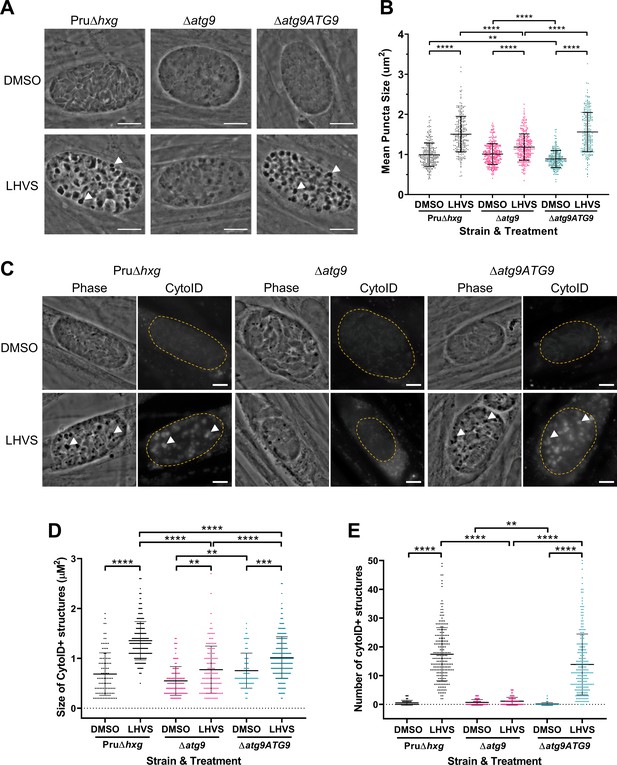
TgATG9 is required for efficient autophagosome production in T. gondii bradyzoites.
(A) Phase contrast images showing that the development of dark puncta in in vitro differentiated bradyzoites following LHVS treatment is dependent on the expression of TgATG9. Arrowheads indicate a subset of dark puncta. Scale bar, 10 µm. (B) Quantification of dark puncta size. Each dot represents the mean size of puncta within one cyst. Data are merged from six biological replicates, with a minimum of 22 cysts analyzed per sample type, per biological replicate, and a minimum total of 242 cysts analyzed per sample type across all biological replicates. (C) CytoID staining of autolysosomes in in vitro differentiated bradyzoite cysts. Arrowheads indicate an arbitrary subset of dark puncta and the corresponding CytoID-positive structures. Scale bar, 5 µm. Quantification of the size (D) and number (E) of CytoID-positive structures in in vitro differentiated bradyzoites. Each dot represents the average puncta measurement within a single cyst. Data are merged from three biological replicates, with a minimum total of 81 cysts analyzed per sample type across three biological replicates. Cysts were identified by Dolichos lectin staining and measurements of dark puncta and CytoID were made exclusively within Dolichos lectin-positive regions. For panels B, D, and E, bars represent mean ± SD. Statistical comparisons were done using a Kruskal-Wallis test with Dunn’s multiple comparisons. Statistical significance is indicated as follows: **p<0.01; ***p<0.001; ****p<0.0001. Non-significant differences are not indicated. Statistical analysis is only shown for comparisons that have one variable, that is, different strain or different treatment.
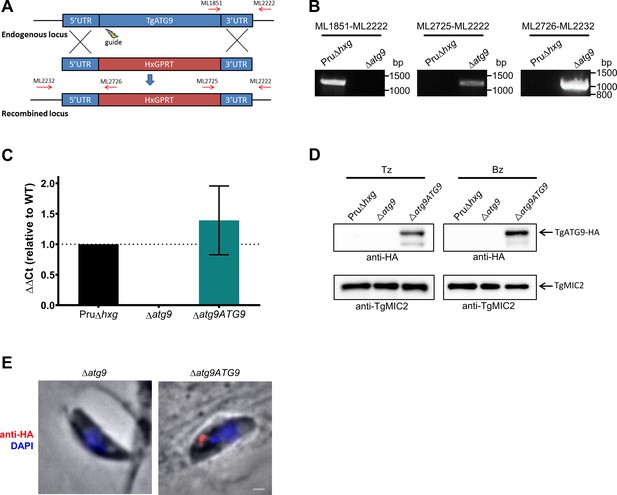
Targeted deletion and genetic complementation of TgATG9.
(A) The TgATG9 gene was replaced with an HXGPRT selectable marker by homology-directed repair facilitated by CRISPR-Cas9 double-strand break directed via a single sgRNA (guide). Primers used to validate correct integration are indicated as red arrows. (B) Polymerase chain reaction (PCR) validation of ∆atg9 parasites using the primers indicated in panel A. (C) Real-time quantitative PCR (qRT-PCR) analysis showing relative transcript levels of TgATG9 in Pru∆hxg, ∆atg9, and ∆atg9ATG9 strains. (D) Western blots of tachyzoites (Tz) and bradyzoites (Bz) showing re-expression of TgATG9-HA in both stages of ∆atg9ATG9 parasites detected with anti-HA antibodies. The same blot was stripped and reprobed with anti-TgMIC2 as a loading control. (E) Immunofluorescence assay (IFA) showing re-expression of TgATG9-HA in a newly invaded tachyzoite. Scale bar, 100 nm.
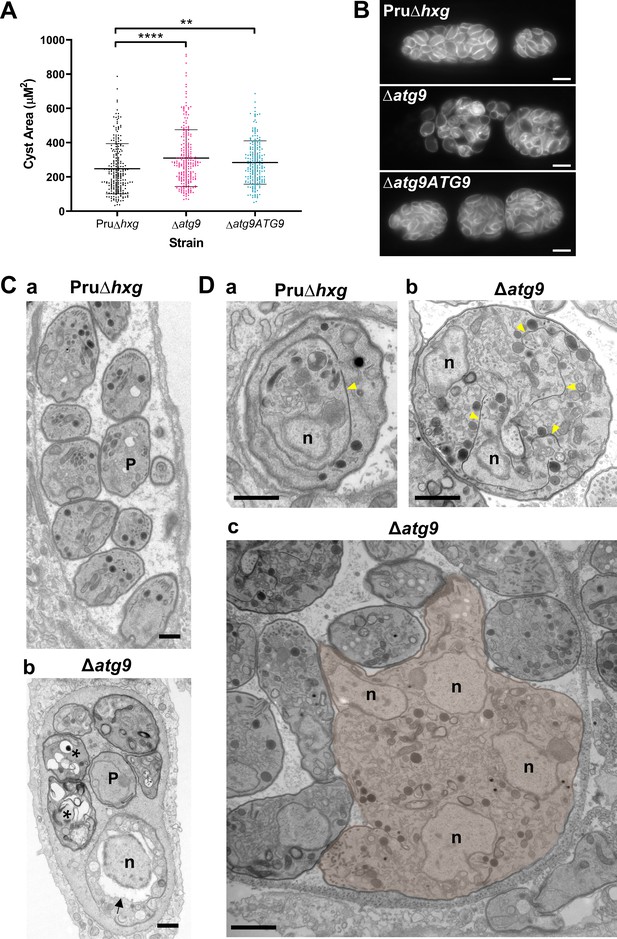
Canonical autophagy is required for normal bradyzoite morphology and cell division.
(A) Quantification of cyst size based on staining for the cyst wall with Dolichos lectin. Each dot represents the size of one cyst. Data are merged from three biological replicates, each with a minimum of 52 cysts analyzed per sample type, per biological replicate, and a minimum total of 199 cysts analyzed per sample type across three biological replicates. Bars indicate mean ± SD. Statistical comparisons were done using a Kruskal-Wallis test with Dunn’s multiple comparisons. Significance is indicated as **p<0.01; ****p<0.0001. Non-significant differences are not indicated. (B) Immunofluorescence imaging of bradyzoites stained with anti-TgIMC1 revealed disorganization of the inner membrane complex in ∆atg9 parasites and a ‘bloating’ phenotype. Scale bar, 10 µm. (C) Transmission electron microscopy (TEM) showing a cyst containing normally developed bradyzoites from Pru∆hxg (a) and aberrant ∆atg9 bradyzoites (b), with highly vacuolized, dying parasites (asterisks) or abnormally enlarged nuclear envelope (arrow). P, parasite. (D) TEM showing normal endodyogeny for Pru∆hxg bradyzoites with a nascent daughter containing well-organized organelles surrounded by the IMC (yellow arrowhead in panel a), and aberrant parasite division with poor packaging of organelles by the IMC (b), resulting in enlarged multinucleated ∆atg9 bradyzoites (pseudocolorized parasite, c). Note that Pru∆hxg and mutant parasites are shown at the same magnification for direct comparison. All scales bars are 1 μm.
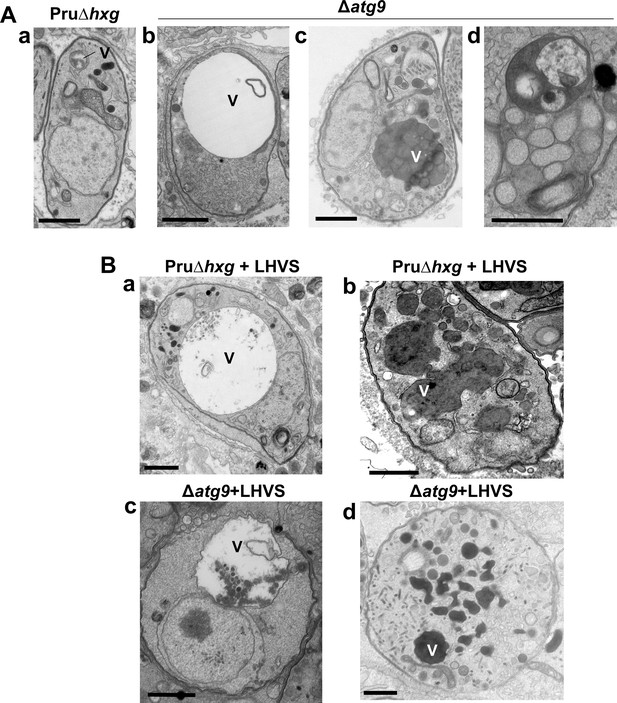
A lack of canonical autophagy leads to ultrastructural abnormalities in the vacuolar compartment (VAC) of bradyzoites.
(A) Transmission electron microscopy (TEM) showing a normal VAC (V) in Pru∆hxg bradyzoites (panel a). Three types of VAC abnormalities were observed in ∆atg9 bradyzoites: a very enlarged electron-lucent (e-lucent) compartment (panel b), small electron-dense vesicles (panel c), or a very enlarged electron-dense compartment (panel d). (B) TEM of VAC in Pru∆hxg and ∆atg9 bradyzoites treated with LHVS showing for both strains very large VAC with e-lucent content (panels a and c) or electron-dense content (panels b and d). All scales bars are 1 μm.
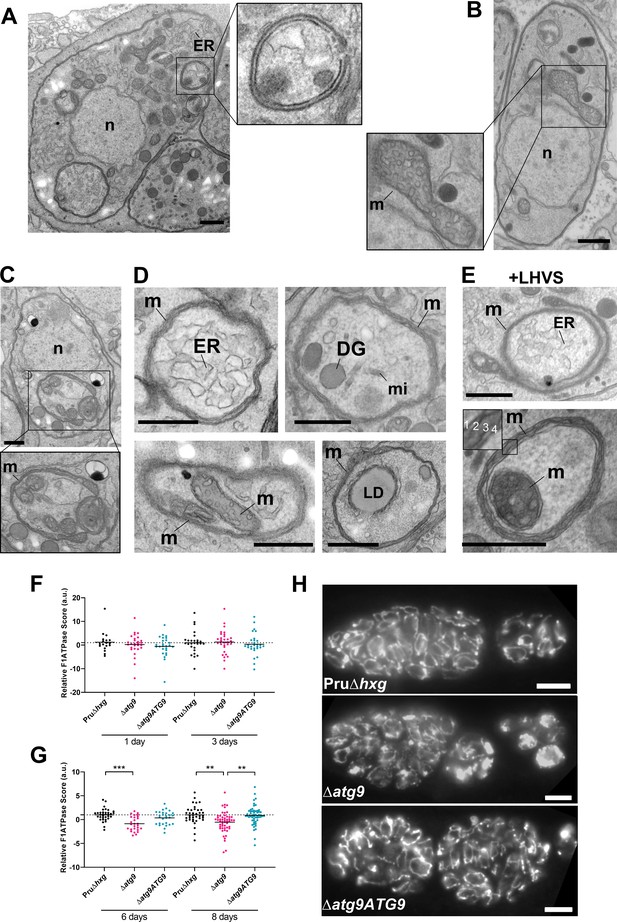
Autophagic structures and abnormal mitochondria in Δatg9 bradyzoites.
(A) Transmission electron microscopy (TEM) of ∆atg9 parasites showing a double-membrane structure containing cytoplasmic components including endoplasmic reticulum (ER). (B) TEM showing a normal mitochondrion in Pru∆hxg bradyzoites. (C) TEM of ∆atg9 bradyzoites presenting thin mitochondria (m) in a horseshoe conformation with bulbous ends, wherein cristae can be seen. Nucleus (n) is denoted. (D) TEM of ∆atg9 parasites revealing mitochondrial profiles (m) enveloping many organelles, including ER, dense granules (DG), micronemes (mi), mitochondria (m), and lipid droplets (LD). (E) Examples of ER and mitochondrial section wrapped by the mitochondrion after bradyzoite treatment with LHVS. Inset of the lower panel illustrates the four membranes observed in such structures. All TEM scale bars are 500 nm. Mitochondria morphology scores (relative F1F0ATPase score) for Pru∆hxg, ∆atg9, and ∆atg9ATG9 parasites 1 and 3 (F) or 6 and 8 (G) days after the addition of alkaline media to stimulate differentiation from the tachyzoite to bradyzoite life stage. Relative F1F0 ATPase Score was normalized to the Pru∆hxg strain within each time point. Each data point represents one cyst. Solid lines represent the mean from three biological replicates. Statistical significance between samples was determined using a Kruskal-Wallis test with Dunn’s multiple comparisons and is indicated with **p<0.01 or ***p<0.001. (H) Immunofluorescence imaging of bradyzoites stained with α-F1F0 ATPase revealed an aberrant mitochondrial network in some ∆atg9 parasites. Scale bar, 10 µm.
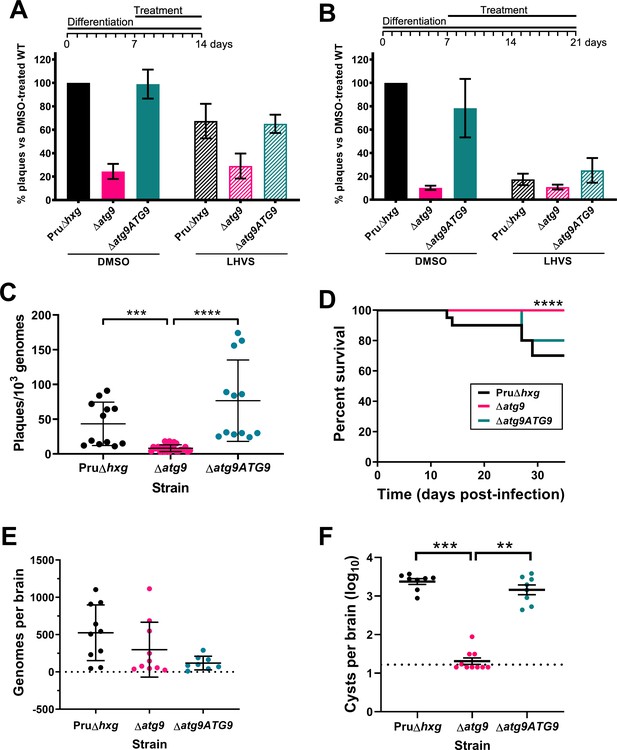
Autophagy is required for bradyzoite viability and the persistence of tissue cysts.
Quantification of bradyzoite viability by quantitative polymerase chain reaction (qPCR)/plaque assay after differentiation media for 1 week followed by treatment with DMSO (vehicle control, blue) or LHVS (red) for a further 1 week (A) or 2 weeks (B). Data are from three biological replicates each with three technical replicates and are normalized to Pru∆hxg set at 100%. Bars represent mean ± SEM. (C) Viability of untreated bradyzoites after 2 weeks of differentiation in vitro. Each dot represents viability from one technical replicate. Data are merged from four biological replicates. Statistical comparisons were done using a Kruskal-Wallis test with Dunn’s multiple comparisons. Significance is indicated as ***p<0.001; ****p<0.0001. Non-significant differences are not indicated. (D) Analysis of virulence based on survival of infected mice. Mice were infected intraperitoneally with 150 tachyzoites of each strain. Infected mice that became moribund before the endpoint at day 35 post-infection were humanly euthanized. Mice infected with ∆atg9 showed significantly better survival based on Mantel-Cox log-rank test (****p<0.0001). (E) Analysis of parasite dissemination to the brain. Brains were harvested from infected mice at 7 days post-infection and the number of genomes present in each brain homogenate was determined by qPCR. No significant differences were seen between strains based on analysis with a Kruskal-Wallis test with Dunn’s multiple comparisons. (F) Analysis of brain cyst burden in infected mice. Brains were harvested from mice 5 weeks post-infection and cysts were counted in blinded samples of brain homogenates by microscopy. Data is plotted as log10 cysts per brain. The dotted line intersecting the y axis represents the limit of detection (<33 cysts/brain). Samples for which no cysts were observed in the 30 µl of homogenate analyzed were given a value that is half the limit of detection. Bars represent mean ± SEM and statistical comparisons were done using a Kruskal-Wallis test with Dunn’s multiple comparisons. Significance indicated as **p<0.01, ***p<0.001. Non-significant differences are not indicated.
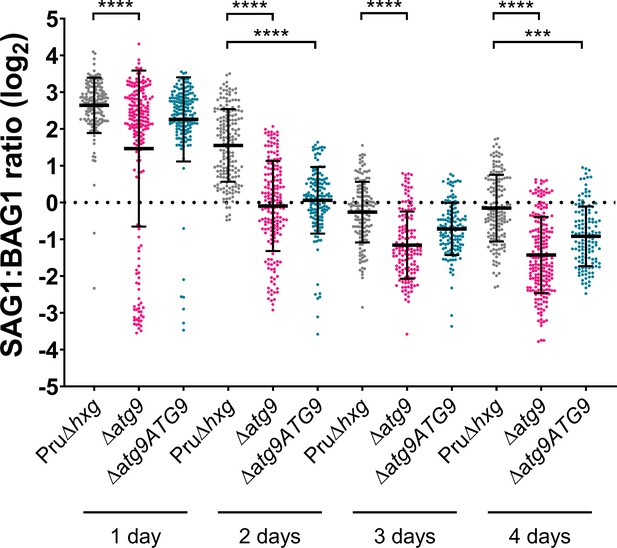
Analysis of differentiation.
In vitro parasitophorous vacuoles (PVs)/cysts were probed for the tachyzoite-specific marker SAG1 and the bradyzoite-specific marker BAG1. The intensity of SAG1 and BAG1 signals in each PV/cyst was determined using a pipeline in the automated image analysis software CellProfiler. The ratio of SAG1:BAG1 was calculated, log2 transformed, and plotted. Each dot represents one technical replicate from three biological replicates. Statistical significance between samples was determined using a Kruskal-Wallis test with Dunn’s multiple comparisons and is indicated with ****p<0.0001 or ***p<0.001. Each biological replicate contained between 38 and 94 technical replicates. Each technical replicate represents an individual PV/cyst. A dotted line dissects the y axis at 0, indicating where SAG1 and BAG1 signal was equal and therefore data points below this line indicate PVs/cysts containing more BAG1 (bradyzoite marker) than SAG1 (tachyzoite marker).
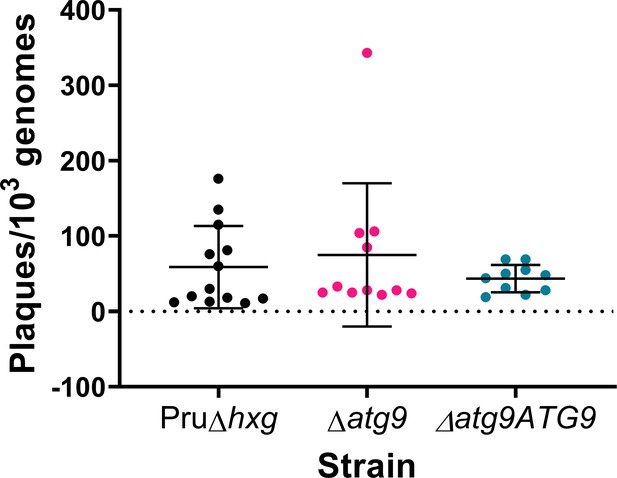
TgATG9 is not required for tachyzoite plaque formation.
Tachyzoites were isolated and applied to fresh cell monolayers for plaque formation over the course of 10 days. Plaques were normalized to 103 genomes of input parasites, as measured by quantitative polymerase chain reaction (qPCR). Each dot represents one technical replicate from three (∆atg9ATG9) or four (Pru∆hxg and ∆atg9) biological replicates. No statistically significant differences were found using a Kruskal-Wallis test with Dunn’s multiple comparisons.
Tables
Phenotypes observed by transmission electron microscopy (TEM).
Phenotype | Pru∆hxg | Δatg9 | ||
---|---|---|---|---|
DMSO | LHVS | DMSO | LHVS | |
Abnormally dividing bradyzoites | <1 (2/144)1 | 8.4 (17/202) | 26.0 (61/235) | 23.2 (44/190) |
Live2 bradyzoites with electron-dense vacuolar compartment (VAC) | 0 (0/144) | 93.1 (188/202) | 12.3 (29/235) | 94.2 (179/190) |
Autophagic structures with two membranes in live bradyzoites | 0 (0/144) | 6.9 (14/202) | 6.0 (14/235) | 10.5 (20/190) |
Abnormally thin mitochondria in live bradyzoites | 0 (0/144) | 11.9 (24/202) | 22.6 (53/235) | 23.7 (45/190) |
Degenerated cysts | 3.0 (1/33) | 11.8 (6/51) | 30.0 (18/60) | 23.4 (11/47) |
-
1Values are percentage (number of bradyzoites or cysts with phenotype/total number of bradyzoites or cysts enumerated).
2Only bradyzoites that appeared to be intact and likely viable were included in the analysis.
Additional files
-
Supplementary file 1
Primers used in this study.
- https://cdn.elifesciences.org/articles/59384/elife-59384-supp1-v2.docx
-
Supplementary file 2
CellProfiler parameters for determining mitochondria morphology.
- https://cdn.elifesciences.org/articles/59384/elife-59384-supp2-v2.docx
-
Transparent reporting form
- https://cdn.elifesciences.org/articles/59384/elife-59384-transrepform-v2.pdf