Excitatory and inhibitory receptors utilize distinct post- and trans-synaptic mechanisms in vivo
Figures
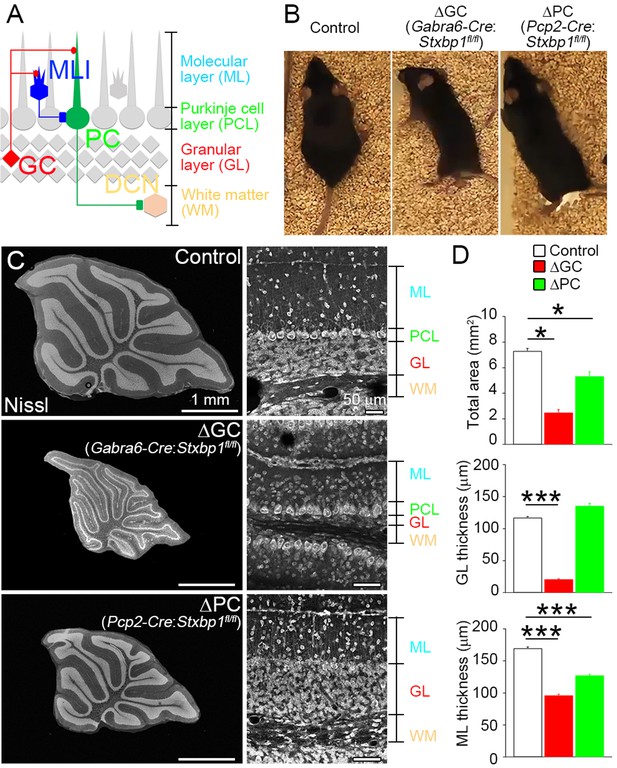
Adult cerebellar organization after cell-autonomous elimination of neurons.
(A) Synaptic circuit organization in the adult cerebellum. Granule cells (GCs) send excitatory inputs to Purkinje cells (PCs) and molecular layer interneurons (MLIs). MLIs form inhibitory synapses on to PCs that send inhibitory inputs to the deep cerebellar nucleus (DCN). (B) Cerebellar GC- and PC-specific conditional Stxbp1 homozygous mice (ΔGC mice and ΔPC mice, respectively) manifest ataxia at 5–6 months of age (see Extended Data Videos 1 and 2). (C) Cerebellar histology of control (top), ΔGC (middle), and ΔPC (bottom) mice at P30. (D) Significant decrease in the total cerebellar area and the thicknesses of granular layer (GL) and molecular layer (ML) (n = 45–67 regions from three mice each). Note that gross histoarchitecture of cerebellum is maintained in ΔGC and ΔPC mice. Data are means ± SEMs; Kruskal–Wallis test with Steel post-test; *p < 0.05, ***p < 0.001. The numerical values are summarized in source data.
-
Figure 1—source data 1
Adult cerebellar organization after cell-autonomous elimination of neurons.
- https://cdn.elifesciences.org/articles/59613/elife-59613-fig1-data1-v2.xlsx
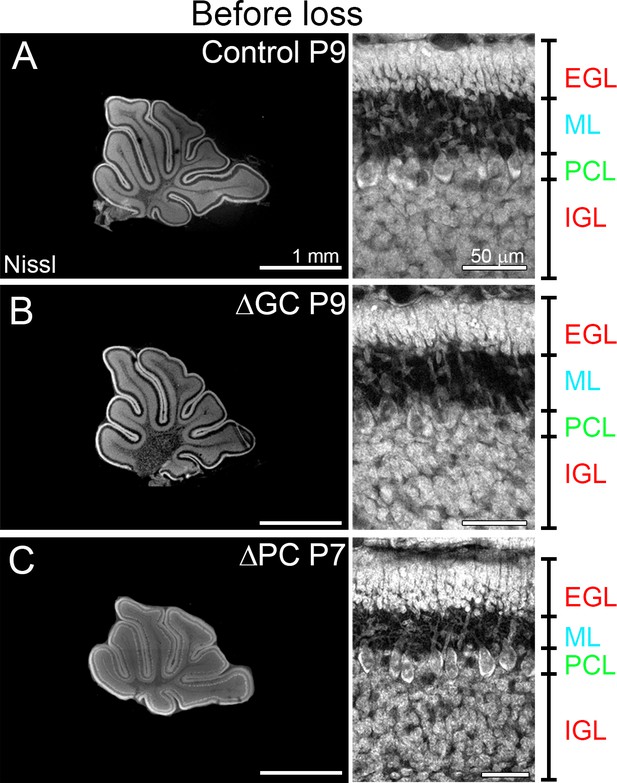
Normal cerebellar histology of mouse brains at P7−9.
Cerebellar granule cell (GC)-specific and Purkinje cell (PC)-specific conditional Stxbp1 homozygous mice (ΔGC mice and ΔPC mice, respectively) are generated, and cerebellar structures are examined by fluorescent Nissl staining at the late stage of cerebellar development, around P7−9. The four layers, namely, the external granular layer (EGL), molecular layer (ML), Purkinje cell layer (PCL), and internal granular layer (IGL) formed similarly in control (A), ΔGC mice (B), and ΔPC mice (C). This suggests normal cerebellar formation at early postnatal stage of cerebellar development.
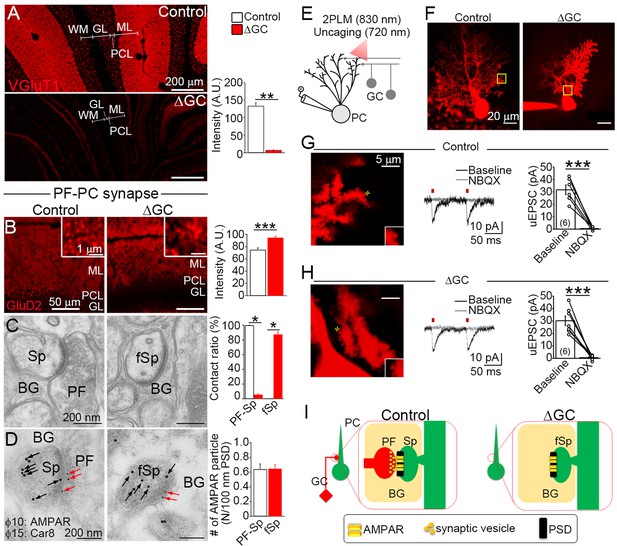
Excitatory AMPARs remain at spiny synapses without presynaptic terminals.
(A) Vesicular glutamate transporter (VGluT1) signal from GC axons, that is, parallel fibers (PFs), is significantly decreased in the molecular layer (ML) of the ΔGC mice (n = 6 images from three mice each). A.U.: arbitrary units. (B–D) Analysis of parallel fiber (PF)–Purkinje cell (PC) spiny synapses. (B) GluD2 show punctate localization in the ML and increase signal intensity in the ΔGC mice (n = 20 images from three mice each). A.U.: arbitrary units. (C) Electron micrographs showing a spine (Sp) making synaptic contact with a PF in a control mouse and a free spine (fSp) lacking synaptic contact in a ΔGC mouse (n = 6 regions from three mice each). BG: Bergmann glia. (D) Postembedding immunogold for panAMPAR (10 nm, red arrows) on Car8 (15 nm, black arrows)-labeled PC spines (Sp) or a free spine (fSp) in control and ΔGC mice, respectively (n = 114–130 from three mice each). BG: Bergmann glia. (E) Schematic diagram illustrating the experimental design of combined electrophysiology, imaging and uncaging in acute cerebellar slices from ΔGC and control littermates. Excitation wavelengths employed were 830 nm (for imaging) and 720 nm (for glutamate uncaging). (F) 2 P reconstruction of a Purkinje cells loaded with Alexa 594 in ΔGC and control mice. Left, example of the position of the uncaging laser beam (yellow cross) in control (G) and ΔGC (H) littermates. Middle, representative uncaging glutamate-evoked excitatory postsynaptic currents (uEPSCs). NBQX (10 µM) was bath applied to block AMPARs. Right, summary plot demonstrating that NBQX (10 µM) inhibits uEPSC evoked by glutamate uncaging (control, 6 cells from 3 mice; ΔGC, 7 cells from 4 mice). Data are presented as mean ± SEM. ***p < 0.001, paired t-test. (I) After ablation of PFs AMPAR clustering and postsynaptic density (PSD)-like specialization are maintained on free PC spines in ΔGC mice. BG: Bergmann glia. Data are means ± SEMs, Mann–Whitney U-test (A–D), *p < 0.05, **p < 0.01, ***p < 0.001. The numerical values are summarized in source data.
-
Figure 2—source data 1
Excitatory AMPARs remain at spiny synapses without presynaptic terminals.
- https://cdn.elifesciences.org/articles/59613/elife-59613-fig2-data1-v2.xlsx
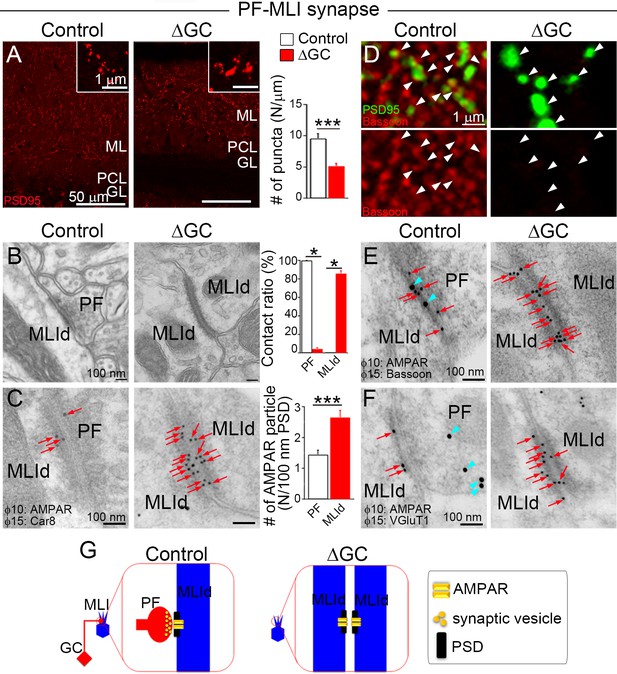
Excitatory AMPARs remain at shaft synapses without presynaptic terminals.
(A) The number of postsynaptic density (PSD)-95 puncta is reduced (n = 6 images from three mice each) and individual puncta is enlarged in molecular layer (ML) of the ΔGC mice (n = 1421 puncta in control and 686 in ΔGC from three mice each). (B) Electron micrographs showing a typical parallel fiber (PF)–molecular layer interneuron (MLI) synapse on MLI dendritic shaft (MLId) shaft synapse in a control mouse and an atypical dendrodendritic contact between MLIs in a ΔGC mouse (n = 6 images from three mice each). (C) Postembedding immunogold for panAMPAR (10 nm, red arrows) and Car8 (15 nm) (n = 25–42 from three mice each). Dendrites of MLI are identified by negative labeling for Car8 and the lack of dendritic spines. A bar graph shows the contact ratio of MLId with PF terminals or other MLId. (D) Double immunofluorescence for bassoon (red) and PSD-95 (green). Arrowheads indicate PSD-positive puncta. Note that PSD-95 signals are facing to bassoon signals in control. (E, F) Double-labeling postembedding immunogold for panAMPAR (10 nm, red arrows) (n = 25–42 from three mice each) and active zone protein, bassoon (15 nm, blue arrowheads in E) or synaptic vesicle-associated protein vesicular glutamate transporter (VGluT1) (15 nm, blue arrowheads in F). Presynaptic proteins bassoon and VGluT1 are not detected at and around dendrodendritic contact sites. (G) After ablation of PFs, AMPAR clustering and PSD-like specialization are maintained in dendrodendritic contact sites between MLIs in ΔGC mice. Data are means ± SEMs, Mann–Whitney U-test (A–C), *p < 0.05, ***p < 0.001. The numerical values are summarized in source data.
-
Figure 3—source data 1
Excitatory AMPARs remain at shaft synapses without presynaptic terminals.
- https://cdn.elifesciences.org/articles/59613/elife-59613-fig3-data1-v2.xlsx
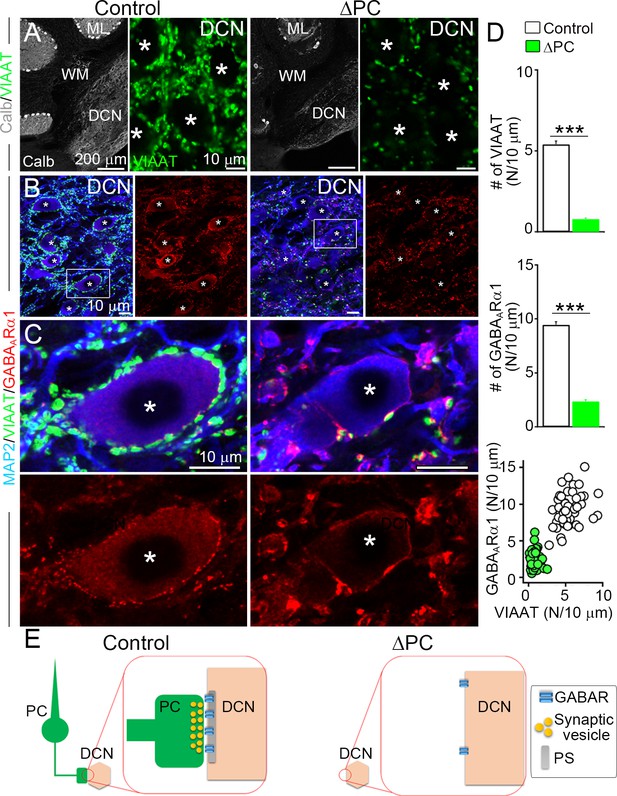
Inhibitory GABAARs require presynaptic terminals in the deep cerebellar nuclei (DCN).
(A) Immunofluorescence showing marked reductions of calbindin (Calb)-labeled Purkinje cells (PCs) in the cerebellar cortex and of vesicular inhibitory amino acid transporter (VIAAT)-labeled inhibitory terminals in the DCN of the ΔPC mice. (B, C) Triple immunofluorescence for MAP2(microtubule associated protein 2) (blue), VIAAT (green), and GABAARα1 (red) in DCN boxed regions in B are enlarged in C. Asterisks indicate cell bodies of DCN neurons, which are fringed by numerous bright clusters of GABAARα1 in control mice but are greatly reduced in ΔPC mice. (D) Bar graphs showing reduced densities of VIAAT (top)- and GABAARα1 (middle)-positive puncta on the surface of DCN neurons in ΔPC mice (number per 10 μm, n = 49–60 neurons from three mice each). Kruskal–Wallis test followed by Steel–Dwass post-test for the comparison of scatter plot (bottom) indicates significant reduction (p < 0.001). (E) After ablation of PC terminals, postsynaptic GABAARα1 is declustered from somatic membrane of DCN neuron. Data are shown as means ± SEMs, ***p < 0.001. The numerical values are summarized in source data.
-
Figure 4—source data 1
Inhibitory GABAARs require presynaptic terminals in the deep cerebellar nuclei (DCN).
- https://cdn.elifesciences.org/articles/59613/elife-59613-fig4-data1-v2.xlsx
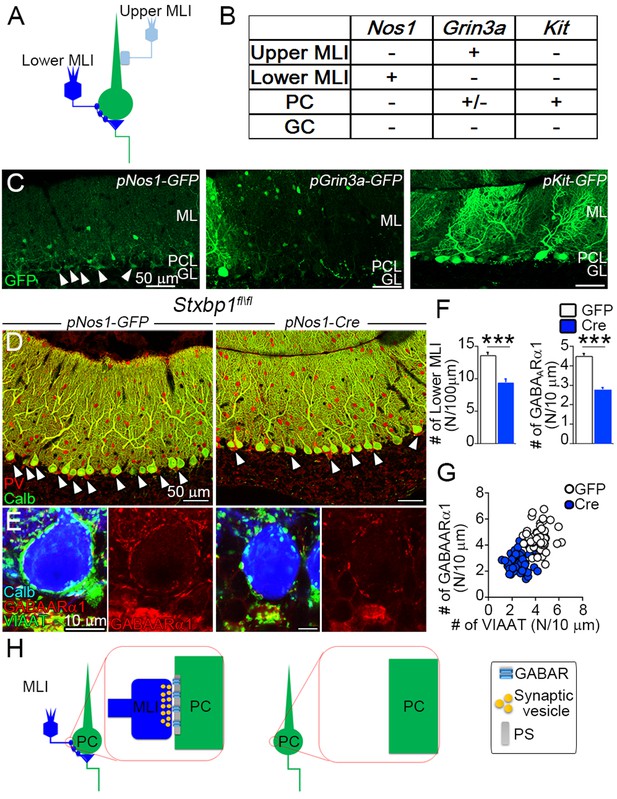
Inhibitory postsynaptic sites at Purkinje cell (PC) somas require presynaptic terminals.
(A) Wiring diagram between molecular layer interneurons (MLIs) and PCs. Lower MLIs, also known as basket cells, innervate PC somata and surround the axon initial segment of PCs with the pinceau formation. Upper MLIs corresponding to stellate cells innervate dendritic shafts of PCs. (B, C) Adenoassociated viruses (AAVs) under different promoters targeting to MLIs. Summary of GFP expression is shown in a table (B) and representative images (C). GFP expression is found preferentially in lower MLIs and pinceau formation in AAV pNos1-GFP (arrowheads in C). (D, E) Cerebella of Stxbp1fl/fl mice-injected AAV pNos1-GFP (right) and -Cre (left). Multiple labeling for calbindin (green) and parvalbumin (red) in D and for GABAARα1 (red), vesicular inhibitory amino acid transporter (VIAAT) (green), and calbindin (blue) in E. (F) The densities of lower MLIs (left, N/100 μm of PC layer, n = 12–15 images from three mice each, Mann–Whitney U-test) and GABAARα1-positive puncta on PC somata (right, N/10 μm of membrane, n = 55 neurons from three mice each, Student’s t-test). (G) Scatter plot of the number of GABAARα1- (vertical axis) against VIAAT-positive puncta (horizontal axis) on the surface of each PC soma (N/10 μm, right, n = 55 neuron from 3 mice each, one-way analysis of variance) (ANOVA) (F(3,216) = 94.8, with Bonferroni post hoc test, p < 0.001). (H) Postsynaptic GABAARα1 requires inhibitory presynaptic terminals at MLI–PC synapse. After ablation of MLI terminals, postsynaptic GABAARα1 is declustered from somatic membrane of PC. Data are shown as means ± SEMs; ***p < 0.001. The numerical values are summarized in source data.
-
Figure 5—source data 1
Inhibitory postsynaptic sites at Purkinje cell (PC) somas require presynaptic terminals.
- https://cdn.elifesciences.org/articles/59613/elife-59613-fig5-data1-v2.xlsx
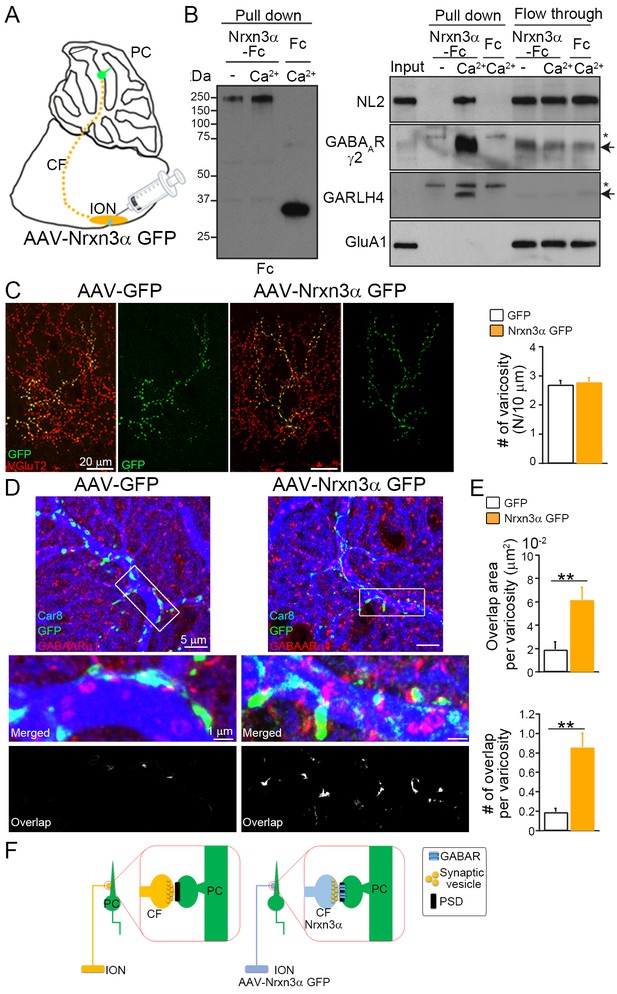
Ectopic Nrxn3 expression in excitatory climbing fibers (CFs) recruits inhibitory GABARs.
(A) Adenoassociated viruses (AAVs) carrying GFP or Nrxn3α fused with GFP (Nrxn3α GFP) are injected into the inferior olivary nucleus (IONs) that project excitatory CFs to Purkinje cells (PCs). (B) The extracellular domain of neurexin-3 alpha (Nrxn3α) fused with Fc and secreted Fc are pulled down with protein A and used for pull down with adult mouse cerebellar lysate with and without calcium (Ca2+). Nrxn3α-Fc pulls down neuroligin 2 (NL2), GABAARγ2, and GARLH4, but not GluA1, in a calcium-dependent manner. The unbound proteins are detected in both conditions with and without calcium, suggesting no obvious protein degradation. Arrows and asterisks indicate specific and nonspecific bands, respectively. The raw images are provided in source data. (C) Both GFP and Nrxn3α-GFP (green) are colocalized with presynaptic VGluT2 (red) along CF arbors. No significant difference is found between AAV-GFP and AAV-Nrxn3α GFP in the number of GFP-positive varicosities per 10 μm axon of labeled CFs (N/10 μm) (n = 34–50 images from three GFP- and Nrxn3α GFP-injected mice). (D, E) GABAAR clustering at CF–PC synapses is frequently detected after transfection of AAV-Nrxn3α GFP, as shown by triple immunofluorescence for Car8 (blue), GFP (green), and GABAARα1 (red). (E) The area and number of overlapped regions between GFP and GABAARα1 per single CF varicosity (n = 7–18 images from three AAV-injected mice). (F) Nrxn3α expression in excitatory CF induces clustering of inhibitory GABAARα1 at the CF–PC postsynapse. Data are shown as means ± SEMs, Mann–Whitney U-test (D, E); **p < 0.01. The numerical values are summarized in source data.
-
Figure 6—source data 1
Ectopic Nrxn3 expression in excitatory climbing fibers (CFs) recruits inhibitory GABARs.
- https://cdn.elifesciences.org/articles/59613/elife-59613-fig6-data1-v2.xlsx

Elimination of presynaptic neurons reduces synapse number without producing free spines in hippocampus.
(A–C) Electron micrographs showing CA1 region of wild-type (A) and Stxbp1fl/fl mice injected with AAV-synapsin promoter-driven (pSynapsin) Cre (B) and wild-type injected with AAV-pSynapsin Cre and -pSynapsin flex diphteria toxin A (DTA). AAVs were injected at both sides of CA3 region at P30–40. In CA3 elimination groups (B, C), spines contacting to terminals with forming asymmetry synapse (asterisks) was significantly decreased. (D, E) Graphs showing the number of synapses at CA1 regions (D) (N/100 µm2, n = 10–30 images from three mice) and the ratio of free spine relative to total spines (E) (n = 10–30 images from three mice). Note free spines were rarely produced in elimination of CA3 neuron. Data are shown as means ± SEMs, Kruskal–Wallis test followed by Steel–Dwass post-test; ***p < 0.001.
Videos
Sample video of two mice eliminating cerebellar granule cells (ΔGC) and two control mice.
Sample video of two mice eliminating cerebellar Purkinje cells (ΔPC) and two control mice.
Tables
Reagent type (species) or resource | Designation | Source or reference | Identifiers | Additional information |
---|---|---|---|---|
Strain, strain background (Escherichia coli) | Putative Nos1 promoter | BACPAC Resources Center (BPRC) | BAC: RP24-84E3 | |
Strain, strain background (Escherichia coli) | Putative Grin3a promoter | BACPAC Resources Center (BPRC) | BAC: RP23-104D24 | |
Strain, strain background (Escherichia coli) | Putative Kit promoter | BACPAC Resources Center (BPRC) | BAC: RP23-232H18 | |
Strain, strain background (Mus musculus) | Wild-type (C57BL/6 J) | The Jackson Laboratory | Stock# 00064 | |
Strain, strain background (Mus musculus) | Mouse: Stxbp1lox/lox | Verhage et al., 2000 | N/A | |
Strain, strain background (Mus musculus) | Mouse: Tg(Gabra6-Cre) | MMRRC | ID# 015966-UCD | |
Strain, strain background (Mus musculus) | Mouse:Tg(Pcp2-Cre) | The Jackson Laboratory | Stock# 004146 | |
Cell line (Homo sapiens) | 293AAV | Cell Biolab | Cat#: AAV-100 | |
Antibody | anti- GABAAR α1(Rabbit polyclonal) | Frontier Inst. | Cat# GABAARa1-Rb-Af660 RRID:AB_2571571 | IHC(1 μg/ml) |
Antibody | anti-GluD2(Rabbit polyclonal) | Frontier Inst. | Cat# GluD2C-Rb-Af1200 RRID:AB_2571601 | IHC (1 μg/ml) |
Antibody | anti-panAMPAR(Guinea pig polyclonal) | Frontier Inst. | Cat# panAMPAR-GP-Af580, RRID:AB_2571610 | IHC (1 μg/ml)IEM (5 μg/ml) |
Antibody | anti-VGluT1(Guinea pig polyclonal) | Frontier Inst. | Cat# VGluT1-GP-Af570, RRID:AB_2571534 | IHC (1 μg/ml)IEM (10 μg/ml) |
Antibody | anti-VGluT2(Guinea pig polyclonal) | Frontier Inst. | Cat# VGluT2-GP-Af810, RRID:AB_2341096 | IHC (1 μg/ml) |
Antibody | anti-VIAAT(Guinea pig polyclonal) | Frontier Inst. | Cat# VGAT-GP-Af1000, RRID:AB_2571624 | IHC (1 μg/ml) |
Antibody | anti-PSD95(Guinea pig polyclonal) | Frontier Inst. | Cat# PSD95-GP-Af660, RRID:AB_2571539 | IHC (1 μg/ml) |
Antibody | anti-GFP(Goat polyclonal) | Frontier Inst. | Cat# GFP-Go-Af1480, RRID:AB_2571574 | IHC (1 μg/ml) |
Antibody | anti-calbindin(Goat polyclonal) | Frontier Inst. | Cat# Calbindin-Go-Af1040, RRID:AB_2532104 | IHC (1 μg/ml) |
Antibody | anti-MAP2(Goat polyclonal) | Frontier Inst. | Cat# MAP2-Go-Af860, RRID:AB_2571557 | IHC (1 μg/ml) |
Antibody | anti-Car8(Goat/Rabbit polyclonal) | Frontier Inst. | Cat# Car8-Go-Af780, RRID:AB_2571668Cat# Car8-Rb-Af330, RRID:AB_2571667 | IHC (1 μg/ml)IEM (10 μg/ml) |
Antibody | anti-Bassoon(Mouse monoclonal) | Enzo | Cat# SAP7F407, RRID:AB_2313990 | IHC (1 μg/ml)IEM (20 μg/ml) |
Antibody | anti-GABAAR γ2(Rabbit polyclonal) | Millipore | Cat#: AB5559, RRID: AB_11211236 | WB (1:2000) |
Antibody | anti-GARLH4(Rabbit polyclonal) | Yamasaki et al., 2017 | N/A | WB (0.1 μg/ml) |
Antibody | anti-GluA1(Rabbit polyclonal) | Millipore | Cat#: AB1504, RRID: AB_2113602 | WB (1:2000) |
Recombinant DNA reagent | pAAV-DJ(plasmid) | Cell Biolabs | VPK-410-DJ | |
Recombinant DNA reagent | pHelper(plasmid) | Cell Biolabs | VPK-410-DJ | |
Recombinant DNA reagent | pAAV-MCS(plasmid) | Cell Biolabs | VPK-410 | |
Recombinant DNA reagent | pAAV-Promoter less(plasmid) | This paper | N/A | pAAV-MCS (Cell Biolabs) |
Recombinant DNA reagent | Nrxn3alpha pXY-Asc(plasmid) | Horizon | Cat# MMM1013-202798372 | |
Sequence-based reagent | B1.Nrxn3a.For | This paper | PCR primers | GGGGACAAG TTTGTACAAAA AAGCAGGCTC CACCATGAG CTTTACCCTCCACTCAG TTTTCTTC |
Sequence-based reagent | Nrxn3a(-).B5.Rev | This paper | PCR primers | GGGGACAACT TTTGTATACAA AGTTGTCACAT AATACTCCTTG TCCTTGTTTTT CTGTTTC |
Sequence-based reagent | B5.(-M)AcGFP.Fo | This paper | PCR primers | GGGGACAACTTTGTATACAAAAGTTGTGAGCAAGGGCGCC GAGCTGTTC |
Sequence-based reagent | AcGFP*.B2.Rev | This paper | PCR primers | GGGGACCAC TTTGTACAAGA AAGCTGGGT TCACTTGTAC AGCTCATCCATGCC |
Sequence-based reagent | AsCI.DEST.for | This paper | PCR primers | TACATGGCGC GCCACAAGTT TGTACAAAAAAGC |
Sequence-based reagent | DEST.BsiWI.rev | This paper | PCR primers | ATGTACGTAC GACCACTTTG TACAAGAAAGC |
Sequence-based reagent | B5.cre.For | This paper | PCR primers | GGGGACAAC TTTGTATACAA AAGTTGCCACC ATGTCCAATTT ACTGACCG TACACC |
Sequence-based reagent | cre*.B2.Rev | This paper | PCR primers | GGGGACCACT TTGTACAAGAA AGCTGGGTTCA ATCGCCATCTT CCAGCAGGCG |
Sequence-based reagent | B1.pNOS.For | This paper | PCR primers | GGGGACAAGT TTGTACAAAAA AGCAGGCTC CCCTCACCCAT CCCCACCCAC CTCCATCCATAC |
Sequence-based reagent | pNOS.B5.Rev | This paper | PCR primers | GGGGACAACTTTTGTATACAAAGTTGTT GCCGTTCGGC CTTGGGTGG CATGATTTC |
Sequence-based reagent | B1.pGRIN3A.For | This paper | PCR primers | GGGGACAAGTTTGTACA AAAAAGCAG GCTCCCTGC CGTGCAAGGA CCACACATT CTACACTATAC |
Sequence-based reagent | pGRIN3A.B5.Rev | This paper | PCR primers | GGGGACAACTT TTGTATACAAA GTTGTCGGCCA CCTTACCGCGG GCTCCCCCA GCGCCTGG |
Sequence-based reagent | B1.pC-KIT.For | This paper | PCR primers | GGGGACAAGTTTGTACAAA AAAGCAGGCTG TCCACCCCCG GATAGCCACAG TGACTGTGAAATG |
Sequence-based reagent | pC-KIT.B5.Rev | This paper | PCR primers | GGGGACAACTTTTGTATACAAAGTTGT GTGCACCGAG CGCGGCAAA GCCGAGC |
Commercial assay or kit | Endofree QIAGEN Maxi kit | QIAGEN | QIAGEN Cat#12,362 | |
Chemical compound, drug | L-Glutamine | GIBCO | 25030–081 | |
Chemical compound, drug | Penicillin– streptomycin | GIBCO | 15140–122 | |
Chemical compound, drug | DMEM media | SIGMA | 11965092 | |
Chemical compound, drug | Iscove’s modified DM (IMDM) | Thermo | 12440053 | |
Chemical compound, drug | Benzonase nuclease | Sigma | E1014-25kU | |
Chemical compound, drug | Pfu Turbo DNA polymerase | Agilent | 600,250 | |
Software, algorithm | MetaMorph | Molecular Devices | RRID:SCR_002368 |