Preserving inhibition with a disinhibitory microcircuit in the retina
Figures
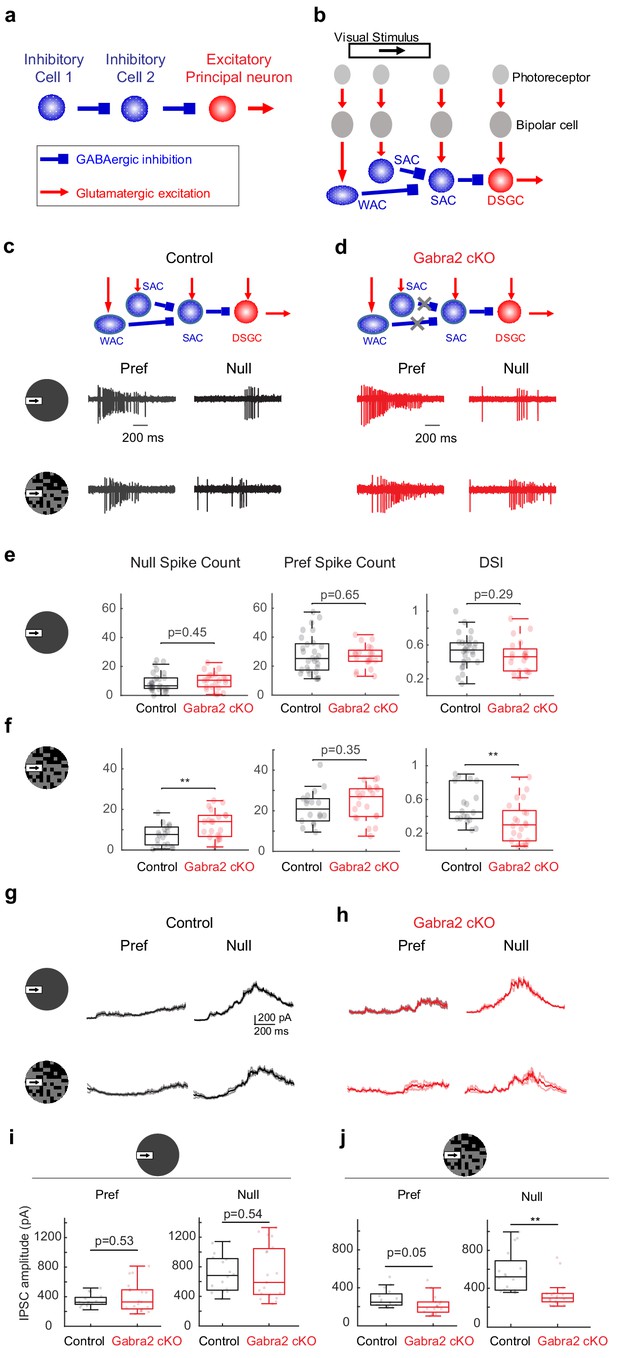
On-Off DSGCs in Gabra2 cKO mice show reduced direction selectivity and reduced null-direction inhibition in the noisy background.
(a) Organization of a canonical disinhibitory microcircuit. (b) Schematic of disinhibitory motifs WAC-SAC-DSGC and SAC-SAC-DSGC in the direction-selective circuit. WAC, SAC, and DSGC receive light-evoked glutamatergic inputs from the photoreceptor-bipolar cell signaling pathway. DSGC axons leave the retina and innervate higher brain nuclei. (c-f) DSGC spiking activity (c) Upper: schematic of disinhibitory motifs in control mice. Lower: example spiking activity of a DSGC in the control group during a moving bar in the preferred (pref) and null directions in noise-free (upper traces) and noisy (lower traces) backgrounds. (d) Same as c, from a DSGC in the Gabra2 cKO group. (e) Summary plots of null-direction (left), preferred-direction (middle) spike counts and direction selectivity index (DSI, see Materials and methods) in control and Gabra2 cKO groups for moving bar in the noise-free background. Box plots represent minimum / first quartile / median / third quartile / maximum values for this and subsequent figures, dots represent individual cells. Control: n = 19 cells from 6 mice. Gabra2 cKO: n = 20 cells from 6 mice. (f) Same as (e) for moving bar in the noisy background. (g-j) DSGC IPSC . (g) Example bar-evoked IPSC traces of a DSGC in the control group during a moving bar in the preferred and null directions in noise-free (upper traces) and noisy (lower traces) backgrounds. Three individual trials (thin lines) and the mean (thick line) are shown. (h) Same as g, from a DSGC in Gabra2 cKO group. (i) Summary plots of preferred- (left) and null-direction (right) IPSC peak amplitudes in control and Gabra2 cKO groups for moving bar in the noise-free background. Control: n = 17 cells from 6 mice. Gabra2 cKO: n = 20 cells from 7 mice. (j) Same as I, for moving bar in the noisy background. See also Figure 1—figure supplements 1–3.
-
Figure 1—source data 1
On-Off DSGCs inGabra2cKO mice show reduced direction selectivity and reduced null-direction inhibition in the noisy background.
- https://cdn.elifesciences.org/articles/62618/elife-62618-fig1-data1-v2.xlsx
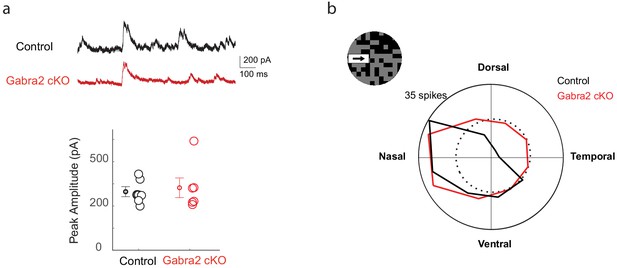
Flickering checkerboard-evoked DSGC response.
(a) Upper: Example DSGC IPSC traces of control and Gabra2 cKO mice during the flickering checkerboard stimulus before the moving bar appeared. Lower: Average peak amplitude of flicker-evoked IPSCs. Data represent mean ±- SEM. Control: 6 cells, two mice. Gabra2 cKO: 6 cells, two mice. (b) Example polar plots of DSGC spiking response duirng the noisy bar stimulus.
-
Figure 1—figure supplement 1—source data 1
Flickering checkerboard-evoked DSGC response.
- https://cdn.elifesciences.org/articles/62618/elife-62618-fig1-figsupp1-data1-v2.xlsx
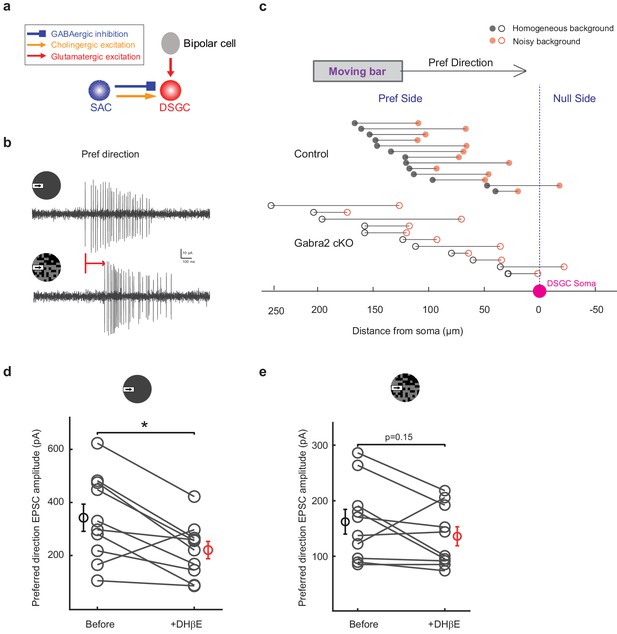
Excitatory inputs onto DSGCs in noisy background are primarily glutamatergic.
(a) Schematic showing sources of synaptic inputs to DSGCs. Excitatory inputs to DSGCs include glutamatergic inputs from bipolar cells and cholinergic inputs from SACs. Cholinergic excitation of DSGCs from laterally connected SACs during the moving bar stimulus arrives earlier than glutamatergic excitation from vertically connected bipolar cells. (b) Example loose-attach recording of a control DSGC evoked by the leading edge of a moving bar in noise-free (upper) and noisy (lower) backgrounds. Red arrow indicates the delay of spiking onset in noisy background. (c) Spatial locations of the leading edge of the moving bar relative to the soma (pink dot at zero on the X-axis) at the onset of bar-evoked DSGC spiking response. For each DSGC, the bar locations for noise-free (black) and noisy (red) backgrounds are plotted at the same Y axis position and connected with a solid black line. Control group cells are represented by filled circles and Gabra2 cKO cells are represented by empty circles. The onset time of DSGC spiking activity was determined by a binless algorithm adapted from Chase and Young, 2007. Mean distance from soma: control noise-free background:127.6 ± 11.5 μm, control noisy background 73.0 ± 12.8 μm, n = 13 cells, Gabra2 cKO noise-free background: background 126.8 ± 21.5 μm, Gabra2 cKO noisy background 74.0 ± 17.7 μm, n = 11 cells. (d) Pair-wise comparison of preferred-direction EPSC amplitude evoked by the moving bar in noise-free background before and after application of 0.08 μM cholinergic receptor antagonist DhβE in control mice. n = 10 cells from five mice. (e) Same as d, during the moving bar stimulus in the noisy background.
-
Figure 1—figure supplement 2—source data 1
Excitatory inputs onto DSGCs in noisy background are primarily glutamatergic.
- https://cdn.elifesciences.org/articles/62618/elife-62618-fig1-figsupp2-data1-v2.xlsx
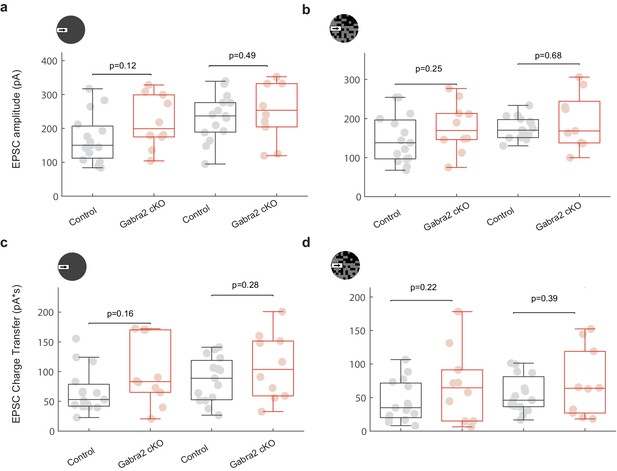
On-Off DSGC EPSCs are not affected in Gabra2 KO during the moving bar stimulus in the noisy background.
(a) EPSC peak amplitudes in response to the moving bar in noise-free background in control (black) and Gabra2 cKO (red) mice. Left: null direction; Right: preferred direction. Control: 16 cells from six mice. Gabra2 cKO: 10 cells from eight mice. (b) Same as a, in the noisy background. (c) Same as a, for excitatory charge transfer (integral of EPSC over time). (d) Same as c, in the noisy background.
-
Figure 1—figure supplement 3—source data 1
On-Off DSGC EPSCs are not affected inGabra2KO during the moving bar stimulus in the noisy background.
- https://cdn.elifesciences.org/articles/62618/elife-62618-fig1-figsupp3-data1-v2.xlsx
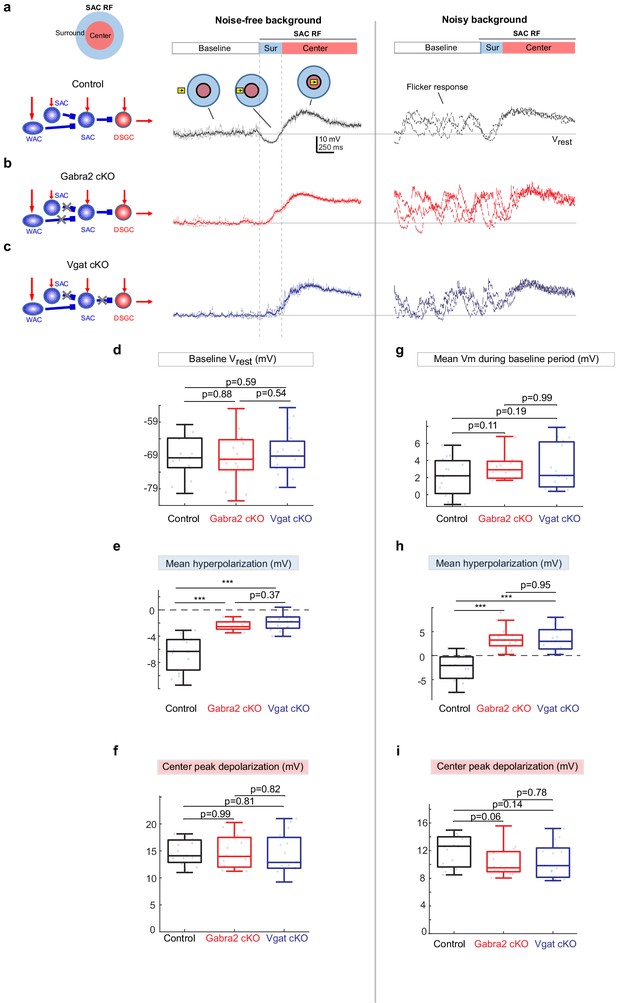
Weakened motion-evoked surround suppression of SACs in Gabra2 cKO and Vgat cKO mice.
(a) Example Vm traces from a control SAC during moving bar in noise-free (left) and noisy background (right). For noise-free condition, lighter traces are three individual sweeps and darker trace represents the mean. For noisy condition, three individual sweeps are shown. For each sweep, the flickering checkerboard pattern was randomly generated and differed from those of other sweeps. Horizontal line indicates the resting membrane potential Vrest calculated as the mean baseline Vm under the noise-free condition. Vertical dashed lines mark the surround suppression time window. Insets: Upper left: schematic of the center-surround RF structure of a SAC. Upper right: the time windows during which the bar moved outside the RF (‘Baseline’), in the RF surround (Sur) and center (‘Center’). (b) Same as a, from a SAC in the Gabra2 cKO group. (c) Same as a, from a SAC in the Vgat cKO group. (d-f): summary plots for noisy-free background.d.Mean Vm during the Baseline time window indicated in a. In noisy-free background it is equivalent to the resting membrane potential Vrest.e.Mean hyperpolarization calculated as the mean Vm during the Surround time window relative to Vrest.f.Peak depolarization calculated as the peak Vm during the Center time window relative to Vrest. (g-i): summary plots for noisy backgroundg.Mean Vm during the Baseline window relative to Vrest.h.Mean Vm during the Surround window relative to Vrest.i.Peak Vm during the Center time window relative to Vrest.Control, n = 15 cells from four mice. Gabra2 cKO, n = 15 cells from four mice. Vgat cKO, n = 15 cells from four mice. See also Figure 2—figure supplement 1.
-
Figure 2—source data 1
Weakened motion-evoked surround suppression of SACs inGabra2cKO and Vgat cKO mice.
- https://cdn.elifesciences.org/articles/62618/elife-62618-fig2-data1-v2.xlsx
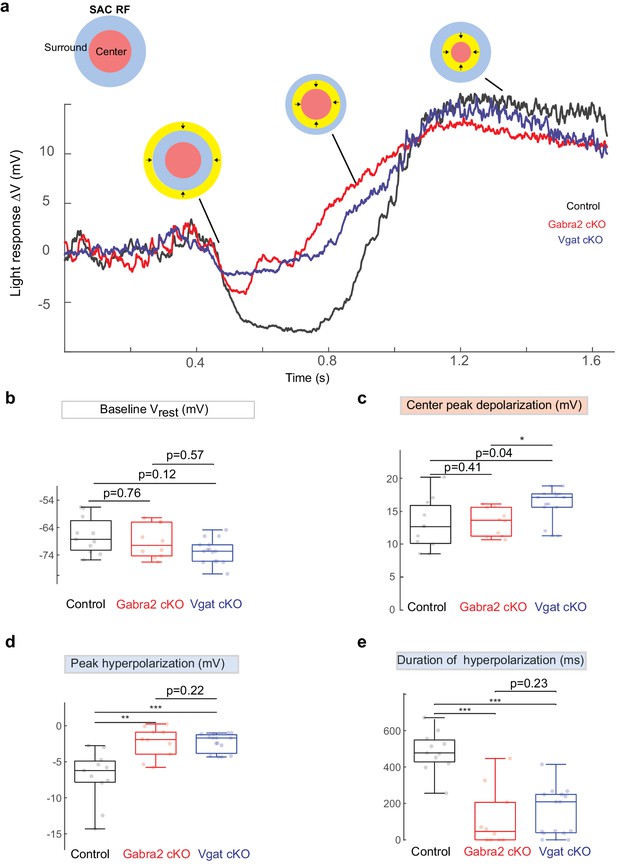
Weakened motion-evoked surround suppression of SACs in Gabra2 cKO and Vgat cKO mice during contracting ring stimulus.
(a) Example Vm traces (baseline adjusted) from three SACs (Control, black; Gabra2 cKO, red; Vgat cKO, blue) during contracting ring stimuli. The ring was centered on the soma and moved inward at a speed of 265 µm/s. Resting membrane potential Vrest calculated as the mean baseline Vm of a 500 ms time window before visual stimuli was presented to the retina. Insets: Upper left: schematic of the center-surround RF structure of an SAC. (b) Mean Vm during the Baseline time window. Box plots represent minimum/first quartile/median/ third quartile/maximum values for this and subsequent figures, grey dots represent individual cells. (c) Peak depolarization calculated as the peak Vm relative to Vrest. (d) Peak hyperpolarization calculated as the valley of Vm during relative to Vrest when contracting rings stimulates the surround of the SAC. (e) Duration of hyperpolarization phase when contracting ring activates the surround of SAC. The duration of hyperpolarization is defined as the total period of Vm hyperpolarized by more than 1 mV when contracting ring moves in the surruond of the SAC. Control, n = 11 cells from five mice. Gabra2 cKO, n = 10 cells from four mice. Vgat cKO, n = 15 cells from three mice.
-
Figure 2—figure supplement 1—source data 1
Flickering checkerboard-evoked DSGC response.
- https://cdn.elifesciences.org/articles/62618/elife-62618-fig2-figsupp1-data1-v2.xlsx
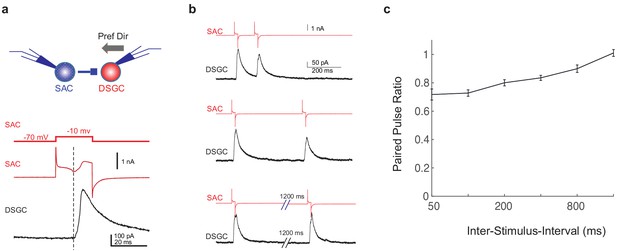
GABAergic SAC-DSGC synapses in adult mice exhibit paired pulse depression.
(a) Upper: schematic of paired voltage clamp recordings between a DSGC and a SAC on the null side of the DSGC. Preferred direction of the DSGC is shown as the black arrow. Lower: command potential of the SAC (upper trace), holding current of the SAC (middle trace), and DSGC IPSC evoked by SAC depolarization (lower trace). Dashed line indicates the onset of the IPSC, which coincides with the inward calcium current activated in the SAC (Koren et al., 2017). (b) Example traces of SAC and DSGC recordings at different inter-pulse intervals (200, 400, 1600 ms). (c) Summary plot of paired pulse ratio (2nd/1st peak amplitudes) as a function of inter-pulse interval. X-axis is in log scale. N = 17 pairs from three mice.
-
Figure 3—source data 1
GABAergic SAC-DSGC synapses in adult mice exhibit paired pulse depression.
- https://cdn.elifesciences.org/articles/62618/elife-62618-fig3-data1-v2.xlsx
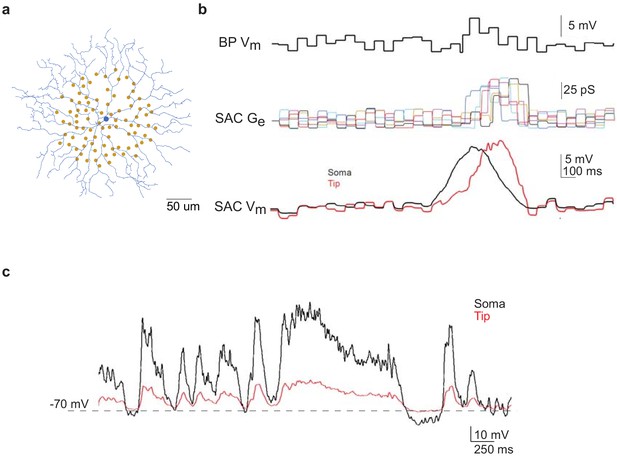
Computational modeling of SAC light responses at soma and dendritic tips during the moving bar stimulus in the noisy background.
(a) A digitized SAC (blue) and locations of bipolar cell somas (yellow dots) used for simulation. (b) Upper trace: simulated Vm of a bipolar cell during moving bar stimulus in noisy background. Middle traces: simulated excitatory conductance (Ge) of the SAC evoked by six example bipolar cell inputs. Colored traces are SAC Ge evoked by individual bipolar cells. Lower traces: simulated SAC somatic Vm at the soma and at the distal tip of a dendrite. (c) Simulation of soma-to-dendritic tip attenuation in a SAC during voltage clamp recording. Black trace: scaled somatic Vm waveform (black trace) of a SAC based on the current clamp recording of a SAC Vm during the moving bar stimulus in noisy background. Red trace: simulated Vm at the SAC’s distal dendritic tip in the voltage-clamp configuration.
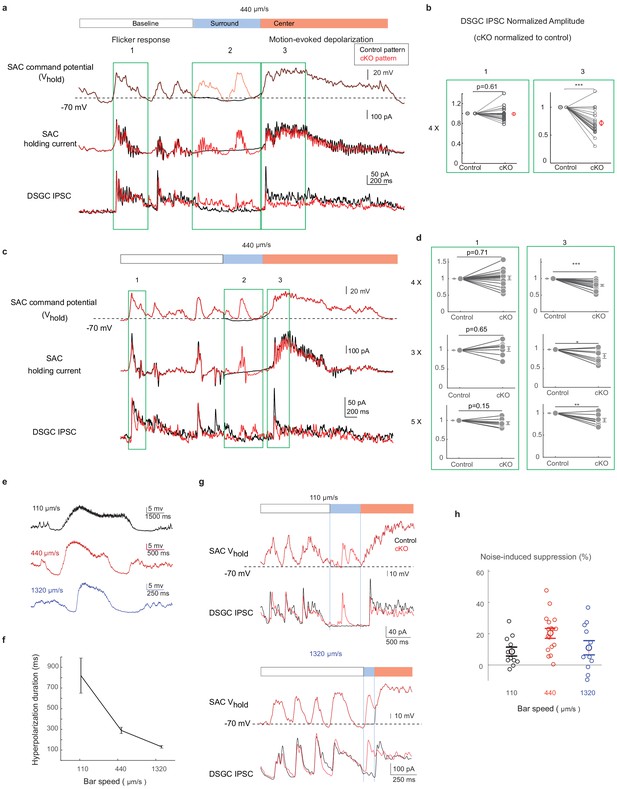
The activation pattern of Gabra2 cKO SACs during motion in the noisy background induces synaptic depression between SACs and DSGCs in control mice.
(a) Top traces: the scaled control (black) and Gabra2 cKO (red) waveforms during noisy bar stimulus used to voltage-clamp SAC somatic Vm in control mice. Middle traces: holding currents of a SAC during the paired SAC-DSGC voltage-clamp recordings. Downward deflections represent SAC calcium currents activated by membrane depolarization. Lower traces: DSGC IPSCs evoked by the control and the cKO waveforms. Schematic on top of the traces indicates time windows corresponding to the baseline period, bar-evoked surround suppression and bar-evoked depolarization during current clamp experiments in Figure 2. Green boxes numbered 1, 2, and 3 are regions in baseline, surround and center time windows chosen for subsequent quantification. (b) Pair-wise comparisons of DSGC IPSC amplitude evoked by the scaled (4x) control and cKO waveforms in time windows 1 and 3. For each cell pair, the IPSC peak amplitude evoked by the cKO waveform is normalized to that of the control waveform for each time window. N = 17 pairs from seven mice. (c) Same as a, with a different representative cKO waveform. (d) As b, but with different scale factors for the SAC command waveforms in c. (e) Example control SAC Vm traces during moving bar stimuli over gray background at various speeds. (f) Duration of the hyperpolarization window during noise-free moving bar as a function of bar speed. 110 μm/s: 11 cells, 4 mice, 440 μm/s: 15 cells, 4 mice, 1320 μm/s: 21 cells, 6 mice. Data are represented as mean ± SEM. (g) Same as a, but with scaled cKO SAC Vm waveforms at 110 μm/s (upper) and 1320 μm/s (lower). For control waveforms, mean membrane potential of the control waveforms replaces the cKO traces in the surround suppression time window. (h) Summary of synaptic suppression of the SAC-DSGC synapse induced by the Gabra2 cKO waveform relative to the response evoked by the control waveform for various motion speeds. Noise-induced expression is calculated as the percentage decrease of motion-evoked cKO response relative to the control. 110 µm/s: 11 pairs from three mice; 440 µm/s: 17 pairs from seven mice; 1320 µm/s: 11 pairs from three mice. Data are represented as mean ± SEM. See also Figure 5—figure supplement 1.
-
Figure 5—source data 1
The activation pattern ofGabra2cKO SACs during motion in the noisy background induces synaptic depression between SACs and DSGCs in control mice.
- https://cdn.elifesciences.org/articles/62618/elife-62618-fig5-data1-v2.xlsx
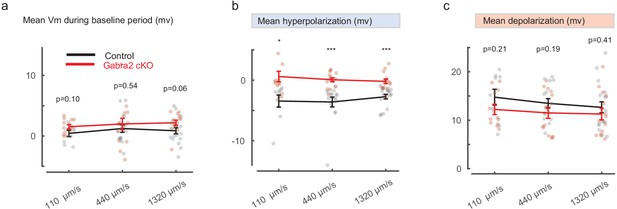
Gabra2 cKO SACs show enhanced noise responses prior to the motion-evoked responses at different speeds.
(a) Mean Vm during the Baseline window relative to Vrest. (b) Mean Vm during the Surround window relative to Vrest. (c) Peak Vm during the Center time window relative to Vrest. 110 μm/s: Control 9 cells, cKO 12 cells; 440 μm/s: Control 15 cells, cKO 15 cells; 1320 μm/s: Control 18 cells, cKO 13 cells.
-
Figure 5—figure supplement 1—source data 1
Gabra2cKO SACs show enhanced noise responses prior to the motion-evoked responses at different speeds.
- https://cdn.elifesciences.org/articles/62618/elife-62618-fig5-figsupp1-data1-v2.xlsx
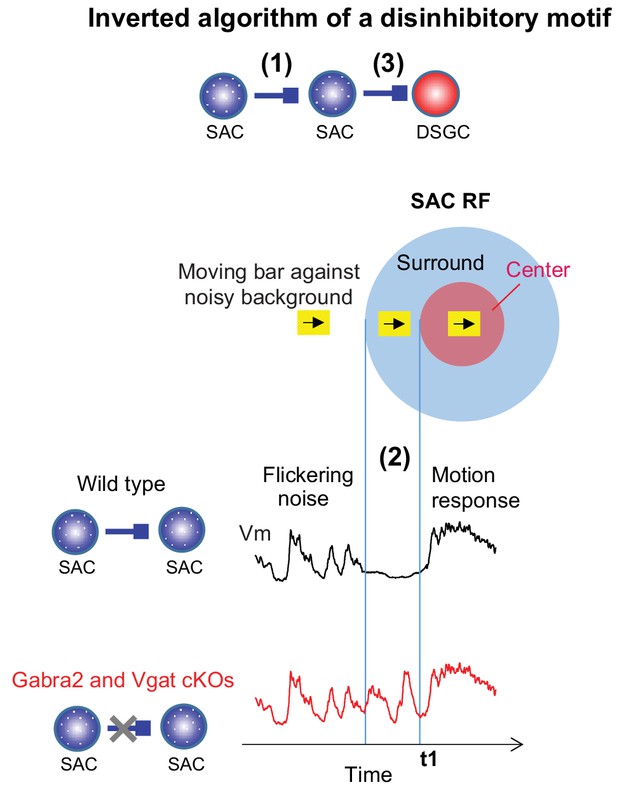
Mechanisms underlying the preservation of motion-evoked DSGC inhibition by the SAC-SAC-DSGC disinhibitory motif.
The inverted algorithm of the SAC-SAC-DSGC disinhibitory motif arises from three mechanistic components during motion processing in the noisy background. The SAC-SAC inhibition contributes to a strong RF surround of SACs (1), which enables motion-evoked suppression of SAC Vm that dampens SAC flicker response (2). Dampened SAC flicker response before its motion-evoked response prevents short-term synaptic depression of the SAC-DSGC synapse during motion stimuli (3) and preserves the strength of null-direction inhibition of the DSGC (see more in Discussion).
Tables
Reagent type (species) or resource | Designation | Source or reference | Identifiers | Additional information |
---|---|---|---|---|
Strain, strain background (M. musculus) | wild-type (C57BL/6J) mice | The Jackson Laboratory | RRID:IMSR_JAX:000664 | |
Strain, strain background (M. musculus) | Chat-IRES-Cre (129S6-Chattm2(cre)Lowl/J) mice | The Jackson Laboratory | RRID:IMSR_JAX:006410 | |
Strain, strain background (M. musculus) | floxed tdTomato (129S6-Gt(ROSA)26Sortm9(CAG-tdTomato)Hze/J) mice | The Jackson Laboratory | RRID:IMSR_JAX:007905 | |
Strain, strain background (M. musculus) | Drd4-GFP (SW-Tg(Drd4-EGFP)W18Gsat/Mmnc) mice | MMRRC | RRID:MMRRC_000231-UNC | |
Strain, strain background (M. musculus) | Gabra2 flox/flox mice | Gift from Dr. Uwe Rudolph, Auferkorte et al., 2012 | ||
Strain, strain background (M. musculus) | Slc32a1t flox/flox(Slc32a1tm1Lowl/J) mice | The Jackson Laboratory | RRID:IMSR_JAX:012897 | |
Chemical compound, drug | D-AP5 | Tocris | Cat#0106; CAS: 79055-68-8 | |
Chemical compound, drug | DNQX disodium salt | Tocris | Cat#2312; CAS: 1312992-24-7 | |
Chemical compound, drug | Dihydro-β-erythroidine hydrobromide (DhβE) | Tocris | Cat#2349; CAS: 29734-68-7 | |
Chemical compound, drug | L-AP4 | Tocris | Cat#0103; CAS: 23052-81-5 | |
Software, algorithm | NeuronC Neural Simulation Language | Data subfolder nc/models/sbac_noise | manual: http://retina.anatomy.upenn.edu/~rob/neuronc.html source code:ftp://retina.anatomy.upenn.edu/pub/nc.tgz | |
Software, algorithm | PCLAMP 10 | Molecular Devices | https://www.moleculardevices.com/systems/conventional-patch-clamp/pclamp-10-software; RRID:BDSC_14352 | |
Software, algorithm | Prairie View | Bruker Technologies | https://www.bruker.com/products/fluorescence-microscopes/ultima-multiphoton-microscopy/ultima-in-vitro/overview.html | |
Software, algorithm | MATLAB | MathWorks | http://www.mathworks.com; RRID:SCR_01622 | |
Other | Custom MATLAB scripts for visual stimulation and data analysis | This paper | https://github.com/chrischen2/eLife2020Stimulus.git; Chen, 2020; copy archived at swh:1:rev:dd7cc7b01d0fd41d62335ceef0f72a5e921cf374 |