Processing of motion boundary orientation in macaque V2
Figures
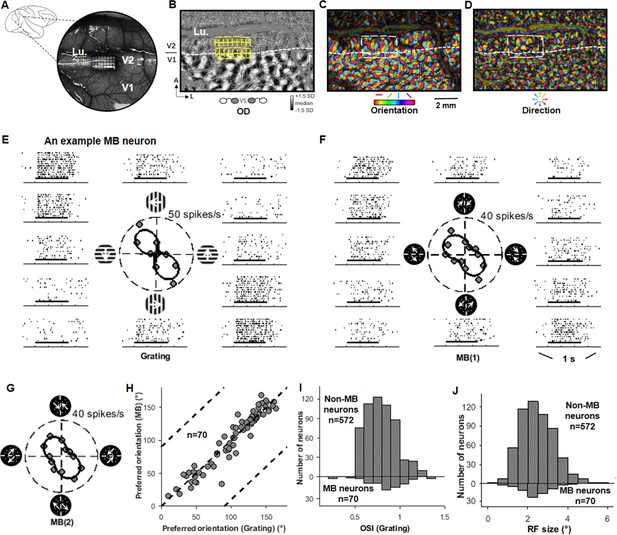
Map-guided array recording and V2 motion boundary (MB) neurons.
(A) Illustration of an imaging chamber location and a 32-channel (4 × 8) array in an example case. Lu: lunate sulcus. (B) An ocular dominance map showing patterns in V1. The location of a 32-channel array is illustrated. (C) An orientation map for the same cortex region shown in (B). (D) A motion-direction map for the same cortex region shown in (B). (E) The responses of an example V2 neuron to sine-wave gratings presented in 12 drifting directions in 30° steps (six orientations). Short horizontal bars indicate stimulus presentation time (500 ms). (F) The responses of the same neuron to 12 MB1 stimuli (six orientations). In each MB1 stimulus, the moving axis of the random dots (RDs) was 45° clockwise from the midline. Each orientation was presented two times in which the RD-moving directions in the two sides were switched, plotted on opposite sides of the polar plot (also see Figure 1—figure supplement 1E). This neuron exhibited a strong MB orientation tuning, which was similar to its tuning to gratings (E). (G) Similar to (F), the responses of the same neuron to another set of MB stimuli (MB2), in which motion axis of the RDs had a different angle with the MB (135° clockwise from the midline). Also see Figure 1—figure supplement 1E. (H) For the 70 MB neurons, their preferred MB orientations were similar to their preferred grating orientations. (I) The distributions of the orientation selectivity index, measured with sine-wave gratings, for MB and non-MB neurons in V2. The MB neurons showed stronger orientation selectivity than the non-MB neurons (Wilcoxon test, p<0.01). (J) The distributions of the receptive field sizes for the MB neurons and the non-MB neurons in V2 were similar.
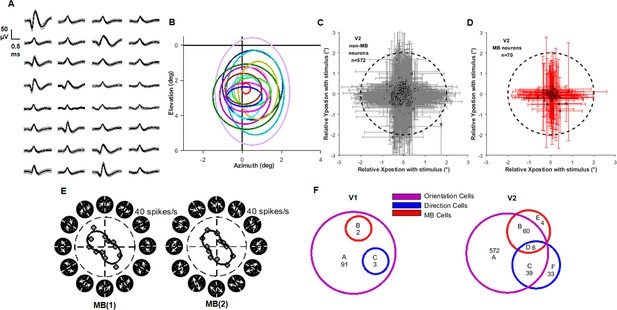
Additional information on array recordings.
(A) An example of waveforms of isolated units in a 32-channel array recorded in 1 day. (B) Receptive fields of 13 neurons recorded in 1 day’s recording shown in (A). (C) Receptive field (RF) positions and sizes of all non-motion boundary (MB) neurons (gray crosses) in relative to the MB stimulus (dotted circle). (D) Similar to (C), but for all MB neurons. (E) The orientation tuning plots of the example neuron shown in Figure 1F, G with all the MB stimuli were plotted. (F) The censuses of recordings from V1 and V2. Numbers in each circle represent neurons that passed that test. The following three types of neurons were described in the results: ‘MB neurons’ are those in red circles (B + D + E). ‘Non-MB neurons’ are those labeled ‘A’. Note that we excluded DS neurons (C + F) from the ‘non-MB’ group since they respond strongly to the random dots in the MB stimuli. ‘DS neurons’ are those in blue circle ‘C + D + F’. Note that the four neurons in V2 group E were not orientation neurons (did not reach the criteria for orientation selectivity to grating stimuli). However, they exhibited MB orientation selectivity. Thus, these four neurons were considered MB neurons and included in the MB results.
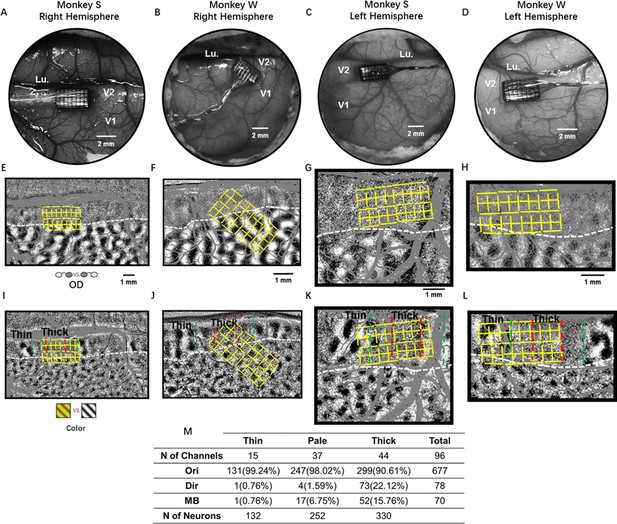
Array locations in relative to the V2 compartments.
(A–D) Images of all four array implants in four hemispheres. (E–H) Corresponding to (A–D), ocular dominance maps imaged before the array implants. The white dashed lines represent V1/V2 borders according to the V1 ocular dominance patterns. (I–L) Corresponding to (E–H), color maps imaged before the array implants. The white dotted lines represent V1/V2 borders. Thin stripes were identified based on the color/luminance patches in V2 (black and white patched in V2). Thick (between two red dashed lines) and pale (between green and red dashed lines) stripes were identified between the thin stripes and according to their width ratio (thick:pale = 2:1.5, Shipp and Zeki, 1989). (M) Numbers of different types of neurons recorded from each stripe type and percentages of the neurons in each stripe. Note that the tuning types were not mutually exclusive (e.g., a motion boundary neuron could also be an orientation neuron).
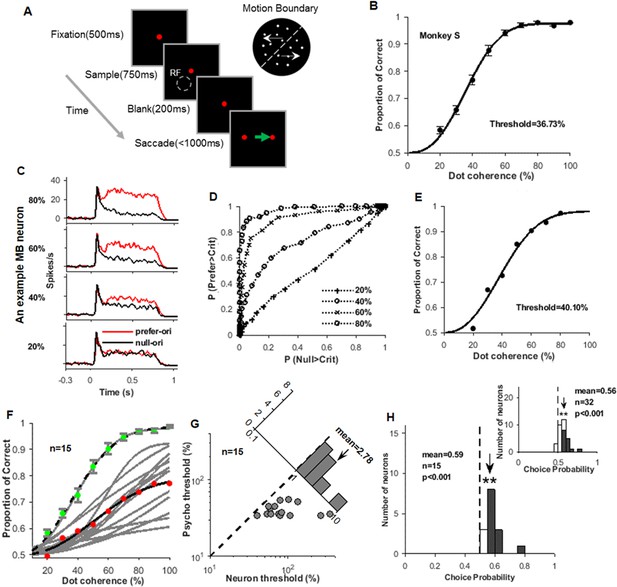
Animal performance and neural responses in the orientation-discrimination task.
(A) An illustration of the motion boundary (MB)-orientation-discrimination task. A fixation point appeared for 500 ms, then a MB stimulus appeared for 750 ms. The fixation point maintained for additional 200 ms before it was replaced by two saccade targets. During this period, the monkey was required to maintain fixation at the fixation point. The monkey needed to make an eye saccade choice within 1000 ms, according to the tilt of the MB line. For example, for MB stimulus tilted to the right of vertical (as shown in the top right), the monkey should saccade to the right target. (B) The psychometric function of monkey S in the MB-orientation-discrimination task. (C) The response peri-stimulus time histograms (PSTHs) of an example MB neuron to MB stimuli presented different levels of motion coherence (four out of seven were shown), and the red and black curves are for the preferred and null MB orientations, respectively. (D) The receiver operating characteristic (ROC) curves obtained from the response PSTHs in (C), and horizontal and vertical coordinates are false alarm rate and hit rates, respectively. (E) The neurometric function obtained from the ROC curves in (D). (F) The neurometric functions (gray) of the 15 MB neurons recorded with the coherence stimuli. The red dots represent the average of the 15 neurometric functions, and the black line is its fitted curve. The green dots represent the average psychometric function measured during the recordings of the 15 neurons, and the black dashed line is its fitted curve. (G) Comparison of the neuronal thresholds and behavioral thresholds. The inset plot shows the distribution of ratios of the neural and behavioral thresholds. (H) The distribution of the choice probability (CP) values for the 15 MB neurons tested with the coherence stimuli, in which 12 neurons had CPs significantly larger than 0.5 (filled bars). The mean CP value (0.59) is also larger than 0.5 (t-test, p<0.001). The inset plot shows the distribution of CPs for all of the 32 neurons, including 15 tested with coherence stimuli (shown in the main panel) and 17 tested with brightness stimuli (also see Figure 2—figure supplement 1B–E).
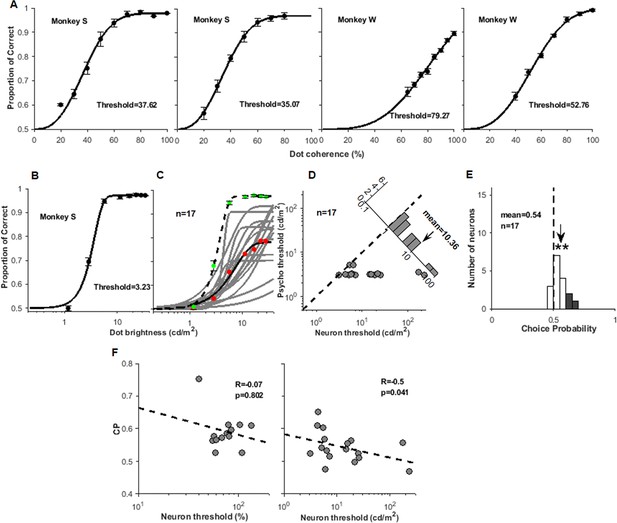
Additional information for behavioral tests.
(A) Four psychometric functions measured during four array recordings using coherent stimuli. (B) The psychometric function measured in monkey S using brightness stimuli. (C) The 17 neurometric functions (gray) measured with brightness stimuli in monkey S. The red dots represent the average of the 17 neurometric functions, and the black line is its fitted curve. The green dots represent the average psychometric function measured during the recordings of the 17 neurons, and the black dashed line is its fitted curve. (D) Comparison of behavioral and neuronal thresholds for the 17 neurons recorded using brightness stimuli. The inset shows the ratio of neural-behavioral thresholds. (E) The distribution of choice probabilities (CPs) measured with brightness stimuli, three of which were significantly larger than 0.5 (bootstrap test, p<0.05). (F) The relationship of neuron threshold with CP value for coherent stimuli and brightness stimuli, respectively.
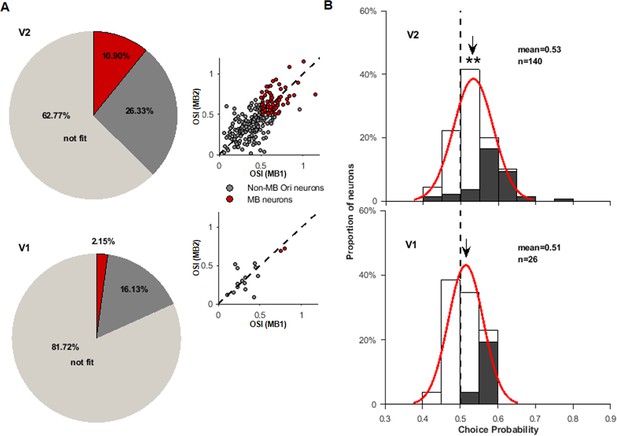
Comparison of V1 and V2 responses to motion boundary (MB) stimuli.
(A) Two pie charts show the percentages of neurons in the recorded V2 (above) and V1 (below) neurons. Light gray represents neurons whose responses could not be fitted (R²<0.7) by the von Mises function (‘not fit’). Dark gray represents neurons that had their responses well fitted (R²≥0.7) but either had low orientation selectivity indices (<0.5) or the two preferred MB orientations did not match (differed by >30°). Red section represents MB neurons. On the right side of each pie chart, neurons’ orientation indices for MB1 and MB2 stimuli are plotted. The colors are consistent with those in the pie charts, and dashed lines are the diagonal lines. (B) The distributions of choice probabilities (CPs) for all measured V2 (top) and V1 (bottom) neurons (including MB and non-MB neurons). For non-MB neurons, the preferred choice was based on their preferred orientations for gratings. Filled bars represent neurons having CP significantly different from 0.5.

Comparison of receptive fields (RFs) of V1 and V2 neurons.
(A) The spatial relationship between the stimuli and RFs of non-motion boundary (MB) V2 neurons. Dots represent RF centers, and the gray bars represent the sizes of RFs in x (horizontal) and y (vertical) dimensions. The dashed circle represents the 4° stimulus patch. This panel is the same as Figure 1—figure supplement 1C. (B) Similar to (A) but for V2 MB neurons. This panel is the same as Figure 1—figure supplement 1D. (C) RF size distributions for MB and non-MB V2 neurons. This panel is the same as Figure 1J. The mean RF size for all V2 neurons was 2.47° ± 0.85°. (D) Distributions of distances between RF centers and the center of the stimuli for MB and non-MB V2 neurons. (E–H) Similar to (A–D) but for V1 neurons. The mean RF size for all V1 neurons was 2.5° ± 1.18°.

Choice probability (CP) distributions of motion boundary (MB) and non-MB neurons in V1 and V2.
(A) CP distribution for all 32 MB neurons in V2 (same as Figure 2H inset), in which 15 neurons had CPs significantly larger than 0.5 (filled bars). The arrow indicates the mean CP (0.56), which is larger than 0.5 (t-test, p<0.001). (B) CP distribution for all 108 non-MB neurons in V2, in which 34 neurons had CPs significantly larger than 0.5 (filled bars). The arrow indicates the mean CP (0.53), which is larger than 0.5 (t-test, p<0.001). (C) CP distribution for all two MB neurons in V1, in which one neuron had CP significantly larger than 0.5 (filled bar). (D) CP distribution for all 24 non-MB neurons in V1, in which five neurons had CPs significantly larger than 0.5 (filled bars). The arrow indicates the mean CP (0.51), which is not different from 0.5 (t-test, p=0.2098).
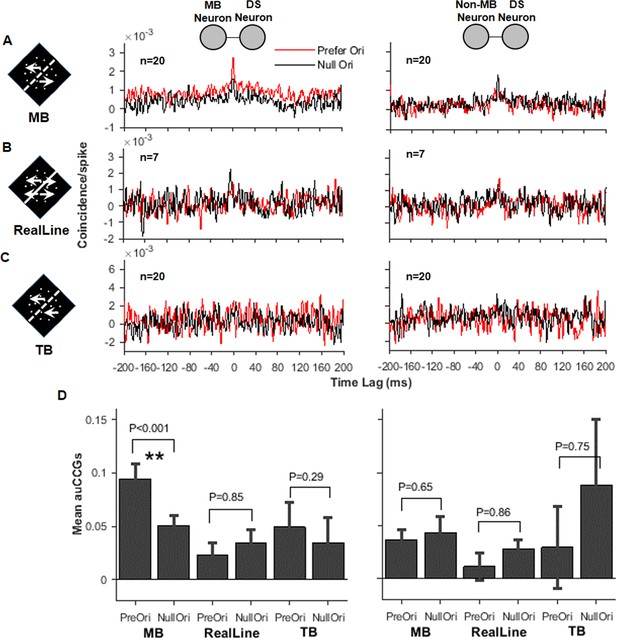
Correlated activity between the direction-selective (DS) and motion boundary (MB) neurons in V2.
(A) Cross-correlograms (CCGs) for MB–DS pairs (left) and non-MB–DS pairs (right) in MB stimulus conditions. Red curves are for preferred orientations and black for null orientations. For non-MB neurons, MB stimuli were oriented along their preferred and null orientations for gratings. (B) Similar to (A), CCGs for real-line conditions. (C) Similar to (A) and (B), CCGs for temporal boundary conditions. (D) Accumulated CCGs for all stimulus conditions shown in (A–C). Only the MB–DS pairs in the MB conditions exhibited significantly higher correlation for the preferred orientation than the null one (t-test, p<0.001). Error bar: SEM.
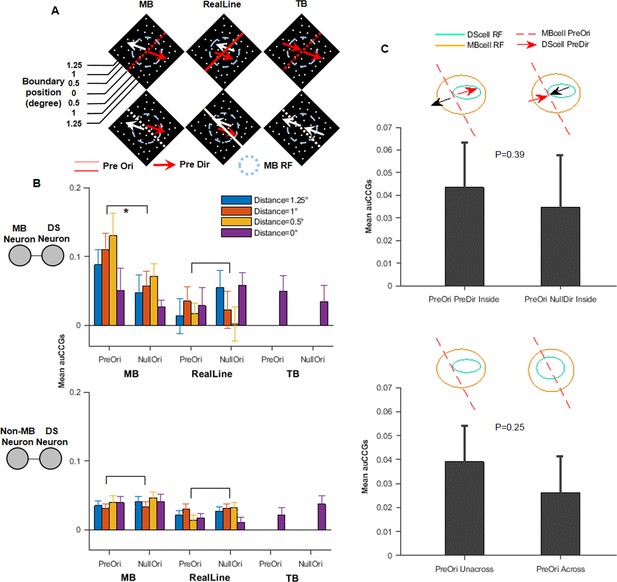
Additional information for the correlation tests.
(A) Illustrations of the three types of stimuli tested different boundary positions. (B) Similar to Figure 4D, with accumulative cross-correlograms (auCCGs) from different boundary positions plotted separately. Results from symmetrical positions were averaged. For temporal boundary stimuli, only one position was tested. (C) Top: average auCCG values for two stimulus conditions: random dot moving along the direction-selective (DS) neurons’ preferred direction (left bar) or, null direction (right bar), motion boundary (MB) was placed bordering the receptive field (RF) of the DS neurons. Although the average auCCG shows some difference, it is not statistically significant (paired t-test, p=0.39). Bottom, average auCCG values for two stimulus conditions: MB was placed bordering the RF of the DS neurons (left bar) or across the RF center (right bar). The ‘bordering’ condition had a higher but not significant value compared to the ‘center’ one (t-test, p=0.25).
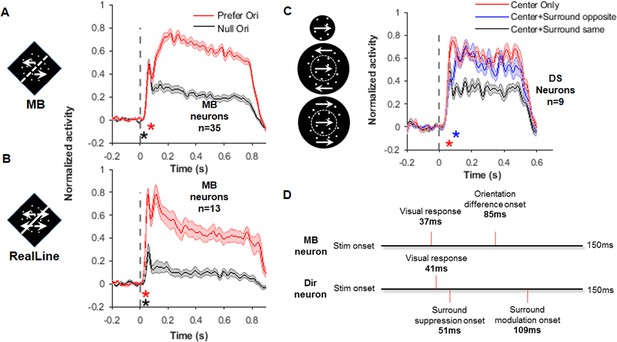
Response time courses of the motion boundary (MB) and direction-selective (DS) neurons.
(A) Average response PSTHs for the MB neurons to the MB stimuli presented at their preferred (red) and null orientations. The black asterisk indicates the time for visual response delay (37 ms), which was similar for these two stimulus conditions. This value was also similar to that in non-MB neurons for the same stimuli (39 ms, see Figure 5—figure supplement 1A). The red asterisk indicates the time point (85 ms) where responses to the preferred and null orientations started to show differences (i.e., MB orientation time). Error shading represents SEM, same for below. (B) Average response PSTHs for the MB neurons to the real-line stimuli. The two asterisks indicate the visual response delay (37 ms, black asterisk) and the orientation-selective response time (35 ms, red asterisk), respectively. Non-MB neurons’ responses to the real line were similar (Figure 5—figure supplement 1B). (C) Average response PSTHs for DS neurons in responding to three different stimuli: center only (red), center and surround with the same direction (black), and center and surround with opposite directions (blue). The center random dots were always moving at the DS cell’s preferred direction in these three conditions. The red asterisk indicates time when the ‘center surround same’ responses start to different from the ‘center-only’ responses. The blue asterisk indicates the time where the ‘center surround opposite’ responses started to different from the ‘center surround same’ responses. (D) A summary of time points shown in (A–C). The time MB neurons start to show MB orientation selectivity (85 ms) falls in the time range where surround effects emerge in the DS neurons.
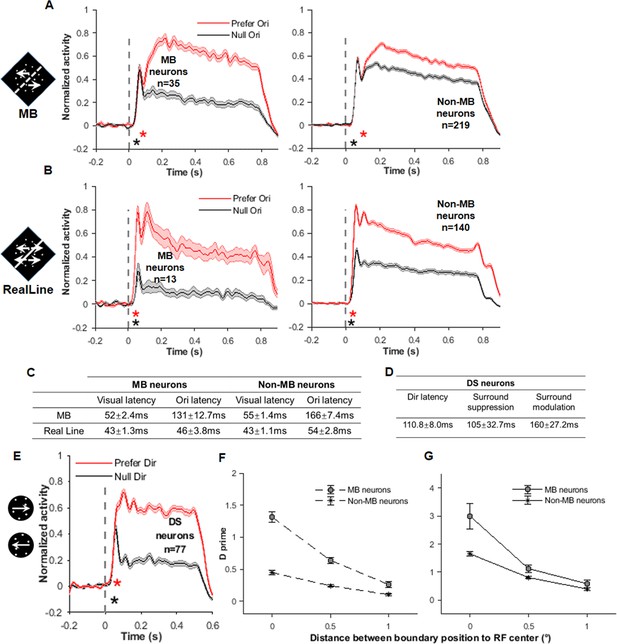
Additional information for response delay.
(A) Comparison of response time courses of motion boundary (MB) neurons (left, same as in Figure 5A) and non-MB neurons (right) when they were responding to MB stimuli. (B) Comparison of response time courses of MB neurons (left, same as in Figure 5A) and non-MB neurons (right) when they were responding to real-line stimuli. (C) A summary table of delay times shown in (A) and (B). To compare with the delays measured with population method described in the main text, here we calculated the delays for each neuron and averaged. We found that delays calculated from the two methods were consistent. (D) A summary table of delay times for the direction-selective (DS) neurons, based on analysis of individual neurons. (E) The response time courses of DS neurons when they were responding to random dots stimuli. The red asterisk indicates the onset time of direction selectivity for DS neurons (49 ms). The black asterisk indicates the visual latency for DS neurons (39 ms, which is similar to that obtained by surround modulation stimuli, e.g., Figure 5D). (F) D' calculated for MB and non-MB neurons in MB stimulus conditions, plotted as a function of the MB positions. (G) D' calculated for MB and non-MB neurons in real-line stimulus conditions, plotted as a function of the MB positions.
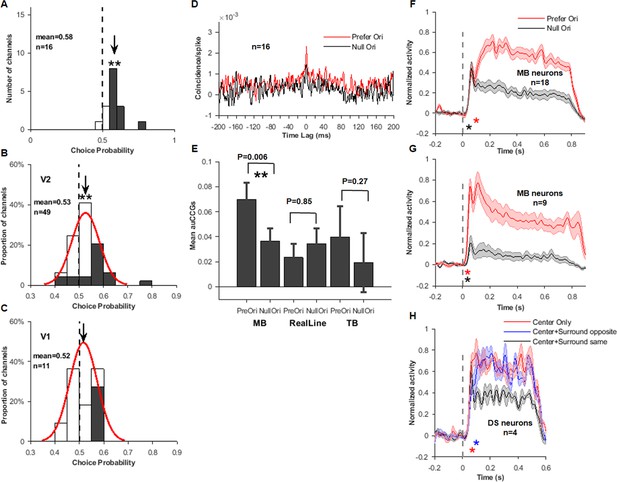
Results from ‘one-cell-per-channel’ dataset.
(A) Choice probability (CP) distribution for V2 motion boundary (MB) neurons (corresponding to inset plot in Figure 2H). (B) CP distribution for V2 MB and non-MB neurons (corresponding to Figure 3B top panel). (C) CP distribution for V1 MB and non-MB neurons (corresponding to Figure 3B bottom panel). (D) Cross-correlogram (CCG) for MB neurons responding to MB stimuli (corresponding to Figure 4A left panel). (E) Accumulative cross-correlogram (AuCCG) for MB neurons responding to six stimulus conditions (corresponding to Figure 4D left panel). (F) Response time courses of MB neurons to MB stimuli (corresponding to Figure 5A). (G) Response time courses of MB neurons to real-line stimuli (corresponding to Figure 5B). (H) Response time courses of direction-selective neurons (corresponding to Figure 5C).
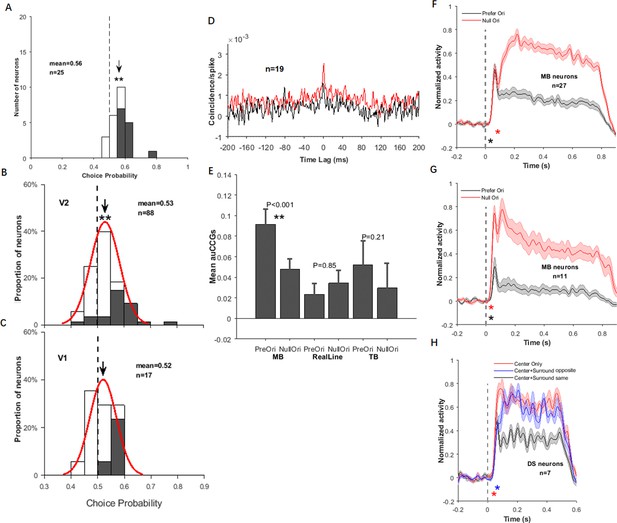
Main results analyzed based-on ‘unique-units’ dataset.
(A) Choice probability (CP) distribution for V2 motion boundary (MB) neurons (corresponding to inset plot in Figure 2H). (B) CP distribution for V2 MB and non-MB neurons (corresponding to Figure 3B top panel). (C) CP distribution for V1 MB and non-MB neurons (corresponding to Figure 3B bottom panel). (D) Cross-correlogram (CCG) for MB neurons responding to MB stimuli (corresponding to Figure 4A left panel). (E) Accumulative cross-correlogram (AuCCG) for MB neurons responding to six stimulus conditions (corresponding to Figure 4D left panel). (F) Response time courses of MB neurons to MB stimuli (corresponding to Figure 5A). (G) Response time courses of MB neurons to real-line stimuli (corresponding to Figure 5B). (H) Response time courses of direction-selective neurons (corresponding to Figure 5C).

Two alternative direction-selective to motion boundary (DS-to-MB) models in which surround suppression of DS neurons plays different roles.
Both models show the spatial relationship between a MB (dashed line) and the receptive fields of DS and MB neurons (circles). The DS neurons have a center-surround organization, which prefers different motion directions (small arrows). Model 1: the MB runs across the edge between the center and surround of the DS receptive field. Similar to the center-surround mechanism in detection of various types of boundaries (e.g., luminance, color, disparity), here boundary detection relies on the center-surround mechanism of the DS neurons, which detect the motion contrast at the MB. Previous work has shown that, in such conditions, DS neurons are activated optimally (Figure 5 in Hu et al., 2018). In addition, the precise location of the MB is detected at the same time. Model 2: MB detection is achieved by comparing the activation of two DS neurons based on their different preferred directions. Thus, surround suppression plays a less significant role in this model. Although this model is also applicable, it has been shown that DS neurons are suboptimally activated in such conditions (Figure 5 in Hu et al., 2018) and a precise MB location is unavailable. Thus, we mainly considered Model 1 in the present work.
Videos
Motion boundary (MB) stimulus for fixation task.
The video shows the MB stimuli used to test neurons’ MB orientation tuning during the fixation tasks. A MB stimulus is shown in two orthogonal orientations, each having two conditions in which the moving direction of the dots on the two sides was switched.
Motion boundary (MB)-position stimuli.
The video shows the MB-position stimuli used to test MB–DS correlation during the orientation-discrimination tasks. A MB was presented at different orientations and positions. DS: direction-selective.
Real-line position stimuli.
The video shows the real-line stimuli as control for the motion boundary-position stimuli.
Temporal boundary (TB) stimuli.
The video shows the TB stimuli used as a control for the motion boundary-position stimuli.
Tables
Reagent type (species) or resource | Designation | Source or reference | Identifiers | Additional information |
---|---|---|---|---|
Strain, strain background (macaque, male) | Macaca mulatta | Suzhou Xishan Zhongke animal Company, Ltd | NCBITaxon:9544 | http://xsdw.bioon.com.cn/ |
Software, algorithm | MATLAB-R2018b | MathWorks | SCR_001622 | R2018b |
Software, algorithm | Codes and ‘all cell’ dataset | This paper | N/A | http://dx.doi.org/10.17632/fjy37kc8pd.3 |
Software, algorithm | Codes and unique-unit dataset | This paper | N/A | http://dx.doi.org/10.17632/fjy37kc8pd.3 |
Software, algorithm | Codes and ‘one-cell-per-channel’ dataset | This paper | N/A | http://dx.doi.org/10.17632/fjy37kc8pd.3 |
Other | 32-channel-array | Blackrock Microsystems, LLC | N/A | https://www.blackrockmicro.com/ |
Other | 64-channel Multichannel Neural Recording (AlphaLab SNR) | Alpha Omega | N/A | https://www.alphaomega-eng.com/ |
Other | Imager 3001 (Optical Imaging) | Optical Imaging Ltd | N/A | https://optimaging.com/ |
Other | Eyelink Desktop(eyelink 1000) | SR Research | N/A | https://www.srresearch.com/ |
V1 | V2 | |
---|---|---|
MB neurons | 2 | 70 |
Well fitted (R²≥0.7), but OSI<0.5 or two preferred MB orientation were different (>30°); | 15 | 169 |
Can not fit (R²<0.7) | 76 | 403 |
Additional files
-
Supplementary file 1
Number of V2 electrodes (and neurons) from which motion boundary neurons were recorded.
- https://cdn.elifesciences.org/articles/61317/elife-61317-supp1-v3.docx
-
Supplementary file 2
Number of V2 electrodes (and neurons) from which choice probability was calculated.
Note: The right hemisphere array in monkey W had a low electrode number since two thirds of its electrodes were located in V1.
- https://cdn.elifesciences.org/articles/61317/elife-61317-supp2-v3.docx
-
Transparent reporting form
- https://cdn.elifesciences.org/articles/61317/elife-61317-transrepform-v3.docx