Glycinergic axonal inhibition subserves acute spatial sensitivity to sudden increases in sound intensity
Figures
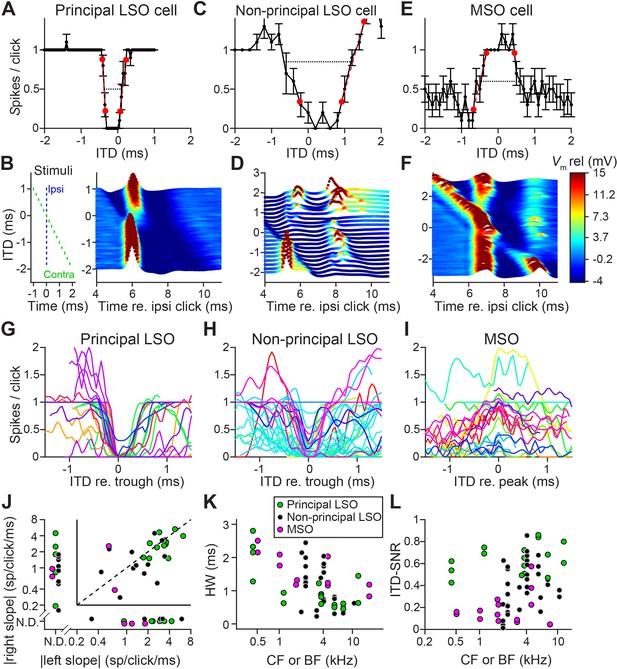
Sharp ITD-sensitivity to clicks in LSO but not MSO.
(A) Click-ITD function (10 repetitions) of an LSO principal cell (CF = 5.7 kHz). Data are represented as mean ± SEM. Red circles indicate ITD values near the trough when the spike rate reached 20% or 80% of the maximum. Black dotted line indicates halfwidth of the central trough. By convention, negative ITD refers to the ipsilateral click leading the contralateral one, and vice versa for positive ITD. (B) Waterfall plots of the intracellular response of the cell in A. The membrane potential, averaged per ITD value across 10 repetitions, is color-coded. For clarity and to aid comparison with D and F, colors correspond to values clipped between the limits shown in the color scale in F. Inset on left indicates timing of the contralateral click (green) relative to the ipsilateral click (blue) for different ITDs. Leading or lagging inhibition does not affect the EPSP sufficiently to inhibit spiking, except over a small time window at ITDs near 0 ms. (C–F) Similar to A and B, for a non-principal LSO cell (C and D; CF = 4.1 kHz), and for an MSO neuron (E and F; CF = 4.6 kHz). In MSO, an excitatory response is present to clicks from either ear: there is some modulation of spike rate but it never decreases to 0. Red circles in E indicate ITD values where the spike rate reached 20% or 80% of the maximum. Black dotted line indicates halfwidth of the central peak. (G–I) Population of ITD functions for identified principal LSO neurons (G: 24 data sets, 8 cells), non-principal LSO neurons (H: 38 data sets, 11 cells), and MSO neurons (I: 25 data sets, 11 cells; 6 out of 11 cells were anatomically verified). To reduce clutter, tuning functions were aligned at the most negative ITD value of trough (G and H) or peak (I). Different colors indicate different cells. (J) Steepness of the slope (measured at 20% and 80% points) to the right of the central trough (for LSO cells) or peak (for MSO cells) plotted against steepness of the slope to the left of the central trough or peak. Abscissa and ordinate are scaled logarithmically. N.D.: data points for which either the left slope or the right slope is not defined because spike rate did not reach the respective threshold (e.g. the right slope of the MSO cell in E). Data sets for which both left and right slopes were not defined are not shown (LSO principal: one data set; LSO non-principal: nine data sets; MSO: 10 data sets). Only cells for which the trough was lower than 0.5 sp/click (LSO) or the peak was higher than 0.5 sp/click (MSO) were included. Principal LSO (green): 23 data sets, 8 cells; Non-principal LSO (black): 27 data sets, 8 cells; MSO (magenta): seven data sets, 4 cells. (K) Halfwidth of the central peak or trough as a function of CF or BF. Abscissa is scaled logarithmically. Only cells for which the trough was lower than 0.5 sp/click (LSO) or the peak was higher than 0.5 sp/click (MSO) were included. Principal LSO: 17 data sets, 8 cells; Non-principal LSO: 26 data sets, 9 cells; MSO: 11 data sets, 6 cells. (L) ITD-SNR (Hancock et al., 2010) as a function of CF or BF. Abscissa is scaled logarithmically. Principal LSO: 16 data sets, 7 cells; Non-principal LSO: 34 data sets, 9 cells; MSO: 16 data sets, 7 cells. Legend in K applies also to J and L. Numerical data represented as graphs in this figure are available in a source data file (Figure 1—source data 1).
-
Figure 1—source data 1
Excel table with data represented in this figure.
- https://cdn.elifesciences.org/articles/62183/elife-62183-fig1-data1-v2.xlsx
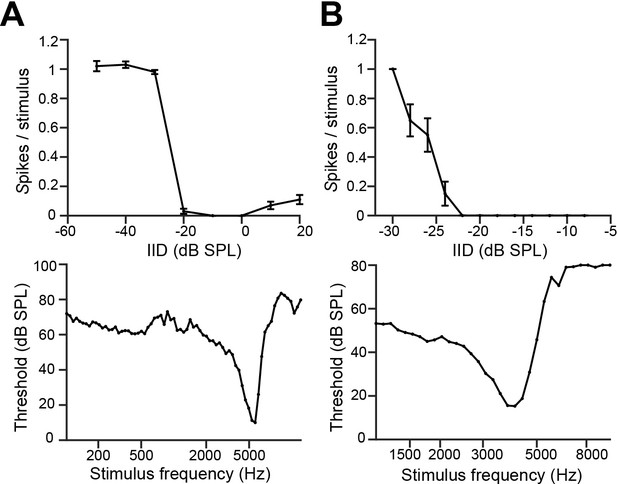
Physiological data of LSO cells in Figure 1.
(A) Top: IID function to 5.7 kHz tones for the same principal LSO neuron as in Figure 1A. Data are represented as mean ± SEM. Ipsilateral sound level was kept constant at 60 dB SPL. Stimulus duration: 25 ms. By convention, positive IIDs refer to contralateral sound levels exceeding the ipsilateral sound level. Bottom: frequency threshold tuning function to ipsilateral short tones. CF is 5.7 kHz with threshold 10 dB SPL. Note that abscissa is scaled logarithmically. (B) Top: IID function to 4.1 kHz tones for the same non-principal LSO neuron as in Figure 1C. Data are represented as mean ± SEM. Ipsilateral sound level was kept constant at 70 dB SPL. Stimulus duration: 50 ms. Bottom: frequency threshold tuning function to ipsilateral short tones. CF is 4.1 kHz with threshold 15 dB SPL. Numerical data represented as graphs in this figure are available in a source data file (Figure 1—figure supplement 1—source data 1).
-
Figure 1—figure supplement 1—source data 1
Excel table with data represented in this figure.
- https://cdn.elifesciences.org/articles/62183/elife-62183-fig1-figsupp1-data1-v2.xlsx
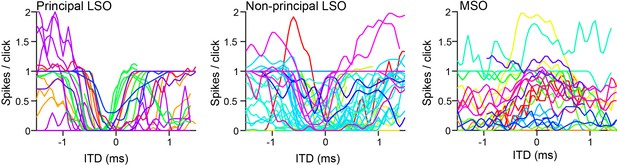
Population data of ITD functions of Figure 1G–I, without centering the left flank of the central trough (LSO) or peak (MSO) at 0 ms.
Numerical data represented as graphs in this figure are available in a source data file (Figure 1—figure supplement 2—source data 2).
-
Figure 1—figure supplement 2—source data 2
Excel table with data represented in this figure.
- https://cdn.elifesciences.org/articles/62183/elife-62183-fig1-figsupp2-data2-v2.xlsx
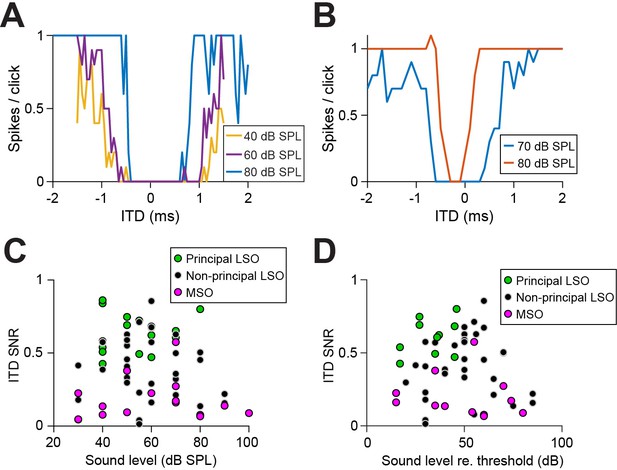
ITD-sensitivity to clicks at different sound levels.
(A,B) For two principal LSO neurons, click ITD functions at different monaural sound levels are shown (IID = 0). Higher sound levels result in steeper slopes and narrower halfwidths. (A) BF = 437 Hz; (B) CF = 12 kHz. (C,D) Monaural sound levels (C) or sound levels relative to the cell’s ipsilateral threshold to clicks (D) do not explain the differences in ITD-SNR between cell types. (C) LSO principal cells: 16 data sets (7 cells); LSO non-principal cells: 34 data sets (9 cells); MSO: 16 data sets (7 cells). (D) LSO principal cells: 10 data sets (5 cells); LSO non-principal cells: 32 data sets (8 cells); MSO: 12 data sets (5 cells). Numerical data represented as graphs in this figure are available in a source data file (Figure 1—figure supplement 3—source data 3).
-
Figure 1—figure supplement 3—source data 3
Excel table with data represented in this figure.
- https://cdn.elifesciences.org/articles/62183/elife-62183-fig1-figsupp3-data3-v2.xlsx
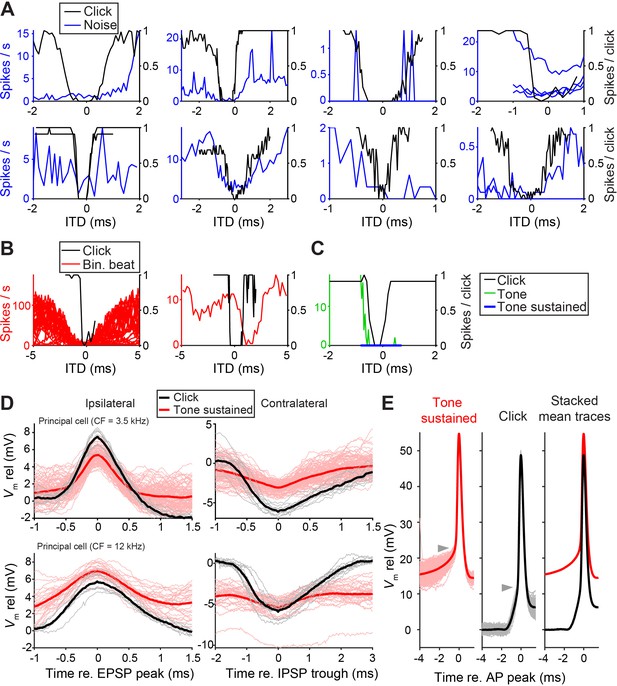
ITD-sensitivity to clicks is steeper than to sustained sounds for LSO cells.
(A) ITD functions to noise (blue) and clicks (black) for 8 LSO neurons. Noise ITD functions include all spikes beyond 10 ms after stimulus onset. Top row are data from juxtacellular recordings, bottom row are data from whole-cell recordings. CFs are respectively (top row, left to right): 3.5 kHz; 7.0 kHz; 3.7 kHz; 2.7 kHz and (bottom row, left to right): 5.7 kHz; 3.5 kHz; 7.5 kHz; 2.3 kHz. Curves are scaled to the maximum response. (B) ITD function to sustained tones and click ITD function for 2 LSO cells. Left panel: tonal ITD functions (red) are derived to binaural beats in the envelope of sounds amplitude-modulated at various frequencies. There was no ITD sensitivity to binaural beats of stimulus fine structure (not shown). CF = 2.7 kHz. Right panel: tonal ITD function from binaural beat (ipsi ear 100 Hz, contra ear 101 Hz, 90 dB SPL; BF = 437 Hz). (C) Click ITD function and ITD function to static tones at CF showing all elicited spikes (green) or all spikes beyond sound onset (blue) for an LSO principal cell (CF = 12 kHz). (D) Largest EPSPs (left column) and largest IPSPs (right column) per trial for monaural clicks and the sustained part of monaural tones at CF (>10 ms after onset), for two principal LSO cells. Thick lines represent means. The data is taken from sound levels that result in similar spike rates when the stimuli are presented ipsilaterally. EPSPs that lead to action potentials are not included. (E) Action potentials elicited during the sustained part of a tone at CF (left panel) or by clicks (middle panel), for a non-principal LSO cell (CF = 7.5 kHz). Thick lines are mean traces, which are plotted on top of each other in the right panel. Triangles indicate approximate action potential voltage threshold, which decreases with steeper EPSP slopes. Numerical data represented as graphs in this figure are available in a source data file (Figure 1—figure supplement 4—source data 4).
-
Figure 1—figure supplement 4—source data 4
Excel table with data represented in this figure.
- https://cdn.elifesciences.org/articles/62183/elife-62183-fig1-figsupp4-data4-v2.xlsx
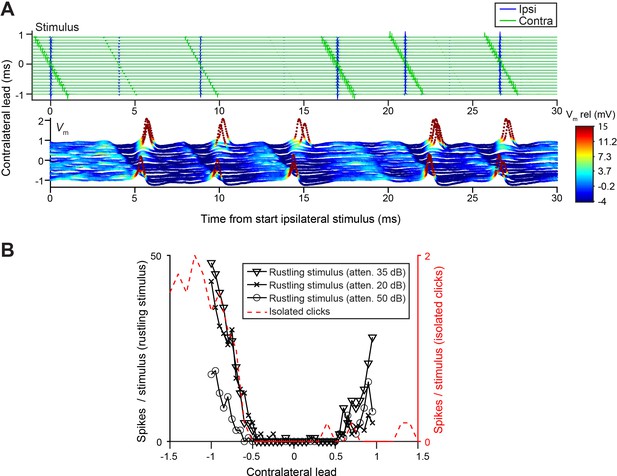
Steep ITD-sensitivity to transients extends to rustling stimuli.
(A) Bottom panel: waterfall plot of responses to a rustling stimulus, an irregular succession of transients designed to mimic natural sounds such as crumpling of leaves (Ewert et al., 2012), from a principal LSO neuron (CF = 3.75 kHz). The membrane potential, averaged per ITD value is color-coded. For clarity values are clipped between the limits shown in the color scale. Top panel: ipsilateral (blue) and contralateral (green) stimulus, shown for different ITD values along the ordinate. Only first 30 ms of stimulus are shown. (B) ITD functions to rustling stimuli at different attenuations for the same cell as in A, as well as an ITD function to single click pairs. Numerical data represented as graphs in this figure are available in a source data file (Figure 1—figure supplement 5—source data 5).
-
Figure 1—figure supplement 5—source data 5
Excel table with data represented in this figure.
- https://cdn.elifesciences.org/articles/62183/elife-62183-fig1-figsupp5-data5-v2.xlsx
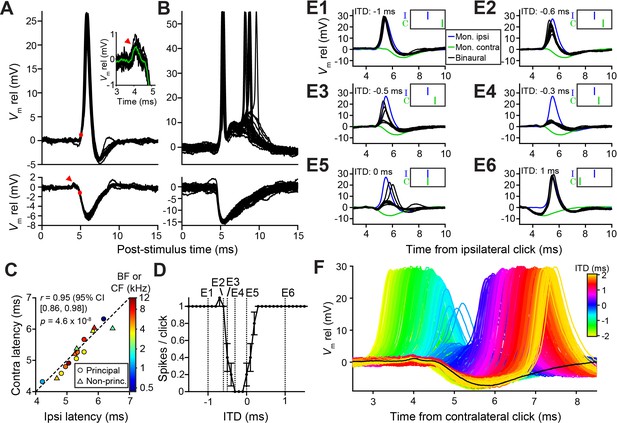
Precise timing and interaction of IPSPs and EPSPs in LSO neurons.
(A–B) Example responses to ipsilateral clicks (top panels) and contralateral clicks (bottom panels) for a principal LSO cell (A; CF = 12 kHz; 50 dB SPL; 10 repetitions) and a non-principal LSO cell (B; CF = 4.1 kHz; 20 dB SPL; 30 repetitions). Red arrowhead indicates prepotential, shown with a blowup in inset. (C) Ipsilateral versus contralateral latency of postsynaptic potentials evoked by monaural clicks for nine principal cells (circles) and six non-principal LSO cells (triangles). Color indicates CF or BF for each cell. Latency was defined as the time relative to click onset when the membrane potential crossed a voltage difference relative to rest with an absolute value equal to 20% of the IPSP amplitude. For the ipsilateral response, this voltage difference was a depolarization (red dot in top panel in A), for the contralateral response this voltage difference was a hyperpolarization (red dot in bottom panel in A). For this analysis, we used the response to identical sound levels for ipsi and contra, at the lowest level generating a maximal monaural ipsilateral response. (D) Click-ITD function for the same neuron as in A. Data are represented as mean ± SEM. Sound level 60 dB SPL at both ears. Dotted vertical lines correspond to the ITD values of the panels in E. (E1–E6) Average responses to monaural ipsilateral (blue) and monaural contralateral (green) clicks are compared to binaural responses (black) for the ITD values indicated by dotted vertical lines in D. (F) Data from the same principal LSO cell as in A,D,E. Colored lines: responses to click pairs of different ITDs, referenced in time to the contralateral (inhibitory) click. Black line: averaged response to contralateral clicks at the same sound level as in D and E (corresponding to green line in E). Numerical data represented as graphs in this figure are available in a source data file (Figure 2—source data 1).
-
Figure 2—source data 1
Excel table with data represented in this figure.
- https://cdn.elifesciences.org/articles/62183/elife-62183-fig2-data1-v2.xlsx
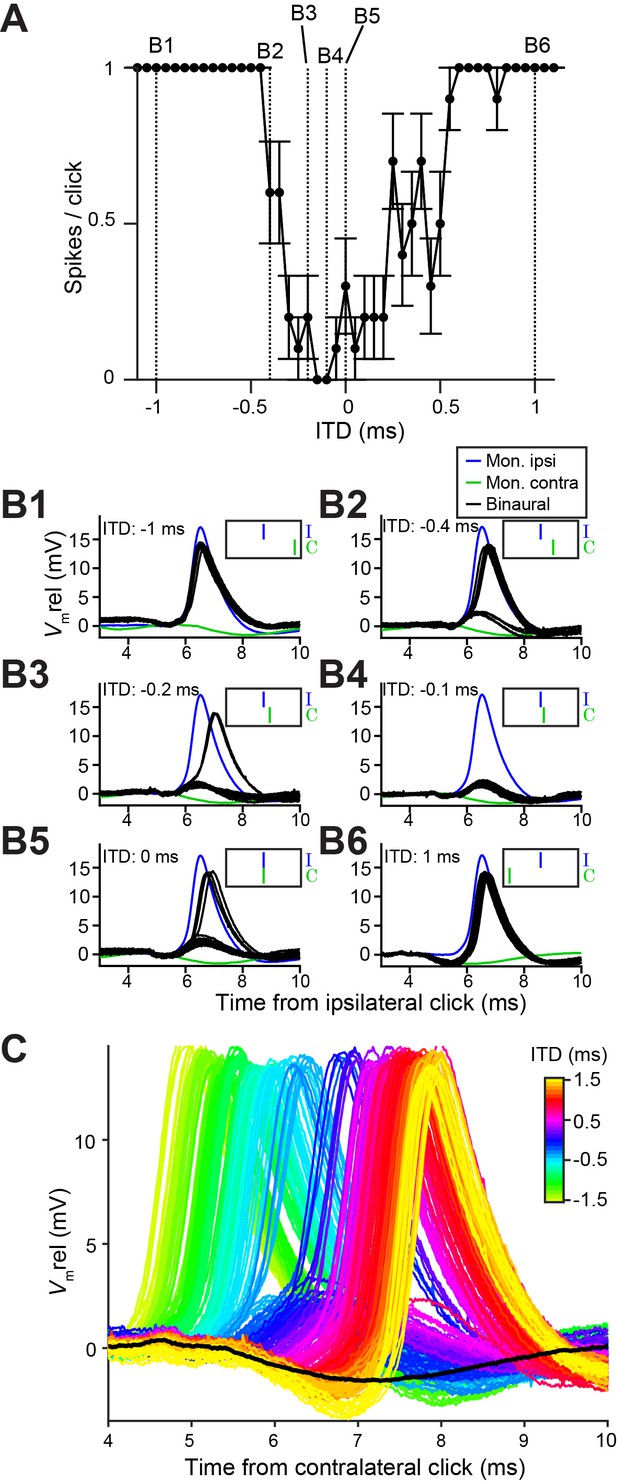
Precise interaction of IPSPs and EPSPs for another principal LSO neuron.
(A–C) Similar to Figure 2D–F, for another principal LSO neuron (CF = 7.5 kHz). Numerical data represented as graphs in this figure are available in a source data file (Figure 2—figure supplement 1—source data 1).
-
Figure 2—figure supplement 1—source data 1
Excel table with data represented in this figure.
- https://cdn.elifesciences.org/articles/62183/elife-62183-fig2-figsupp1-data1-v2.xlsx
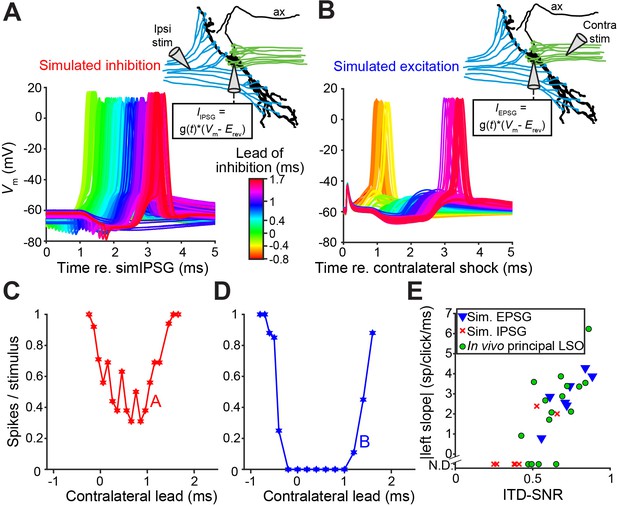
In vitro recordings reveal powerful inhibition for synaptically evoked but not for simulated IPSPs.
(A) Voltage responses from a principal LSO neuron recorded in a brain slice, for which the ipsilateral inputs were activated by electric shocks and the contralateral input was simulated by somatically injecting inhibitory conductances (IPSG) via dynamic clamp. Inset indicates experimental setup. Delay between ipsilateral shock and IPSG was varied and is referenced to the timing of the simulated IPSG, and all recorded membrane potential traces are shown, color coded for the delay. ax: axon. (B) Similar to A, but with contralateral inputs activated by electric shocks and ipsilateral excitatory conductances simulated via somatic dynamic clamp (different neuron than A). (C) Rate delay function corresponding to the experiment in A. (D) Rate delay function corresponding to the experiment in B. (E) Steepness of the slope to the left of the trough for the population of delay functions (Figure 4—figure supplement 1D and E, solid lines), plotted against the ITD-SNR (as in Figure 1L). Data from principal LSO cells recorded in vivo are shown for comparison (16 data sets from 7 cells). N.D.: not defined (slope was not defined when 20% of maximal spike rate was not reached after smoothing [see Materials and methods]). Numerical data represented as graphs in this figure are available in a source data file (Figure 4—source data 1).
-
Figure 4—source data 1
Excel table with data represented in this figure.
- https://cdn.elifesciences.org/articles/62183/elife-62183-fig4-data1-v2.xlsx

In vitro recordings reveal powerful inhibition for synaptically evoked IPSPs but not for IPSPs simulated by current injection.
(A–B) Similar to Figure 4A and B, but now inhibitory (A) or excitatory (B) synaptic inputs were simulated by injecting currents instead of conductances by dynamic clamp. This experiment was done in every neuron in which the dynamic clamp experiment was done (Figure 4), and the examples shown in A and B are traces for the same neurons as in Figure 4A and B. (C) Similar as Figure 4E, for the current injection experiments in panels A and B. ITD-SNR was substantially larger for principal LSO neurons recorded in vivo than for neurons recorded in vitro for which inhibition was simulated by current injection (respective median [IQR] 0.62 [0.17], 7 neurons and 0.33 [0.10], eight neurons); Mann-Whitney U = 50.0, p=0.009; θ = 0.89 (95% CI [0.60, 0.98]). ITD-SNR was substantially larger for in vitro recordings in which inhibitory inputs were activated synaptically compared to those in which inhibition was simulated by current injection (respective median [IQR] 0.68 [0.26], seven neurons and 0.33 [0.10], eight neurons); Mann-Whitney U = 50.0, p=0.009; θ = 0.89 (95% CI [0.60, 0.98]). (D) Solid lines: rate delay functions for the dynamic clamp experiment shown in Figure 4A, for eight neurons. Dashed lines: rate delay functions for the current injection experiment shown in panel A, for the same neurons. Top left panel corresponds to the neuron shown in Figure 4A and C, and panel A. (E) Solid lines: rate delay functions for the dynamic clamp experiment shown in Figure 4B, for seven neurons. Dashed lines: rate delay functions for the current injection experiment shown in panel B, for the same neurons. Top left panel corresponds to the neuron shown in Figure 4B and D, and panel B. Numerical data represented as graphs in this figure are available in a source data file (Figure 4—figure supplement 1—source data 1).
-
Figure 4—figure supplement 1—source data 1
Excel table with data represented in this figure.
- https://cdn.elifesciences.org/articles/62183/elife-62183-fig4-figsupp1-data1-v2.xlsx
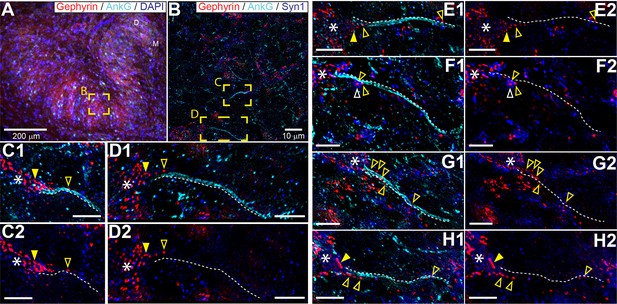
Glycinergic innervation of the axon initial segment of LSO neurons.
(A) An example of an LSO in a coronal tissue section from the Mongolian gerbil outlining subregions targeted for SR-SIM microscopy labeled with gephyrin (red), ankyrinG (cyan), and DAPI (blue). All imaging was targeted to the middle bend of the LSO. (B) An example SR-SIM multichannel image labeled for gephyrin (red), ankyrinG (cyan), and synaptophysin1 (blue). Yellow boxes indicate axon initial segments (AIS) shown in C (mirrored from B) and D. (C–H) Images showing example LSO AISs with (1) or without (2) labels for ankyrinG channel (cyan). White dotted lines lay adjacent to labeled AIS for visual guidance, but are not quantitatively drawn. Putative inhibitory terminals can be seen closely associated with the AIS (open yellow arrowheads) and axon hillock (filled yellow arrowheads). Some large putative gephyrin positive terminals show colocalization with synaptophysin1 labeling (open white arrowheads). White asterisks indicate the soma/dendrite from which the AIS emerges. All scale bars are 5 μm, unless noted otherwise.
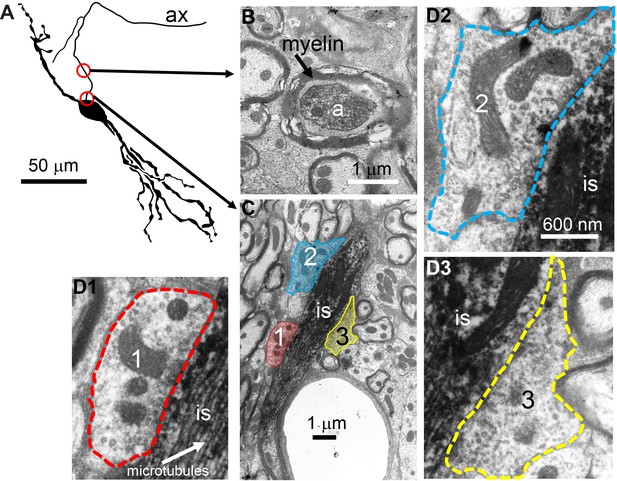
Electron microscopy reveals synaptic terminals on an LSO principal cell’s axon initial segment.
(A) Camera lucida drawing of an LSO principal cell that was intracellularly recorded from and labeled, in vivo. This cell corresponds to cell two in Franken et al., 2018, their Figure 2A. Arrows point to electron micrographs that show portions of the axon (ax) enclosed by the red circles. (B) Electron micrograph showing portion of the axon in the top circle in A. The axon is myelinated here. (C) Electron micrograph showing portion of the axon in the bottom circle in A. This is at the level of the axon initial segment (is). Enclosed colored areas 1–3 represent axon terminals synapsing on the axon initial segment. (D1–D3) Electron micrographs showing larger versions of axon terminals 1–3 in C. Scale bar in D2 applies to all three enlarged micrographs.
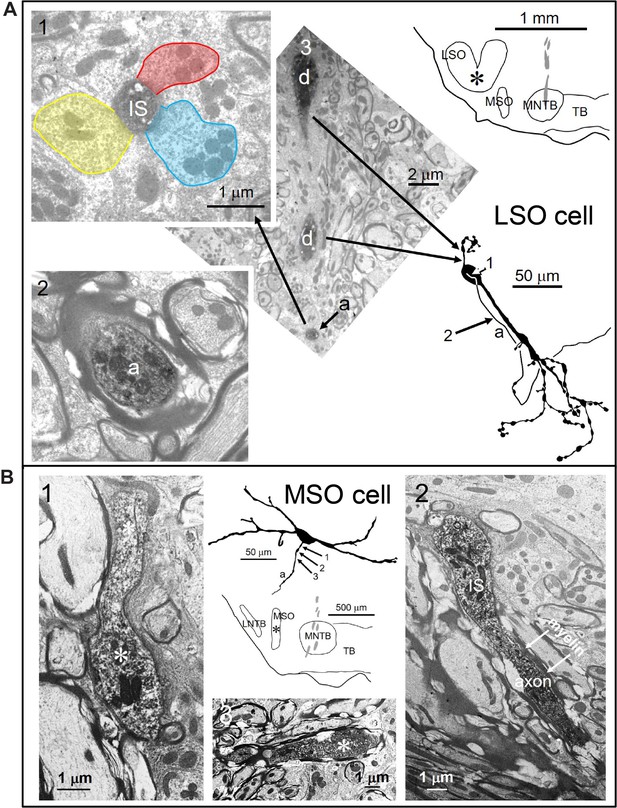
Electron microscopy reveals synaptic terminals on the axon initial segment of principal LSO cells but not of principal MSO cells.
(A) Right: camera lucida drawing of an LSO principal cell that was intracellularly recorded from and labeled in vivo, and its location in a coronal section of the LSO. Numbers and arrows point to locations on the axon shown in the electron micrographs with the same numbers. (1) illustrates the initial segment (IS) close to the cell body and three synapses colored for clarity, (2) the myelinated axon approximately 70 µm from the cell body, and (3) two pieces of the dendrite (d) at the locations pointed to by the arrows as well as the axon (a) initial segment seen enlarged in 1. Scale bar in 1 applies to 1 and 2. In addition to this cell and the one shown in Figure 6, we observed similar synaptic terminals in a third LSO principal cell (data not shown). (B) Absence of innervation of the initial segment of an MSO principal cell. Top middle: camera lucida drawing of an MSO principal cell that was intracellularly recorded from and labeled in vivo, and its location in a coronal section of the MSO. Numbers and arrows point to locations on the axon shown in the electron micrographs with the same numbers. 1: illustrates the initial segment (asterisk) close to the cell body, 2: the transition from initial segment to myelinated axon approximately 20 µm from the cell body, and 3: the myelinated axon approximately 50 µm from the cell body. A second MSO principal cell that was processed for EM also did not show innervation of the axon initial segment (data not shown).
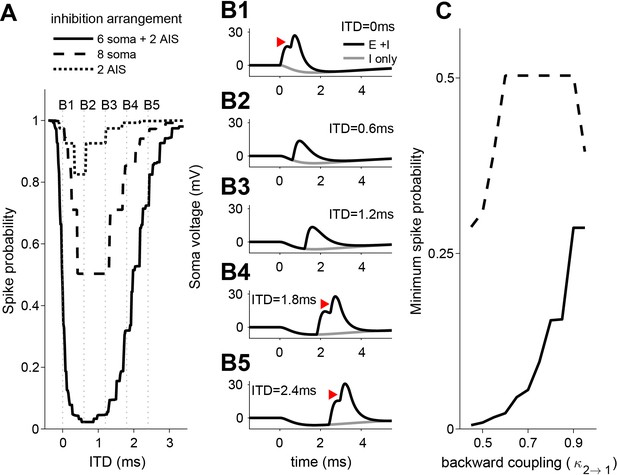
ITD-tuning in a two-compartment LSO neuron model.
(A) ITD-tuning is substantially deeper when inhibitory inputs target AIS as compared to the same total number of inputs targeting the soma only. (B) Soma voltage showing detailed timing of responses to excitatory and inhibitory inputs. All synaptic inputs are activated in these simulations. Inhibition arrangement is six soma and two AIS inputs. Coupling configuration in A and B is = 0.95 and = 0.6. Red arrowheads mark action potentials (backpropagated from initiation site in the AIS compartment). Voltage is expressed relative to rest. (C) More pronounced suppression of spike rates when inhibitory synapses are added to the AIS rather than to the soma occurs for a range of backward coupling values. Inhibition arrangement for the two functions is indicated in the legend in A. Forward coupling in all simulations is = 0.95. Numerical data represented as graphs in this figure are available in a source data file (Figure 7—source data 1).
-
Figure 7—source data 1
Excel table with data represented in this figure.
- https://cdn.elifesciences.org/articles/62183/elife-62183-fig7-data1-v2.xlsx
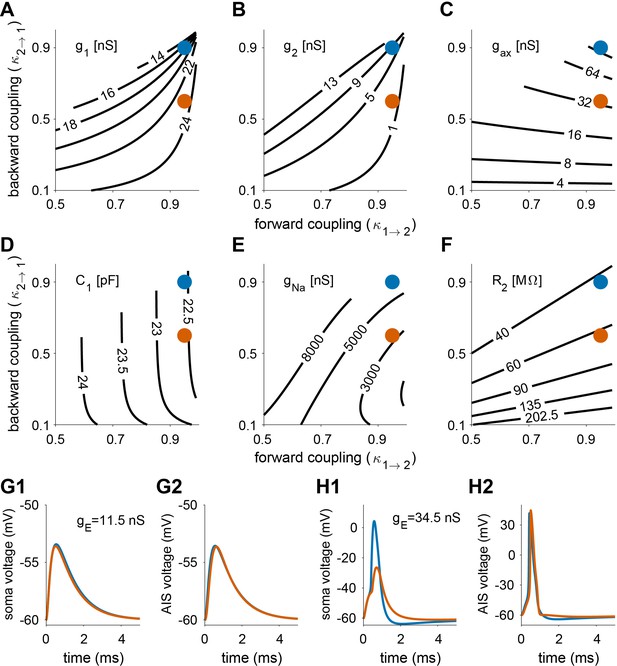
Two-compartment LSO neuron model.
(A–E) Model parameters depend on coupling constants. Each coupling constant pair () defines a model with soma voltage dynamics constructed to match known properties of LSO neurons. (F) AIS input resistance exceeds soma input resistance under the assumption that forward coupling is stronger than backward coupling. (G) Nearly identical subthreshold voltage responses exhibited for two coupling configurations ( for blue, for red). Parameter values for these two models are marked as dots in all previous panels. (H) Spike shape in soma and AIS differs in the two models.
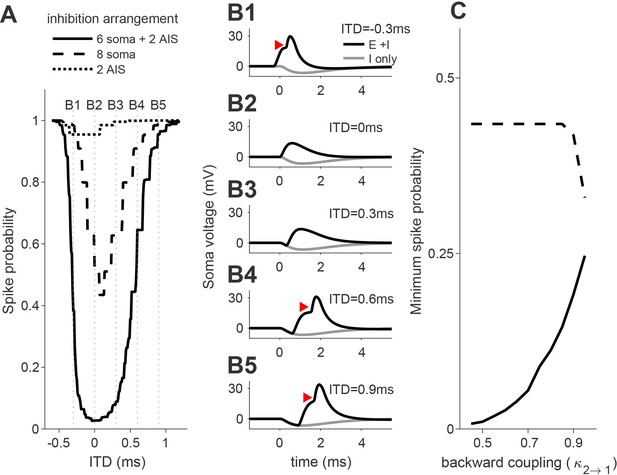
ITD tuning in two-compartment LSO neuron model with synaptic kinetics adapted from Beiderbeck et al., 2018.
See Materials and methods for details. Figure layout same as Figure 7. Numerical data represented as graphs in this figure are available in a source data file (Figure 7—figure supplement 2—source data 1).
-
Figure 7—figure supplement 2—source data 1
Excel table with data represented in this figure.
- https://cdn.elifesciences.org/articles/62183/elife-62183-fig7-figsupp2-data1-v2.xlsx
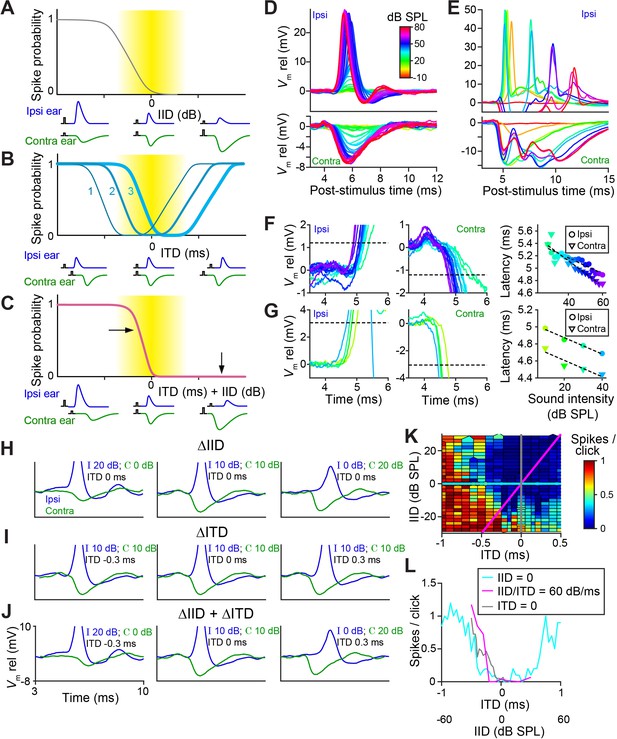
LSO neurons show graded latency-intensity changes which disambiguate spatial tuning.
(A) Cartoon showing change in spike probability for changing IID. Yellow area shows approximate region of physiological IID values. Traces below each plot represent the timing and amplitude of ipsi- and contralateral synaptic events to click-pairs with different IID values. (B) Cartoon showing change in spike probability for changing ITD, for three cases with different centering of the trough. (C) Cartoon showing change in spike probability for combined changes in ITD and IID. Horizontal and vertical arrows indicate effects of adding IID to ITD: a rightward shift in the left slope, and inhibition of the right shoulder of the tuning function. (D) Average responses to ipsilateral clicks (top panel) and contralateral clicks (bottom panel) of different sound levels for a principal LSO cell (CF = 12 kHz; same cell as Figure 2A). (E) Similar to D, for a non-principal (marginal) LSO cell (CF = 4.1 kHz; same cell as Figure 2B). Colors as in D. (F) Ipsilateral (left panel) and contralateral responses (middle panel) for the same principal LSO cell as in D. Colors correspond to sound levels, as in D. Dashed lines indicate the threshold used to calculate latencies, a voltage difference relative to rest with an absolute value of 20% of the average IPSP amplitude (where IPSP amplitude was measured at the lowest sound level that led to the maximal spike rate when presented to the ipsilateral ear). For the ipsilateral ear, this voltage difference was depolarizing, for the contralateral ear, it was hyperpolarizing. Right panel: latency values as a function of sound level, corresponding to the data in the left and middle panel. (G) Similar to F, for the same non-principal LSO cell as in E. (H) Averaged monaural responses to click pairs with different IIDs, for a principal cell (CF = 3.5 kHz). (I) Similar to H, but now ITD varies and IID is kept constant at 0 dB. (J) Similar to H and I, but for combined changes in ITD and IID. (K) Voronoi diagrams of spike rate for different ITD and IID combinations, for the same principal cell as in H-J. Colors are clipped between the limits shown in the color scale. Gray and cyan lines connect data points of respectively IID and ITD functions. Diagonal magenta line connects data points for which there is a consistent change in ITD and IID (60 dB change in IID per 1 ms change in ITD, which is realistic for this CF [Maki and Furukawa, 2005]). Data was pooled across different sound levels. (L) Gray, cyan, and magenta functions show spike rates along the lines of the same color in K. Data from the same principal LSO cell as in H-K. Numerical data represented as graphs in this figure are available in a source data file (Figure 8—source data 1).
-
Figure 8—source data 1
Excel table with data represented in this figure.
- https://cdn.elifesciences.org/articles/62183/elife-62183-fig8-data1-v2.xlsx
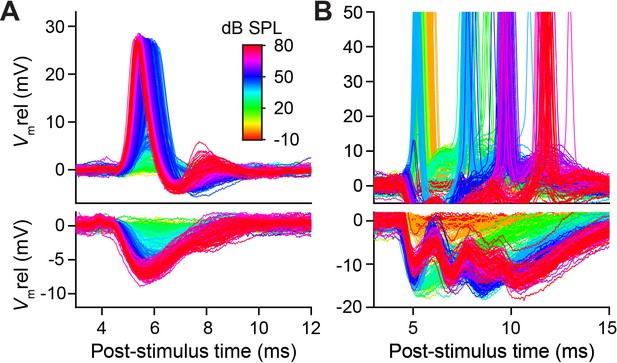
Individual traces corresponding to the mean data shown in Figure 8D and E.
(A) Same cell as Figure 8D. Top panel shows responses to ipsilateral clicks, bottom panel to contralateral clicks. Traces are color-coded according to SPL (same color legend in A and B). SPL is varied from 0 to 80 dB in steps of 2 dB. (B) Same cell as Figure 8E. SPL is varied from −10 to 80 dB in steps of 10 dB. Note that ordinate is adjusted in top panel to better show the subthreshold Vm, resulting in clipping of the spikes. Numerical data represented as graphs in this figure are available in a source data file (Figure 8—figure supplement 1—source data 1).
-
Figure 8—figure supplement 1—source data 1
Excel table with data represented in this figure.
- https://cdn.elifesciences.org/articles/62183/elife-62183-fig8-figsupp1-data1-v2.xlsx
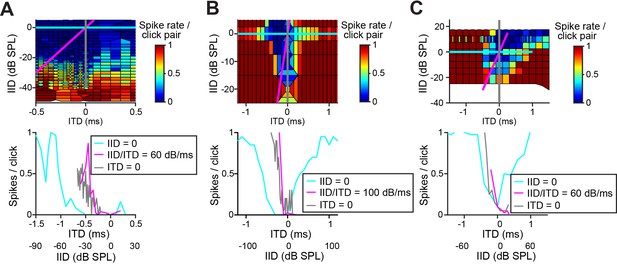
Similar as Figure 8K and L, for three additional LSO neurons.
(A–C) Similar response maps to combined ITD and IID variations as Figure 8K and L, for (A) a non-principal (type 5) LSO cell (CF = 2.0 kHz), (B) another principal LSO cell (CF = 12 kHz) and (C) a juxtacellularly recorded LSO cell (CF = 2.7 kHz). As in Figure 8K and L, top panels show Voronoi plots of average spike rate as a color code for different combinations of ITD and IID. In the bottom panel, an ITD function (cyan), IID function (gray) and a function where both cues are varied (magenta) are plotted. The cue combinations for these three functions are shown by straight lines in the same colors in the top panels. For the magenta function, the slope of IID/ITD was chosen according to CF, to reflect the known dependence of binaural cues on frequency (Maki and Furukawa, 2005). Numerical data represented as graphs in this figure are available in a source data file (Figure 8—figure supplement 2—source data 2).
-
Figure 8—figure supplement 2—source data 2
Excel table with data represented in this figure.
- https://cdn.elifesciences.org/articles/62183/elife-62183-fig8-figsupp2-data2-v2.xlsx
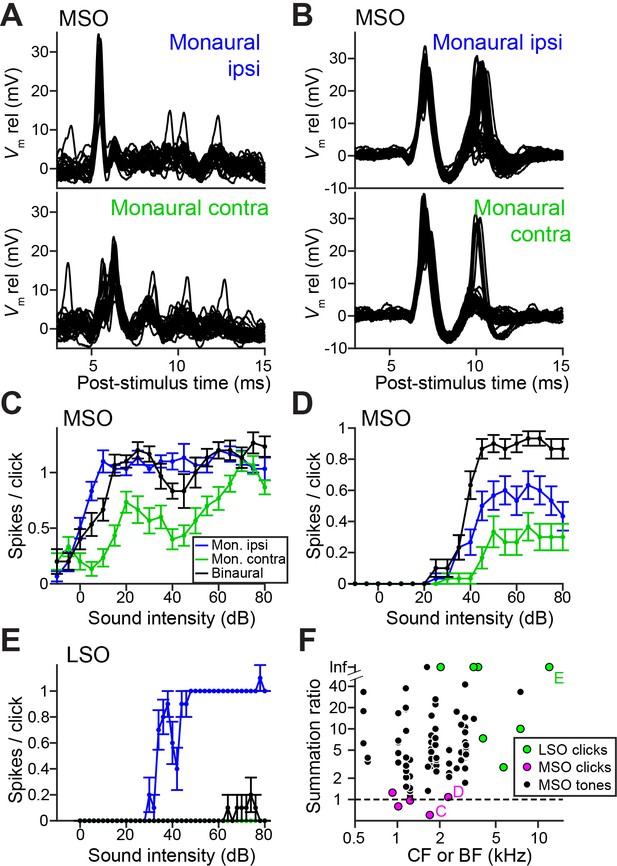
Weak ITD-tuning in MSO neurons results from a breakdown of coincidence detection for transients.
(A) Top panel: Example responses to ipsilateral clicks (top panel) and contralateral clicks (bottom panel) for an MSO cell (CF = 1.8 kHz). Sound level 70 dB SPL. 30 repetitions shown. (B) Similar to A, for another MSO cell (CF = 4.6 kHz). Sound level 70 dB SPL. 30 repetitions shown. (C) Rate-level functions (30 repetitions) for monaural clicks and for binaural clicks at 0 ITD for the same MSO cell as in A. Data are represented as mean ± SEM. (D) Similar to C, for another MSO cell (CF = 2.3 kHz). Thirty repetitions per SPL. (E) Similar to C and D, for a principal LSO cell (CF = 12 kHz). Thirty repetitions per SPL. (F) Summation ratio for LSO responses to clicks (seven data sets, seven cells), MSO responses to clicks (five data sets, five cells) and MSO responses to sustained tones (77 data sets, 22 cells). For a summation ratio of one (dashed horizontal line), the binaural response equals the sum of the monaural responses. Letters C,D,E indicate data points of the cells in the corresponding panels. For MSO responses to tones, one data point with a summation ratio of ~200 is not shown. Numerical data represented as graphs in this figure are available in a source data file (Figure 3—source data 1).
-
Figure 3—source data 1
Excel table with data represented in this figure.
- https://cdn.elifesciences.org/articles/62183/elife-62183-fig3-data1-v2.xlsx
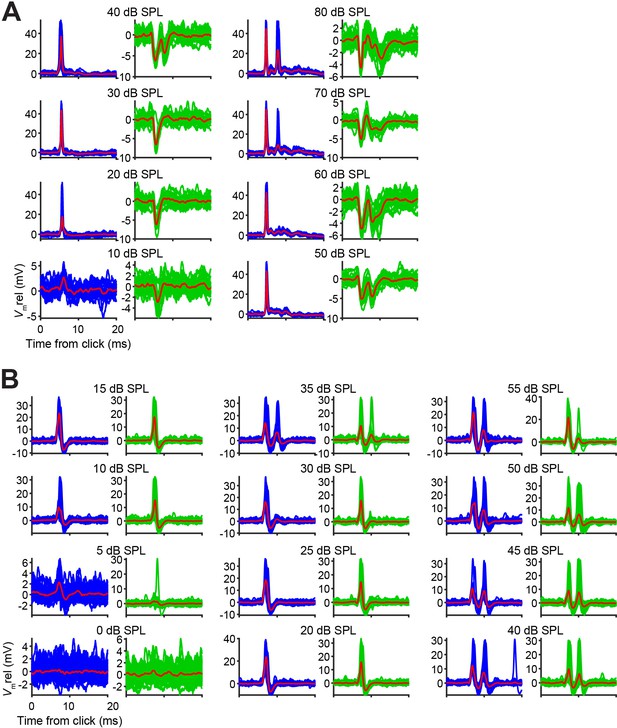
Monaural stimulation often leads to double events both in LSO and MSO.
In both LSO and MSO, double events in response to a single monaural click were sometimes observed, for both ipsi- and contralateral stimulation. Each pair of panels shows individual responses to monaural clicks at one level. Traces in response to ipsilateral clicks are shown in blue (left panels), and those in response to contralateral clicks are shown in green (right panels). The average response is overlaid in red in each panel. (A) Data from principal LSO cell (CF = 1149 Hz; 20 repetitions per level). Double EPSPs can be elicited by a single click, visible here for 70 and 80 dB SPL. Similarly, double IPSPs were often elicited by a single click, here at sound levels of 40 dB or higher. (B) Data from MSO cell (CF = 4.6 kHz; 50 repetitions per level). Again, often double EPSPs were elicited by single monaural ipsilateral or contralateral clicks, at sound levels of 35 dB SPL or higher. These findings suggest that double events are already present in the inputs to LSO and MSO neurons. Indeed, we have observed double firings to single clicks in the monaural input pathways to both circuits (auditory nerve, bushy cells, medial nucleus of the trapezoid body - data not shown). Numerical data represented as graphs in this figure are available in a source data file (Figure 3—figure supplement 1—source data 1).
-
Figure 3—figure supplement 1—source data 1
Excel table with data represented in this figure.
- https://cdn.elifesciences.org/articles/62183/elife-62183-fig3-figsupp1-data1-v2.xlsx
Tables
Reagent type (species) or resource | Designation | Source or reference | Identifiers | Additional information |
---|---|---|---|---|
Strain, strain background (Meriones unguiculatus, male and female) | Wildtype | In vivo: Janvier Labs In vitro: Animals raised in colony at at UT-Austin Animal Resource Center (breeders obtained from Charles River Laboratories) | ||
Antibody | Anti-Synaptophysin1 (Guinea pig polyclonal) | Synaptic Systems | Cat# 101–004; RRID:AB_1210382 | (1:500) |
Antibody | Anti-AnkyrinG (rabbit polyclonal) | Galiano et al., 2012 | (1:200) Courtesy of Dr. Matthew Rasband (Baylor College of Medicine) | |
Antibody | Anti-Gephyrin (mouse monoclonal) | Synaptic Systems | Cat# 147–011; RRID:AB_887717 | (1:200) |
Software, algorithm | MATLAB | The Mathworks | ||
Software, algorithm | IGOR-Pro | Wavemetrics | ||
Software, algorithm | MafDC | Yang et al., 2015 | Courtesy of Dr. Matthew Xu-Friedman | |
Software, algorithm | SIM-post processing software | Zeiss | ||
Software, algorithm | Metamorph | Molecular Devices |
Additional files
-
Source code 1
Code to generate figures of the computational model.
- https://cdn.elifesciences.org/articles/62183/elife-62183-code1-v2.zip
-
Transparent reporting form
- https://cdn.elifesciences.org/articles/62183/elife-62183-transrepform-v2.pdf