Optogenetic strategies for high-efficiency all-optical interrogation using blue-light-sensitive opsins
Figures
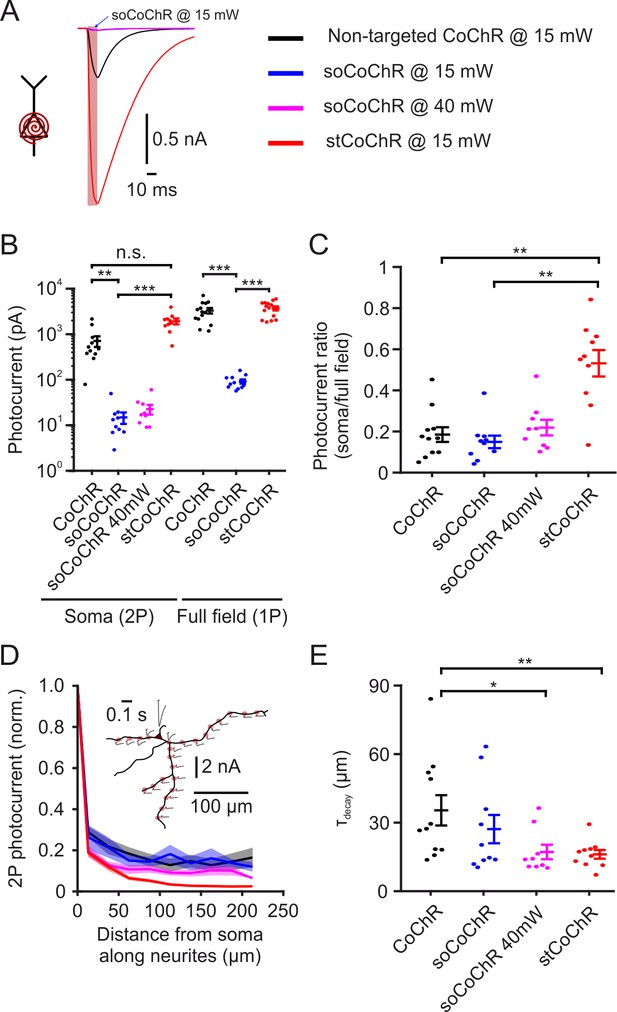
Characterization of soma-targeted CoChR variants.
(A) Example photocurrents recorded in the acute slice preparation from cortical neurons expressing either non-targeted CoChR, stCoChR or soCoChR upon two-photon scanning with a spiral pattern (10 µm diameter) over the soma (λ = 940 nm, 15 or 40 mW on cell, 10.05 ms scan duration, 80 MHz laser repetition rate). Red shaded background indicates scan period. Traces are from cells displaying the median photocurrent per construct. (B) Photocurrents for each CoChR variant under two-photon spiral scan over the soma (as in A) and under one-photon full-field illumination (470 nm, 0.27 mW/mm2, 500 ms). Please note the logarithmic photocurrent scale. Points are individual cells and vertical lines are mean ± SEM. In this as well as in other figures, *p<0.05; **p<0.01; ***p<0.001; n.s., non-significant. (C) Ratio between the photocurrent under soma spiral stimulation and full-field stimulation per cell, based on the data in B. (D) Photocurrents obtained during two-photon spiral stimulation of selected points along the neurites of cells in the acute slice preparation expressing either variant, normalized to the current obtained at the soma. Distances are measured from the soma along the path of the neurite to the stimulation point and are binned with 25 µm intervals. Shaded background represents SEM. Inset shows a morphological reconstruction of an example cell (black) expressing stCoChR with the stimulation points (shaded red circles) and the absolute photocurrent obtained at each point (gr with red tick indicating stimulation period). (E) Decay length constants of the photocurrent with distance from soma, based on individual cell data from D. p values in B,C,E are based on Kruskal-Wallis test with post hoc comparisons using Tukey’s post hoc HSD test.
-
Figure 1—source data 1
Source data for Figure 1 and Figure 1—figure supplement 2.
- https://cdn.elifesciences.org/articles/63359/elife-63359-fig1-data1-v2.xlsx
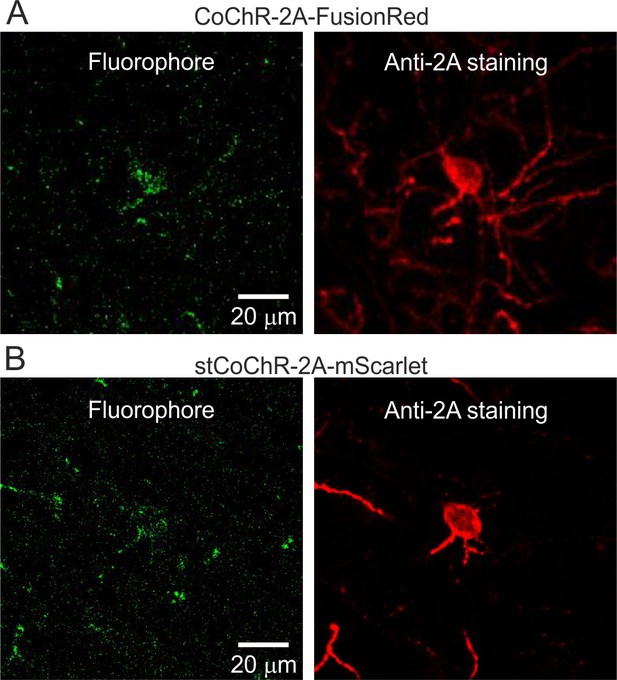
Expression of CoChR and stCoChR.
(A–B) Confocal images (z-stack maximum projections) of cells expressing either non-targeted CoChR (A) or stCoChR (B). The signal of the fluorophore co-expressed with CoChR (FusionRed) and with stCoChR (mScarlet) is shown in the left image. The staining for the 2A peptide that is conjugated to CoChR (A) and to stCoChR (B) is shown in the right image.
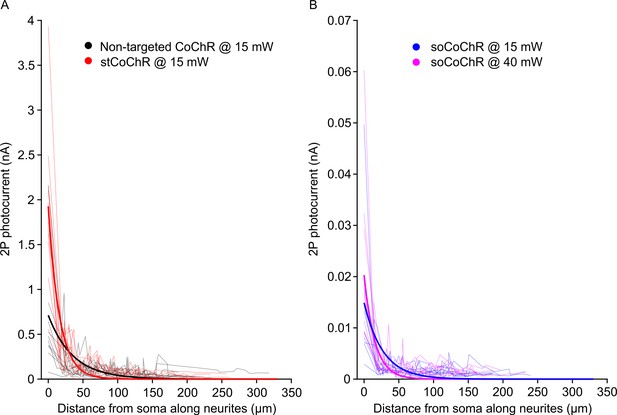
Two-photon photocurrents in neurons expressing different CoChR variants obtained during spiral-scan stimulation of selected points along the neurites.
Related to Figure 1. (A) Photocurrent amplitudes recorded in the acute slice preparation from neurons expressing the non-targeted CoChR (black) and stCoChR (red). Thin lines indicate individual cell data, thick lines indicate average monoexponential fits across recorded cells. (B) Photocurrents recorded in the acute slice preparation from neurons expressing soCoChR at two different average stimulation powers. Thin lines indicate individual cell data, thick lines indicate average monoexponential fits across recorded cells. The same cells expressing soCoChR were used for both stimulation powers.
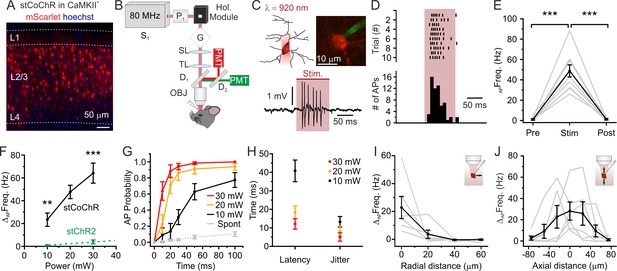
High-efficiency two-photon holographic stimulation of neurons expressing the large-conductance opsin stCoChR in vivo.
(A) Confocal image showing cortical principal cells expressing stCoChR (CoChR-Kv2.1-P2A-mScarlet). (B) Schematic of the optical set up. (C) A holographic oval shape projected on the target neuron (top left), which is recorded in the juxtasomal electrophysiological configuration (top right). Representative trace from one stimulated neuron (bottom panel). The pink bar indicates the stimulation period (stimulation power: 20 mW; illumination duration: 100 ms). (D) Raster plot of holographic stimulation-induced firing activity across consecutive sweeps (top graph) in the cell shown in (C). The corresponding AP time histogram is shown in the bottom panel. (E) Frequency of APs before (Pre), during (Stim), and after (Post) the holographic two-photon stimulation. Individual experiments are shown in gr, the average across experiments in black (stimulation power: 20 mW). One-way repeated measure ANOVA with post hoc Bonferroni correction, p=1.3E-5, n = 10 cells from four mice. (F) Change in AP frequency as a function of the average stimulation power. Black indicates results for cells expressing stCoChR (n = 10 neurons from four animals). Green indicates results for cells expressing stChR2 (n = 8 neurons from four animals). For 10 mW average power, unpaired Student’s t-test, p=3.5E-3; for 30 mW average power, Mann-Whitney test, p=4.6E-5, λstim = 920 nm. Data in green are taken from Forli et al., 2018. (G) Probability of discharging one or more AP as a function of time during holographic stimulation, n = 10 cells from four animals. Red, yellow, and black indicate different average stimulation powers. Gr indicates the spontaneous AP probability in the absence of holographic stimulation. (H) Latency to first AP and jitter of first AP in holographic stimulation experiments on cells expressing stCoChR, n = 10 cells from four mice. Average stimulation power is color coded. (I) Firing frequency increase vs displacement of the excitation volume in the radial direction during holographic illumination of L2/3 cells expressing stCoChR. (J) Firing frequency increase vs displacement of the excitation volume in the axial direction during holographic illumination of L2/3 cell expressing stCoChR. For (I and J), n = 6 cells from five mice. Stimulation power: 15 mW.
-
Figure 2—source data 1
Source data for Figure 2.
- https://cdn.elifesciences.org/articles/63359/elife-63359-fig2-data1-v2.xlsx
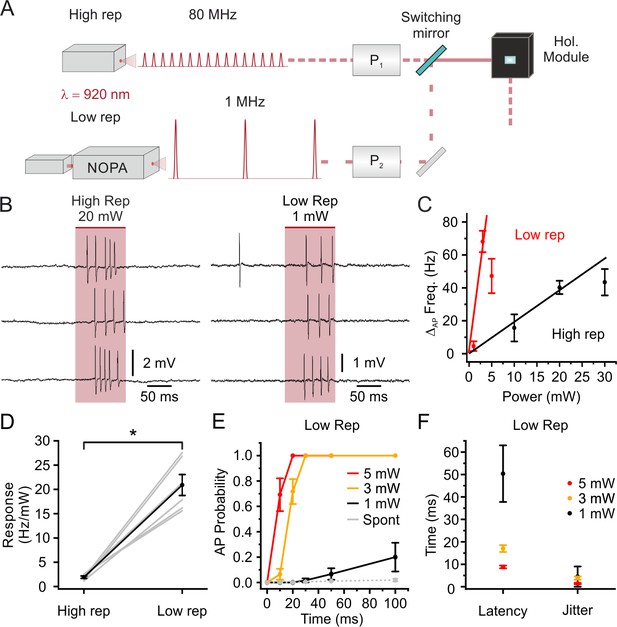
Stimulation with low repetition rate laser sources decreases the average power delivered to the sample while maintaining high stimulation efficiency: principal neurons.
(A) Schematic of the experimental configuration: one high (80 MHz) and one low (1 MHz) repetition rate laser sources are alternated to stimulate the same electrophysiologically recorded cell. Both laser sources are tuned at 920 nm. P1, Pockels cell 1; P2, Pockels cell 2; NOPA, Non- collinear Optical Parametric Amplifier. (B) Representative traces from the same recorded L2/3 pyramidal neuron in vivo showing the effect of holographic stimulation using the high (left, average power: 20 mW) and the low (right, average power: 1 mW) repetition rate laser. (C) Change in AP frequency as a function of average stimulation power. Black indicates results using the high repetition rate laser and red indicates results using the low repetition rate laser. The red and black lines are fitting the values obtained with non-saturating stimulation power (1 and 3 mW for low repetition, 10 and 20 mW for high repetition, respectively). In this as well in the other panels of this figure, n = 6 cells from two mice. (D) Neural response in terms of AP frequency increase per mW of delivered average laser power in the case of stimulation with the high repetition rate laser (High rep) and low repetition rate laser (Low rep). Wilcoxon signed rank test, p=0.031. (E) AP probability as a function of duration in holographic stimulation experiments using low repetition rate on L2/3 pyramidal neurons expressing stCoChR. Red, yellow, and black indicate different average stimulation powers. Grey indicates the spontaneous AP probability in the absence of holographic stimulation. (F) Latency to first AP and jitter of first AP in holographic stimulation experiments using low repetition rate on L2/3 pyramidal neurons expressing stCoChR. Average stimulation power used in the experiments is indicated with the color code.
-
Figure 3—source data 1
Source data for Figure 3.
- https://cdn.elifesciences.org/articles/63359/elife-63359-fig3-data1-v2.xlsx
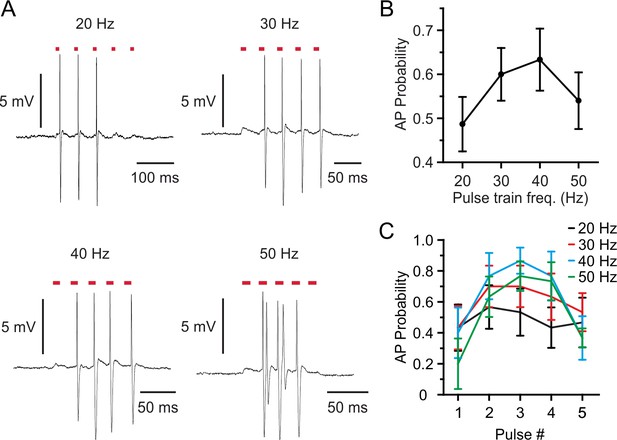
Spike control in stCoChR-expressing neurons at different stimulation frequencies.
(A) Representative traces recorded from the same L2/3 pyramidal neuron during two-photon holographic stimulation with a 5-pulses train at different frequency (pulse duration, 10 ms; average power, 5 mW; stimulation frequency, 20–50 Hz). (B) Probability of discharging at least one AP per pulse as a function of the frequency of the stimulation train. n = 6 neurons from three mice. (C) Probability of eliciting APs for each pulse of the stimulation train. n = 6 neurons from three mice.
-
Figure 3—figure supplement 1—source data 1
Source data_for Figure 3—figure supplement 1.
- https://cdn.elifesciences.org/articles/63359/elife-63359-fig3-figsupp1-data1-v2.xlsx
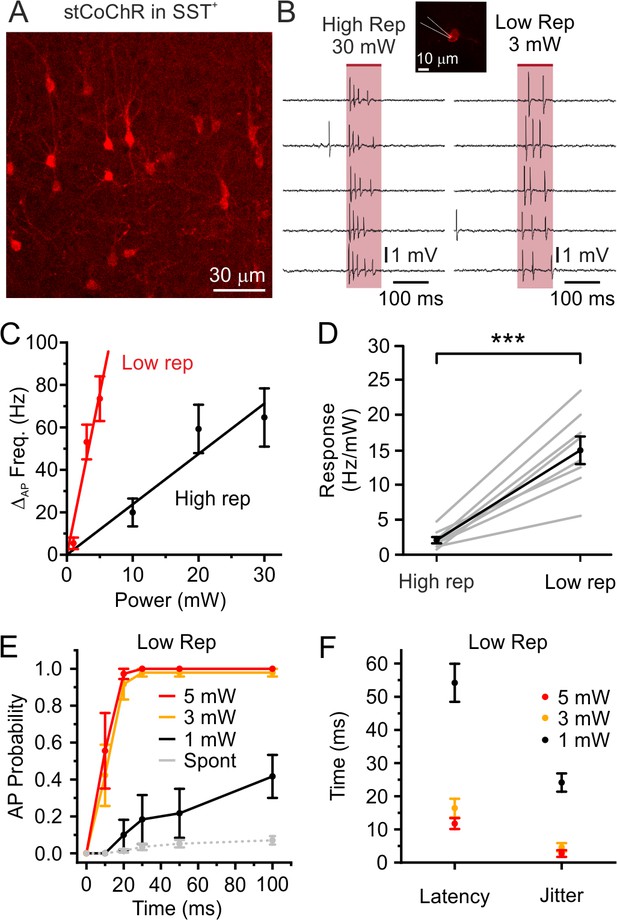
Stimulation with low repetition rate laser sources decreases the average power delivered to the sample while maintaining high stimulation efficiency: somatostatin (SST)-positive interneurons.
Related to Figure 3. (A) Confocal image showing expression of stCoChR (CoChR-Kv2.1-P2A-mScarlet) in SST-positive (SST+) interneurons. (B) Representative traces from the same electrophysiologically recorded interneuron in vivo showing the effect of holographic stimulation using the high (left, 80 MHz) and the low (right, 1 MHz) repetition rate laser. (C) Change in AP frequency as a function of average stimulation power. Black indicates results using the high repetition rate laser (High rep) and red indicates results using the low repetition rate laser (Low rep); n = 8 cells from four animals. (D) Neural response in terms of AP frequency increase per mW of delivered average laser power in the case of stimulation with the high repetition rate laser (High rep) and low repetition rate laser (Low rep). n = 8 cells from four mice; paired Student’s t-test, p=2E-4. (E) AP probability as a function of time in holographic stimulation in SST-positive interneurons. Red, yellow, and black indicate different average stimulation powers. Gr indicates the spontaneous AP probability in the absence of holographic stimulation. n = 6 cells from two mice. (F) Latency (left) to first AP and jitter (right) of first AP in holographic stimulation experiments on SST-positive interneurons expressing stCoChR. Average stimulation power: 5 mW. n = 10 cells from four mice.
-
Figure 3—figure supplement 2—source data 1
Source data_for Figure 3—figure supplement 2.
- https://cdn.elifesciences.org/articles/63359/elife-63359-fig3-figsupp2-data1-v2.xlsx
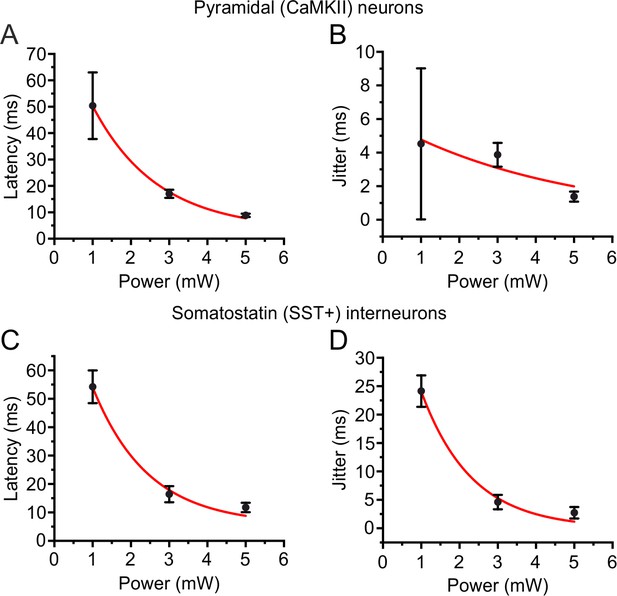
Latency and jitter decrease with the average power of low repetition rate stimulation.
Related to Figure 3. (A) Latency to first AP as a function of the average power of the holographic stimulation with low repetition rate lasers in L2/3 pyramidal neurons. (B) Jitter of first AP as a function of the average power of the holographic stimulation with low repetition rate lasers in L2/3 pyramidal neurons. (C) Same as in (A) but for L2/3 somatostatin-positive interneurons. (D) Same as in (B) but for L2/3 somatostatin-positive interneurons. In A-B, n = 6 cells from two mice; in C-D, n = 10 cells from four mice.
-
Figure 3—figure supplement 3—source data 1
Source data_for Figure 3—figure supplement 1.
- https://cdn.elifesciences.org/articles/63359/elife-63359-fig3-figsupp3-data1-v2.xlsx
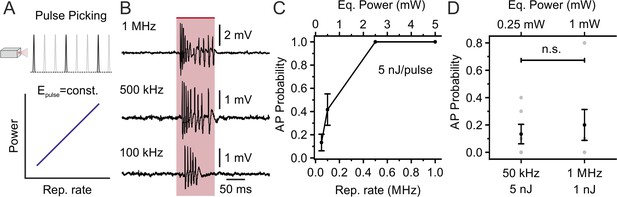
Decreasing the laser repetition rate to <1 MHz allows holographic stimulation of stCoChR-expressing cells with <1 mW average power per cell: principal neurons.
(A) The laser repetition rate is decreased using pulse picking (top panel). With this approach, the average power delivered to the sample linearly increases with the repetition rate (bottom panel). (B) Representative traces from the same electrophysiologically recorded L2/3 pyramidal neuron stimulated at different repetition rates (from top to bottom panel: 1 MHz, 500 kHz, 100 kHz; energy per pulse: 5 nJ). (C) Probability of discharging one or more AP as a function of the laser repetition rate for principal neurons expressing stCoChR in vivo (illumination duration: 100 ms). n = 6 cells from two mice. (D) AP probability obtained at 50 kHz and 1 MHz repetition rates, but delivering lower average power (0.25 mW vs 1 mW) and higher energy per pulse (5 nJ vs 1 nJ) at 50 kHz compared to 1 MHz. n = 6 cells from two mice; Wilcoxon signed rank test p=0.88.
-
Figure 4—source data 1
Source data for Figure 4.
- https://cdn.elifesciences.org/articles/63359/elife-63359-fig4-data1-v2.xlsx
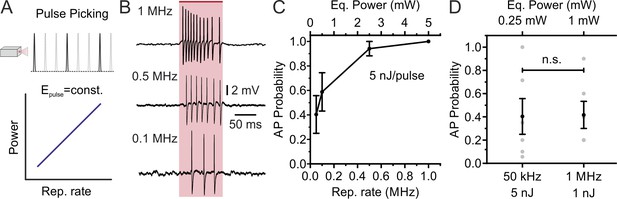
Decreasing the laser repetition rate to <1 MHz allows holographic stimulation of stCoChR-expressing cells with <1 mW average power per cell: SST-positive interneurons.
Related to Figure 4. (A) The laser repetition rate is decreased using pulse picking (top panel). With this approach, the average power delivered to the sample linearly increases with the repetition rate (bottom panel). (B) Representative traces from the same electrophysiologically recorded SST-positive interneuron stimulated at different repetition rates (from top to bottom panel: 1 MHz, 500 kHz, 100 kHz; energy/pulse: 5 nJ). (C) Probability of discharging one or more AP as a function of the laser repetition rate for SST-positive cells expressing stCoChR in vivo (energy per pulse: 5nJ); n = 6 cells from three mice. (D) AP probability obtained at 50 kHz and 1 MHz repetition rate, but delivering lower average power (0.25 mW vs 1 mW) and higher energy per pulse (5 nJ vs 1 nJ) at 50 kHz compared to 1 MHz. n = 6 cells from three mice; paired Student’s t-test, p=0.84.
-
Figure 4—figure supplement 1—source data 1
Source data for Figure 4—figure supplement 1.
- https://cdn.elifesciences.org/articles/63359/elife-63359-fig4-figsupp1-data1-v2.xlsx
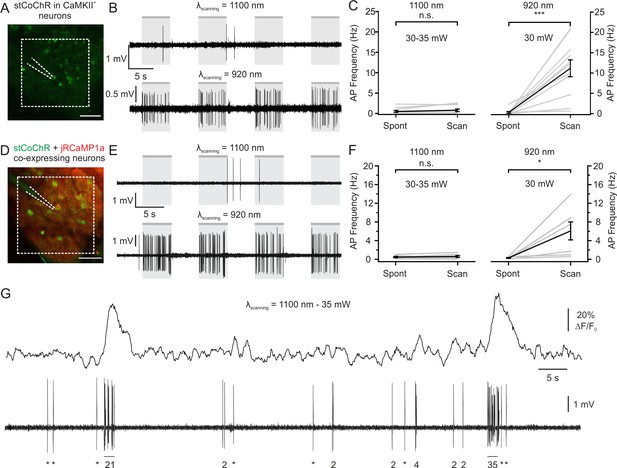
Limited crosstalk between imaging and photostimulation using blue-light-sensitive opsins and red-shifted indicators.
(A) Two-photon image of L2/3 principal cells expressing stCoChR in vivo (CoChR-Kv2.1-P2A-NLS-eGFP). One opsin-positive neuron was recorded with a glass pipette (dashed lines) in the juxtasomal configuration while two-photon raster scanning inside the indicated area (dashed white box, 161.4 × 161.4 μm2) was performed (imaging frame rate: 11 Hz). (B) Traces recorded in the juxtasomal electrophysiological configuration from one stCoChR-expressing neuron during epochs (gr boxes) of two-photon raster scanning at wavelength 1100 nm (top) and 920 nm (bottom). Laser power: 30 mW. (C) Average AP frequency during epochs of spontaneous activity (Spont) and during raster scanning (Scan). The left panel shows results when scanning was performed at λ = 1100 nm. The right panel displays the results when scanning was done at λ = 920 nm. n = 11 cells from six mice; Wilcoxon signed rank test, p=0.31 for λ = 1100 nm; Wilcoxon signed rank test, p=9.8E-4 for λ = 920 nm. (D) Same as (A), but for cells co-expressing stCoChR (CoChR-Kv2.1-P2A-NLS-eGFP) and jRCaMP1a. (E) Same as (B), but from a cell co-expressing stCoChR and jRCaMP1a. (F) Same as (C), but for neurons co-expressing stCoChR and jRCaMP1a. n = 7 cells from three mice; Wilcoxon signed rank test, p=0.21 for λ = 1100 nm; Wilcoxon signed rank test, p=0.02 for λ = 920 nm. (G) Representative trace from a L2/3 pyramidal neuron co-expressing stCoChR and jRCaMP1a. AP firing was recorded in the juxtasomal configuration (bottom) while two-photon raster scanning was performed (top). Imaging frame rate and FOV dimensions as in A; average imaging power, 35 mW. The number of recorded APs is reported below the electrophysiological trace (single APs are indicated with an asterisk).
-
Figure 5—source data 1
Source data for Figure 5.
- https://cdn.elifesciences.org/articles/63359/elife-63359-fig5-data1-v2.xlsx
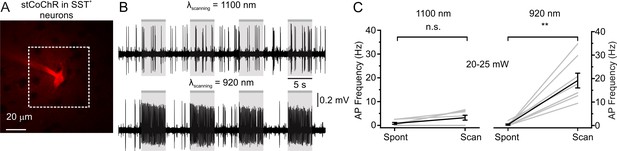
Crosstalk between imaging and photostimulation: SST-positive interneurons Related to Figure 5.
(A) Two-photon image of one recorded L2/3 SST-positive cell expressing stCoChR in vivo. (B) Traces recorded in the juxtasomal configuration from one stCoChR-expressing interneuron during spontaneous activity (white background) and during epochs of two-photon raster scanning (gr background) at wavelength 1100 nm (top) and wavelength 920 nm (bottom). Laser power: 20 mW. (C) Average AP frequency during epochs of spontaneous activity (Spont) and during raster scanning (Scan). The left panel shows results when scanning was performed at λ = 1100 nm. Wilcoxon paired rank test, p=0.06, n = 8 cells from four mice. The right panel displays the results when scanning was done at λ = 920 nm. Wilcoxon paired rank test, p=7.8E-3, n = 8 cells from four mice.
-
Figure 5—figure supplement 1—source data 1
Source data for Figure 5—figure supplement 1.
- https://cdn.elifesciences.org/articles/63359/elife-63359-fig5-figsupp1-data1-v2.xlsx
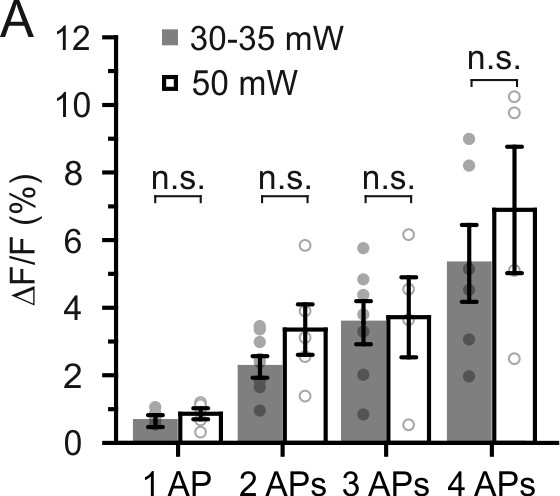
ΔF/F0 of spontaneous jRCaMP1a events did not significantly increase with imaging average power.
(A) ΔF/F0 of spontaneous jRCaMP1a transients associated with the discharge of 1, 2, 3, and 4 APs. AP count was obtained from juxtasomal electrophysiological recordings simultaneously performed with two-photon imaging in jRCaMP1a-expressing neurons (imaging frame rate, 11 Hz; n = 4–8 from two mice). 1 AP, Mann-Whitney test, p=0.38; 2 APs, unpaired Student’s t-test, p=0.19; 3 APs, unpaired Student’s t-test, p=0.91; 4 APs, unpaired Student’s t-test, p=0.46. Measurements from individual cells are represented as dots; bars indicate the average across cells.
-
Figure 5—figure supplement 2—source data 1
Source data for Figure 5—figure supplement 2.
- https://cdn.elifesciences.org/articles/63359/elife-63359-fig5-figsupp2-data1-v2.xlsx
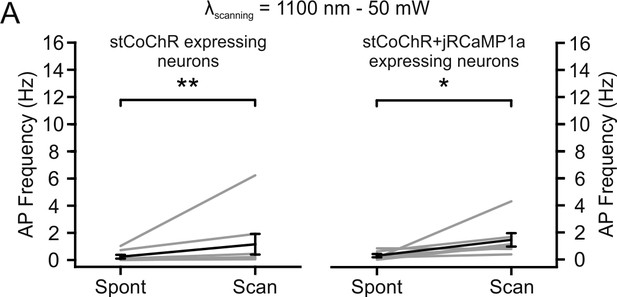
Crosstalk between imaging and photostimulation at 50 mW imaging average power.
(A) Average firing frequency of L2/3 pyramidal neurons expressing stCoChR (left panel) or co-expressing stCoChR and jRCaMP1a (right panel) during epochs of spontaneous activity (Spont) and during two-photon raster scanning (Scan, imaging frame rate, 11 Hz) at 1100 nm and 50 mW average imaging power. Left panel: n = 8 from four mice; Wilcoxon signed ranks test, p=7.8E-3. Right panel: n = 7 from three mice; Wilcoxon signed ranks test, p=0.03.
-
Figure 5—figure supplement 3—source data 1
Source data for Figure 5—figure supplement 3.
- https://cdn.elifesciences.org/articles/63359/elife-63359-fig5-figsupp3-data1-v2.xlsx
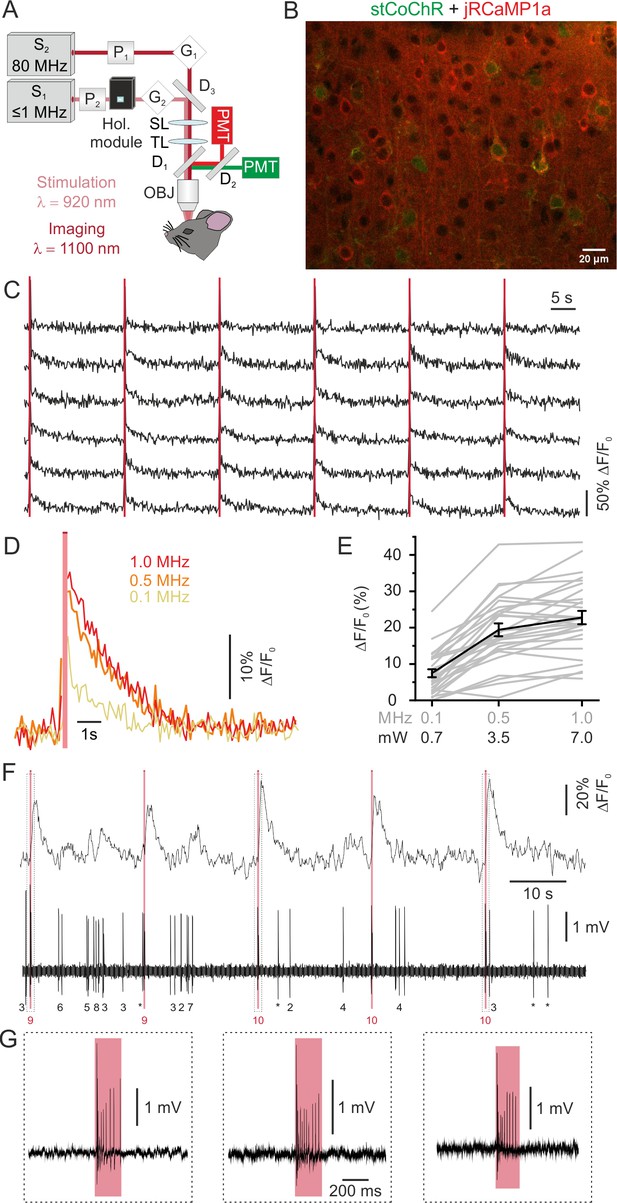
All-optical imaging and high-efficiency manipulation combining jRCaMP1a and stCoChR.
(A) Schematic of the optical system. Two laser sources tuned at different wavelengths are combined in the same set up for simultaneous imaging and optogenetic manipulation. (B) Confocal image showing cortical L2/3 neurons expressing jRCaMP1a (red) and stCoChR (CoChR-Kv2.1-P2A-NLS-eGFP, green). (C) Representative traces showing the response of an ensemble of jRCaMP1a- and stCoChR-expressing neurons to successive holographic stimulations (pink bars) in vivo. Periods of stimulation are blanked. Traces from six stimulated neurons are shown in the panel. Average imaging power range, 30–35 mW; frame rate, 11 Hz. (D) Representative traces showing average jRCaMP1a signals in response to holographic stimulation at different laser repetition rates (from 100 kHz to 1 MHz; average stimulation energy per cell: 7 nJ per pulse, 200 ms illumination; n = 28 cells from two mice, six consecutive stimulations per cell). (E) ΔF/F0 of jRCaMP1a signals as a function of the repetition rate (or the equivalent average power per cell) of the stimulating laser, n = 28 cells from two mice. Mean values (of six consecutive stimulations) from individual cells are shown in grey, the average across experiments in black. (F) Representative trace showing jRCaMP1a fluorescence (top) and the corresponding juxtasomal electrophysiological recording from one cell co-expressing jRCaMP1a and stCoChR. The pink bar indicates the stimulation period (average stimulation power, 5 mW; repetition rate, 1 MHz; stimulus duration, 200 ms). The number of recorded APs is reported below the electrophysiological trace (single APs are indicated with an asterisk; the number of AP during holographic stimulation is shown in red). (G) The juxtasomal electrophysiological trace during holographic stimulation is shown at an expanded time scale and shows stimulation-induced AP trains.
-
Figure 6—source data 1
Source data for Figure 6.
- https://cdn.elifesciences.org/articles/63359/elife-63359-fig6-data1-v2.xlsx
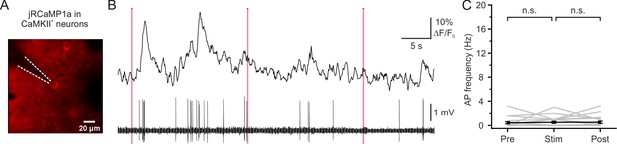
Photostimulation of neurons expressing only jRCaMP1a did not increase the neuron’s firing rate.
(A) Two-photon image of L2/3 pyramidal neurons expressing jRCaMP1a in vivo. The neuron was recorded with a glass pipette (dashed lines), while simultaneously performing two-photon imaging (λ = 1100 nm) and holographic stimulation (λ = 920 nm). (B) Representative fluorescence trace (top panel) and spiking activity (bottom panel) from a target neuron. Pink bars represent holographic stimulation periods (average stimulation power, 7 mW; stimulation duration, 200 ms; laser repetition rate, 1 MHz). Imaging frame rate, 11 Hz. The number of recorded APs is reported below the electrophysiological trace; single APs are indicated with an asterisk. (C) Average firing frequency before (Pre), during (Stim), and after (Post) stimulation. One-way repeated measure ANOVA with post hoc Bonferroni correction, p=0.70, n = 21 cells from four mice.
-
Figure 6—figure supplement 1—source data 1
Source data for Figure 6—figure supplement 1.
- https://cdn.elifesciences.org/articles/63359/elife-63359-fig6-figsupp1-data1-v2.xlsx
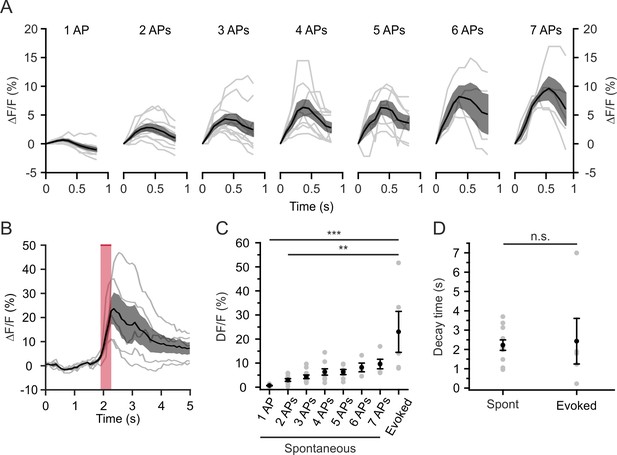
Spontaneous and stimulation-evoked jRCaMP1a transients.
(A) ΔF/F0 of jRCaMP1a transients associated with spontaneous firing of 1–7 APs. Data obtained from simultaneous two-photon imaging and juxtasomal recordings from L2/3 neurons expressing jRCaMP1a or jRCaMP1a and stCoChR. t = 0 represents the time of the first AP in the burst. Single cells are represented as gr lines, the average across cells as black line. The shaded area represents standard error of the mean (SEM) interval. n = 11 from four mice (imaging power, 30–35 mW; imaging frame rate, 11 Hz). (B) ΔF/F0 of jRCaMP1a transients evoked by holographic stimulation of L2/3 neurons. Pink bar represents holographic illumination (average stimulation power, 7 mW; imaging power, 30–35 mW; imaging frame rate, 11 Hz,). Single cells are shown in grey, the average across cells in black. The shaded area represents SEM interval. n = 5 from three mice. (C) ΔF/F0 of spontaneous and evoked jRCaMP1a transients. Single cells are represented as gr dots, average across cells in black. n = 4–11 from four mice for spontaneous events; n = 5 from three mice for evoked events. Kruskal-Wallis p<1E-4, Dunn's multiple comparisons test. Evoked vs 1 APs, p<1E-4. Evoked vs 2 APs, p=5E-3. (D) Decay time for spontaneous and evoked jRCaMP1a events. n = 11 from four mice for spontaneous events; n = 5 from three mice for evoked events, unpaired Student’s t-test, p=0.82.
-
Figure 6—figure supplement 2—source data 1
Source data for Figure 6—figure supplement 2.
- https://cdn.elifesciences.org/articles/63359/elife-63359-fig6-figsupp2-data1-v2.xlsx
Tables
Reagent type (species) or resource | Designation | Source or reference | Identifiers | Additional information |
---|---|---|---|---|
Strain, strain background (M. musculus) | C57BL/6J | Charles River | IMSR Cat# JAX:000664, RRID:IMSR_JAX:000664 | |
Strain, strain background (M. musculus) | STOCK Ssttm2.1(cre)Zjh/J | The Jackson Laboratory | IMSR Cat# JAX:013044, RRID:IMSR_JAX:013044 | |
Recombinant DNA reagent | pAAV-Syn-CoChR-GFP | Addgene | Addgene plasmid #59070; http://n2t.net/addgene:59070; RRID:Addgene_59070 | |
Recombinant DNA reagent | pAAV-EF1α-DIO-CoChR-T2A-FusionRed-WPRE | This paper | See Materials and methods, Section ‘Cloning of stCoChR and preparation of recombinant AAV vectors’ | |
Recombinant DNA reagent | pAAV-EF1α-DIO-CoChR-Kv2.1-P2A-mScarlet-WPRE | This paper | See Materials and methods, Section ‘Cloning of stCoChR and preparation of recombinant AAV vectors’ | |
Recombinant DNA reagent | pAAV-EF1α-DIO-CoChR-Kv2.1-P2A-NLS-eGFP-WPRE | This paper | See Materials and methods, Section ‘Cloning of stCoChR and preparation of recombinant AAV vectors’ | |
Recombinant DNA reagent | pAAV-hSynapsin-FLEX-soCoChR-GFP | Addgene | Addgene viral prep # 107712-AAV9; http://n2t.net/addgene:107712; RRID:Addgene_107712; | |
Recombinant DNA reagent | AAV1-CaMKII0.4-Cre-SV40 | Addgene | Addgene viral prep # 105558-AAV1; http://n2t.net/addgene:105558; RRID:Addgene_105558 | |
Recombinant DNA reagent | AAV1-hsyn-flex-NES-jRCaMP1a | Addgene | Addgene viral prep # 100853-AAV1; http://n2t.net/addgene:100853; RRID:Addgene_100853 | |
Recombinant DNA reagent | AAV1-CAG-flex-NES-jRCaMP1a | Addgene | Addgene viral prep # 100846; http://n2t.net/addgene:100846; RRID:Addgene_100846 | |
Recombinant DNA reagent | pGP-CMV-NES-jRCaMP1a | Addgene | Addgene plasmid # 61562; http://n2t.net/addgene:61562; RRID:Addgene_61562 | |
Recombinant DNA reagent | AAV1-CamKII-jRCaMP1a | This paper | See Materials and methods, Section ‘Cloning of stCoChR and preparation of recombinant AAV vectors’ | |
Software, algorithm | MATLAB | Mathworks | RRID:SCR_001622; https://it.mathworks.com/products/matlab.html | |
Software, algorithm | pClamp 10 | Molecular Devices | RRID:SCR_011323; https://www.moleculardevices.com/products/axon-patch-clamp-system/acquisition-and-analysis-software/pclamp-software-suite | |
Software, algorithm | OriginPro2018 | OriginLab | RRID:SCR_014212; https://www.originlab.com/ | |
Software, algorithm | GraphPad PRISM | GraphPad PRISM | RRID:SCR_002798; https://www.graphpad.com/ | |
Software, algorithm | ImageJ/Fiji | Fiji | RRID:SCR_002285; http://fiji.sc/ | |
Antibody | polyclonal rabbit anti-2A peptide primary antibody (Rabbit polyclonal) | EMD Millipore | Millipore Cat# ABS31, RRID:AB_11214282catalog # ABS31 | Diluted (1:500); production discontinued |
Antibody | polyclonal Cy5-conjugated donkey anti-rabbit secondary antibody (Donkey polyclonal) | Jackson ImmunoResearch | Jackson ImmunoResearch Labs Cat# 711-175-152, RRID:AB_2340607catalog # 711-175-152 | Diluted (1:500) |
Additional files
-
Source code 1
Source code for the analysis of spontaneous jRCaMP1a transients.
- https://cdn.elifesciences.org/articles/63359/elife-63359-code1-v2.zip
-
Source code 2
Source code for the analysis of all-optical recordings.
- https://cdn.elifesciences.org/articles/63359/elife-63359-code2-v2.zip
-
Source code 3
Source code for the extraction of fluorescence traces.
- https://cdn.elifesciences.org/articles/63359/elife-63359-code3-v2.zip
-
Source code 4
Source code for the extraction of fluorescence traces.
- https://cdn.elifesciences.org/articles/63359/elife-63359-code4-v2.zip
-
Supplementary file 1
Supplementary tables comparing photocurrent properties of CoChR, soCoChR, and stCoChR.
Supplementary Table 1.Photocurrents elicited by two-photon spiral scan over the soma for CoChR variants. ‘v’ marks under each post hoc p value denote which CoChR variants were compared post hoc to obtain each specific p value. This also applies to Supplementary Table 2–4. # indicates that a separate Kruskal-Wallis test with separate post hoc comparisons was performed for comparing soCoChR under 40 mW with stCoChR and CoChR, since the two light powers used for soCoChR are paired. The row of soCoChR @ 40 mW presents the results of this separate set of tests, in which soCoChR @ 40 mW replaces soCoChR in the post hoc comparisons. This applies also to Supplementary Table 3–4. Supplementary Table 2. Photocurrents elicited by one-photon full-field illumination of CoChR variants. Supplementary Table 3. Soma-to-full-field photocurrent ratio of CoChR variants. Supplementary Table 4. τdecay of photocurrent with distance from soma along neurites for CoChR variants.
- https://cdn.elifesciences.org/articles/63359/elife-63359-supp1-v2.docx
-
Transparent reporting form
- https://cdn.elifesciences.org/articles/63359/elife-63359-transrepform-v2.docx