Quantitative glycoproteomics reveals cellular substrate selectivity of the ER protein quality control sensors UGGT1 and UGGT2
Figures
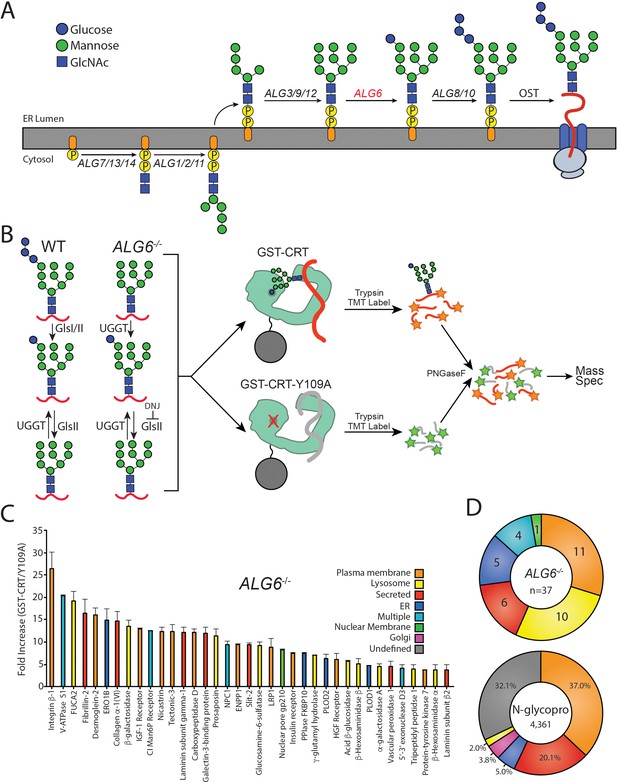
The identification of UDP-glucose:glycoprotein glucosyltransferase (UGGT) 1/2 substrates.
(A) The pathway of N-glycosylation in eukaryotic cells is depicted. N-glycan synthesis is initiated in the outer endoplasmic reticulum (ER) membrane leaflet on a dolichol-P-phosphate facing the cytoplasm. Flipping of the precursor N-glycan to the ER luminal leaflet and further synthesis steps mediated by ALG proteins leads to eventual transfer of a Glc3Man9GlcNAc2 N-glycan to a substrate by the oligosaccharyl transferase complex. ALG6 (red lettering) catalyzes the transfer of the initial glucose onto the Man9 precursor N-glycan. (B) In wild-type (WT) cells, a Glc3Man9GlcNAc2 N-glycan is transferred to substrates. Monoglucosylated substrates may therefore occur via trimming by glucosidases I/II (GlsI/II) or reglucosylation by UGGT1/2. In ALG6-/- cells, a Man9GlcNAc2 N-glycan is transferred to substrates. Therefore, monoglucosylated substrates may only occur through reglucosylation by UGGT1/2. Deoxynojirimycin (500 μM) was added to block the trimming of monoglucosylated substrates by GlsII. ALG6-/- cells were then lysed and split equally between affinity purifications with either GST-CRT or GST-CRT-Y109A bound to glutathione beads. Affinity-purified samples were then reduced, alkylated, trypsinized, and labeled with tandem mass tag (TMT) labels. Samples were then deglycosylated with PNGaseF, pooled, and analyzed by mass spectrometry. (C) Substrates were identified by dividing the quantification of the TMT label in the GST-CRT condition for each protein by that of the associated GST-CRT-Y109A condition, yielding the fold increase. Localization as predicted by UniprotKB annotation is depicted. A cutoff of threefold increase was applied. Data is representative of two independent experiments. Error bars represent standard error of the mean (SEM). (D) The N-glycoproteome (N-glycopro) was computationally determined by collecting all proteins annotated to contain N-glycans by UniprotKB. Annotated localization information was then used to computationally determine the localization distribution of the N-glycoproteome as well as the identified UGGT substrates.
-
Figure 1—source data 1
TMT quantification results for Figure 1C.
- https://cdn.elifesciences.org/articles/63997/elife-63997-fig1-data1-v2.xlsx
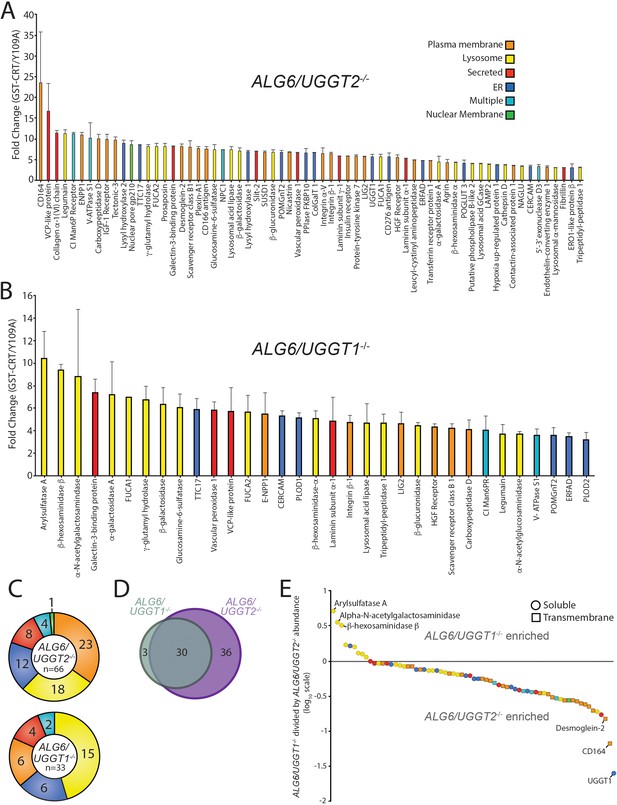
Identification of UDP-glucose:glycoprotein glucosyltransferase (UGGT)1- and UGGT2-specific substrates.
(A) Reglucosylation substrates in ALG6/UGGT2-/- cells were identified and quantified as previously described in Figure 1. Localizations as annotated by UniprotKB are depicted. Data are representative of two independent experiments. Error bars represent SEM. (B) Reglucosylated substrates in ALG6/UGGT1-/- cells were identified and quantified as previously above. (C) The distribution of localizations as annotated by UniprotKB for reglucosylation substrates identified in both ALG6/UGGT2-/- and ALG6/UGGT1-/- cells is depicted. (D) The overlap of reglucosylated substrates identified in both ALG6/UGGT2-/- cells (purple) and ALG6/UGGT1-/- cells (gray) is visualized by a Venn diagram. (E) Reglucosylated substrate enrichment in either ALG6/UGGT1-/- or ALG6/UGGT2-/- cells is depicted by dividing the tandem mass tag quantification for each protein in ALG6/UGGT1-/- cells by the associated value in ALG6/UGGT2-/- cells on a log10 scale. Positive and negative values represent enrichment in ALG6/UGGT1-/- and ALG6/UGGT2-/- cells, respectively. Localization (coloring) and topology (soluble [circles] or transmembrane [squares]) are depicted based on UniprotKB annotation.
-
Figure 2—source data 1
- https://cdn.elifesciences.org/articles/63997/elife-63997-fig2-data1-v2.xlsx
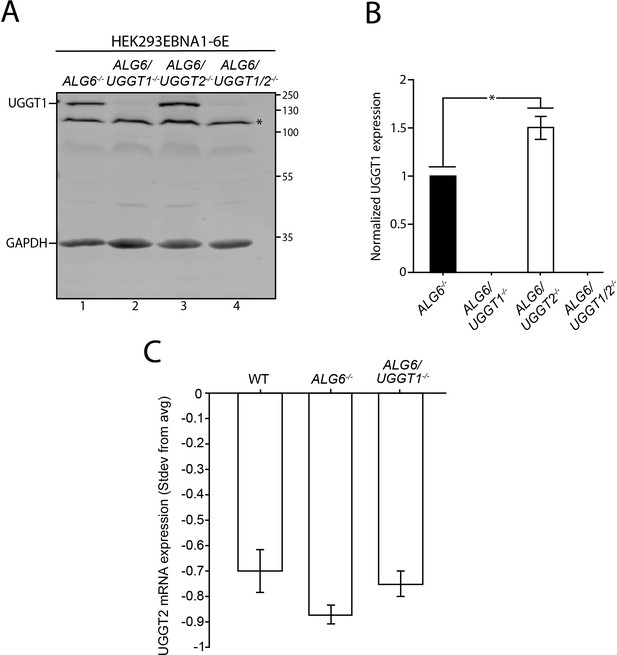
UDP-glucose:glycoprotein glucosyltransferase (UGGT)1 and UGGT2 expression.
(A) The indicated cells were lysed and whole cell lysates were resolved by SDS-PAGE and imaged by immunoblotting against UGGT1 and GAPDH. Asterisk denotes background band. Data are representative of three independent experiments with quantification shown in (B). UGGT1 expression was normalized to that of ALG6-/- cells. Error bars represent standard deviation. Asterisk denotes a p-value of less than 0.05. (C) Counts per million of UGGT2 mRNA generated by RNAseq from Supplementary file 4 was analyzed for the level of UGGT2 mRNA expression in the indicated cell lines. Counts per million of all genes were averaged and the standard deviation from the average for UGGT2 mRNA was determined. Error bars represent the standard deviation. Data are representative of three independent experiments.
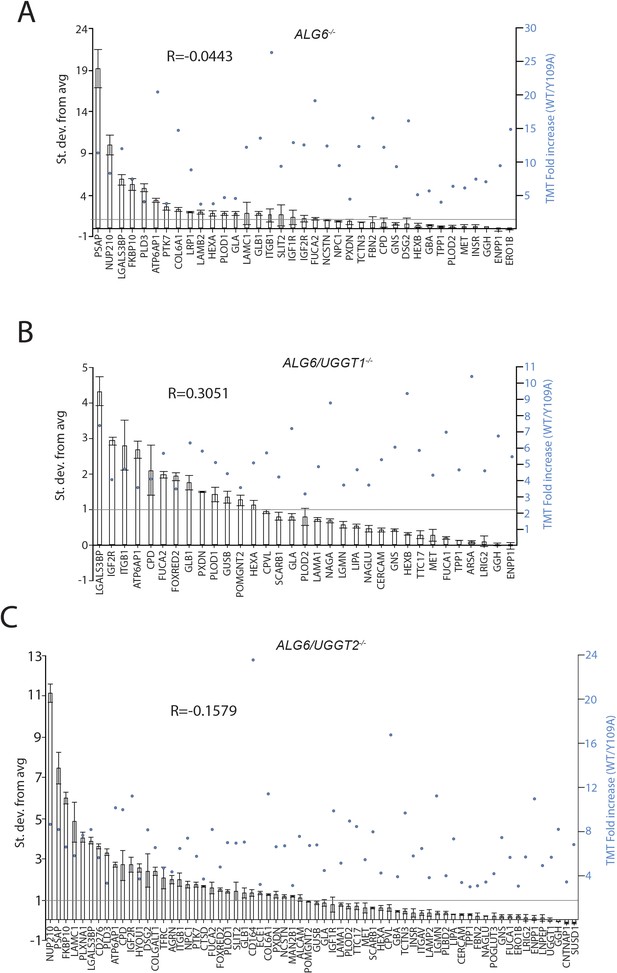
mRNA expression analysis of UDP-glucose:glycoprotein glucosyltransferase (UGGT)1 and UGGT2 substrates.
(A) Reglucosylated substrates identified in ALG6-/- (A), ALG6/UGGT1-/- (B), and ALG6/UGGT2-/- (C) cells were compared to the average expression for the N-glycoproteome in counts per million. The standard deviation from the average is plotted, with the error bars representing the standard deviation. Blue dots above each gene represent the level of fold increase (GST-CRT/GST-CRT-Y109A) found by tandem mass tag (TMT) mass spectrometry. The Pearson’s correlation coefficient (R) between the mRNA expression and TMT mass spectrometry fold increase is shown. Data is representative of three independent experiments.
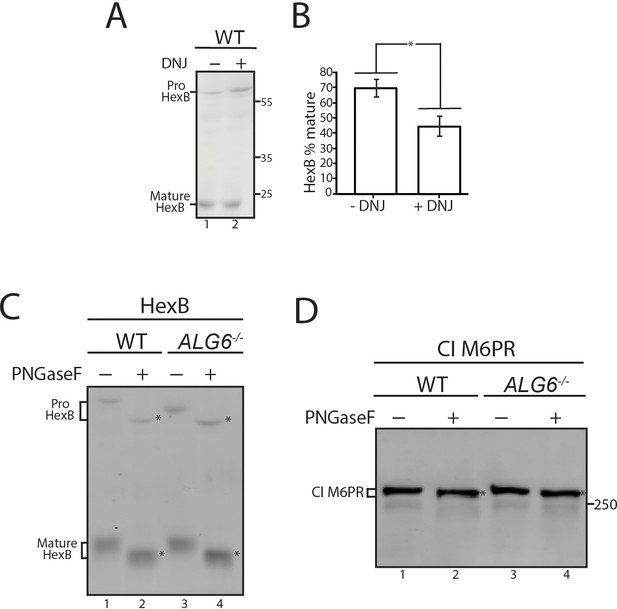
β-hexosaminidase subunit β trafficking and hypoglycosylation and CI-M6PR hypoglycosylation.
(A) Cells treated without or with deoxynojirimycin for 12 hr, lysed and whole cell lysate samples were resolved and imaged by immunoblotting against β-hexosaminidase subunit β. Data is representative of three independent experiments and quantification is shown in (B). (C) The indicated cell lines were lysed and samples were split evenly between non-treated and PNGaseF treated. Asterisks denote deglycosylated protein (D). As described for panel C, except immunoblotting was against CI M6PR.
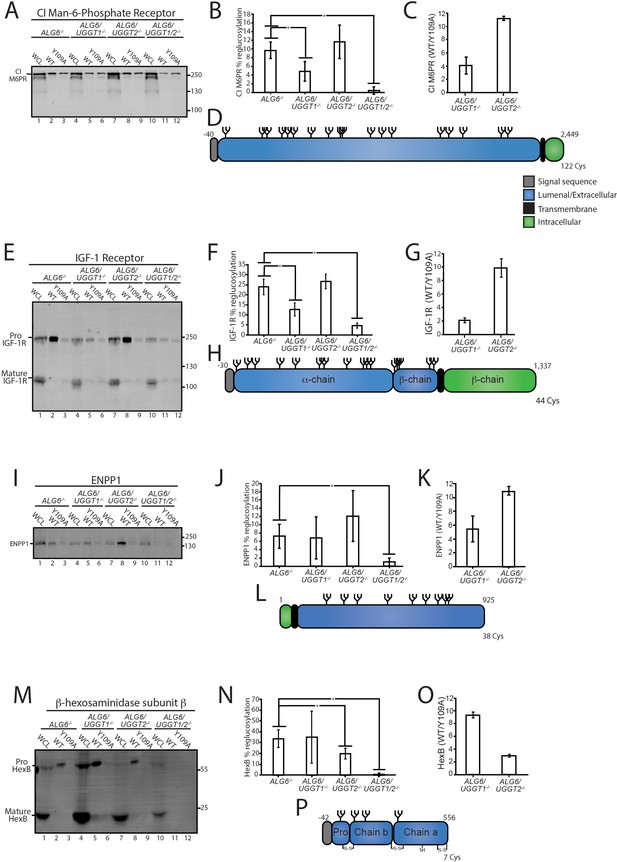
Validation of select reglucosylation substrates.
(A) The designated cell lines were lysed and split into whole cell lysate (WCL, 10%) or affinity purification by GST-CRT-WT or GST-CRT-Y109A and imaged by immunoblotting against the CI Man-6-Phosphate receptor. Data is representative of three independent experiments with quantification shown in panel (B). Quantifications were calculated by subtracting the value of protein in the Y109A lane from the value of protein in the associated wild-type (WT) lane, divided by the value of protein in the associated WCL lane and multiplied by 100. Error bars represent the standard deviation. Asterisks denote a p-value of less than 0.05. (C) Tandem mass tag (TMT) mass spectrometry quantification of CI Man-6-Phosphate receptor reglucosylation from ALG6/UGGT1-/- cells (Figure 2B) and ALG6/UGGT2-/- cells (Figure 2A). (D) Cartoon representation of CI Man-6-Phosphate receptor with N-glycans (branched structures), the signal sequence (gray), luminal/extracellular domain (blue), transmembrane domain (black), and intracellular domain (green) depicted. Number of amino acids and Cys residues are indicated. (E) Reglucosylation of IGF-1R, conducted as previously described above. Pro IGF-1R and mature IGF-1R are both observed due to proteolytic processing. Data are representative of three independent experiments with quantification displayed in (F). (G) TMT mass spectrometry quantification of IGF-1R from Figure 2A and B, as previously described. (H) Cartoon depiction of IGF-1R. (I) The reglucosylation of ENPP1 shown with quantification displayed in J. (K) TMT mass spectrometry quantification of ENPP1 from Figure 2A and B with cartoon depiction of ENPP1 in L. (M) Reglucosylation of β-hexosaminidase subunit β, conducted as previously described with quantifications displayed in N and TMT mass spectrometry quantification of β-hexosaminidase subunit β from Figure 2A and B in O with a cartoon depicting β-hexosaminidase subunit β in P.
-
Figure 3—source data 1
Quantifications for reglucosylation validations.
- https://cdn.elifesciences.org/articles/63997/elife-63997-fig3-data1-v2.xlsx
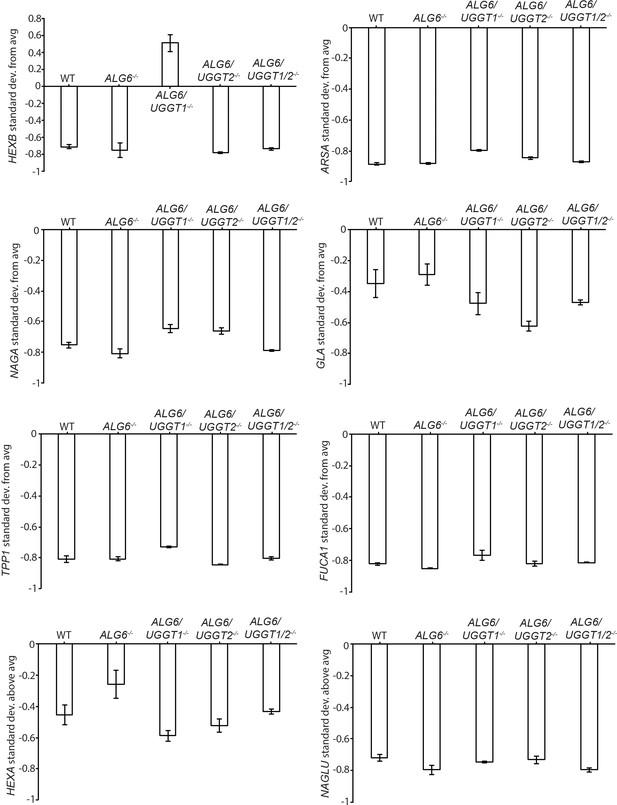
mRNA expression of lysosomal preferential UDP-glucose:glycoprotein glucosyltransferase (UGGT)2 substrates.
(A) The expression of HEXB (A), ARSA (B), NAGA (C), GLA (D), TPP1 (E), FUCA1 (F), HEXA (G), and NAGLU (H) in the indicated cell lines was analyzed by mRNA expression level in the denoted cell lines from RNAseq data presented in Supplementary file 4. The standard deviation from the average expression level in counts per million in each cell line for all genes is plotted. Error bars represent standard deviation. Data are representative of three independent experiments.
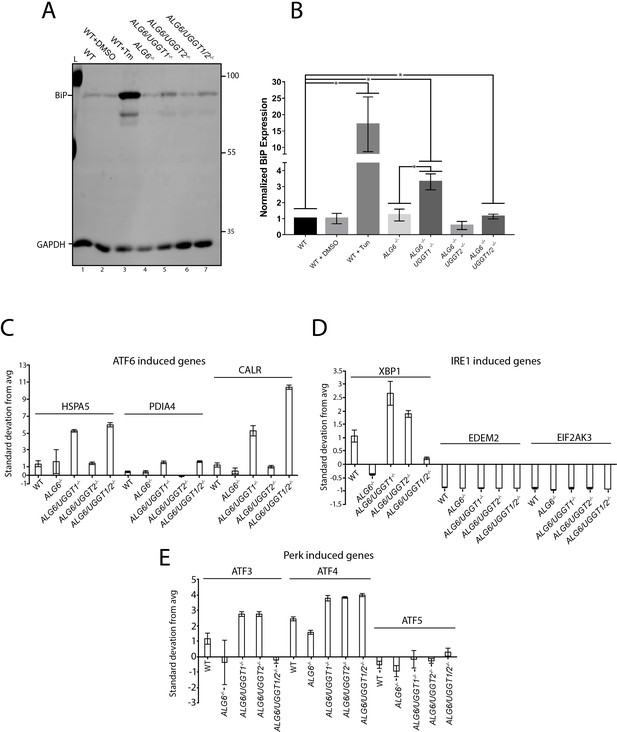
UPR induction in knockout cell lines.
(A) The indicated cells were lysed and whole cell lysate were resolved by SDS-PAGE and imaged by immunoblotting against BiP and GAPDH. DMSO was used as a vehicle control with tunicamycin (5 μg/ml) as a positive control. Data is representative of three independent experiments with quantification displayed in (B). BiP expression levels were normalized to that of wild-type cells and the corresponding GAPDH loading control. Error bars denote standard deviation. Asterisks denote a p-value of less than 0.05. (C) A subset of genes induced by the ATF6 UPR branch was analyzed by mRNA expression level as described in Figure 3—figure supplement 1. Data are representative of three independent experiments. IRE1 (D) and PERK (E) induced genes characterized as described in (C).
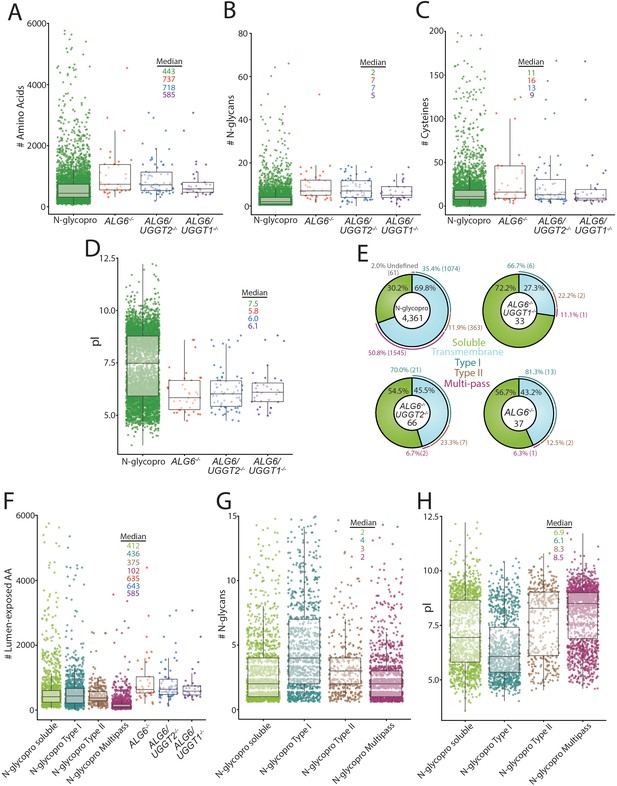
Analysis of substrates of the UDP-glucose:glycoprotein glucosyltransferase (UGGT)s and the N-glycoproteome.
(A) Amino acid lengths of each protein in the indicated data sets were visualized by scatter plot overlaid with a box and whisker plot. Amino acid number was obtained via UniprotKB. All scatter plots with box and whisker plots were generated using R and the ggplot package. The number of N-glycans (B) or Cys residues (C) for each protein in the indicated data sets was visualized by scatter plot overlaid with a box and whisker plot with the numbers determined using their UniprotKB annotation. (D) The isoelectric point (pI) values for each protein in the indicated data sets was visualized by scatter plot overlaid with a box and whisker plot. The pI values were obtained via ExPASy theoretical pI prediction. (E) The computationally predicted N-glycoproteome and the indicated reglucosylation substrates were determined as either soluble or transmembrane using UniprotKB annotations. The transmembrane portion of each data set was then analyzed for type I, type II, or multi-pass topology using the associated UniprotKB annotation. Proteins that were annotated by UniprotKB as transmembrane but lacked topology information were labeled as undefined. (F) The computationally determined N-glycoproteome was separated into soluble, type I, type II, and multi-pass transmembrane proteins using UniprotKB annotations. Luminally exposed amino acids were computationally determined using UniprotKB annotations for each subset of the N-glycoproteome and each indicated reglucosylation substrate data set. The resulting data was visualized by scatter plot overlaid with a box and whisker plot. (G) The indicated N-glycoproteome subsets were analyzed for N-glycan content using UniprotKB annotation and visualized by scatter plot overlaid with a box and whisker plot, as described. (H) The indicated N-glycoproteome subsets were analyzed for predicted pI using ExPASy theoretical pI prediction and visualized by scatter plot overlaid with a box and whisker plot.
-
Figure 4—source data 1
Characteristics of the N-glycoproteome.
- https://cdn.elifesciences.org/articles/63997/elife-63997-fig4-data1-v2.xlsx
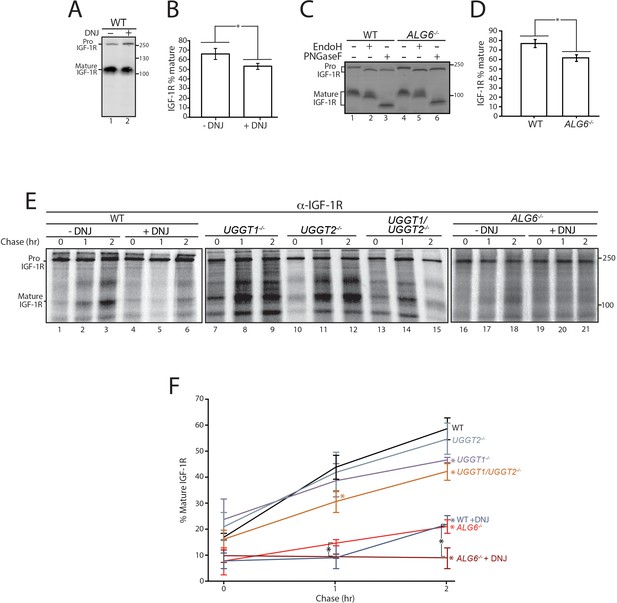
Calnexin/calreticulin cycle role for IGF-1R trafficking.
(A) Wild-type HEK293-EBNA1-6E cells treated without or with deoxynojirimycin (DNJ; 500 μM) for 12 hr were lysed and whole cell lysate samples were resolved by reducing 9% SDS-PAGE and imaged by immunoblotting against IGF-1R. Data are representative of three independent experiments with quantification shown in (B). Percent of IGF-1R mature was calculated by dividing the amount of mature protein by the total protein in each lane. Errors bars represent standard deviation. Asterisk denotes a p-value of less than 0.05. (C) The indicated cell lines were lysed in RIPA buffer. Samples were split evenly between non-treated and PNGaseF or EndoH treated. Samples were visualized by immunoblotting against IGF-1R and data are representative of three independent experiments with quantification displayed in (D). (E) Indicated cells were treated without or with DNJ, pulsed with [35S]-Met/Cys for 1 hr and chased for the indicated times. Cells were lysed and samples were immunoprecipitated using anti-β IGF-1R antibody and resolved by reducing SDS-PAGE and imaged by autoradiography. Data are representative of three independent experiments with quantification shown in (F).
-
Figure 5—source data 1
Quantifications for IGF-1R trafficking puse chase.
- https://cdn.elifesciences.org/articles/63997/elife-63997-fig5-data1-v2.xlsx
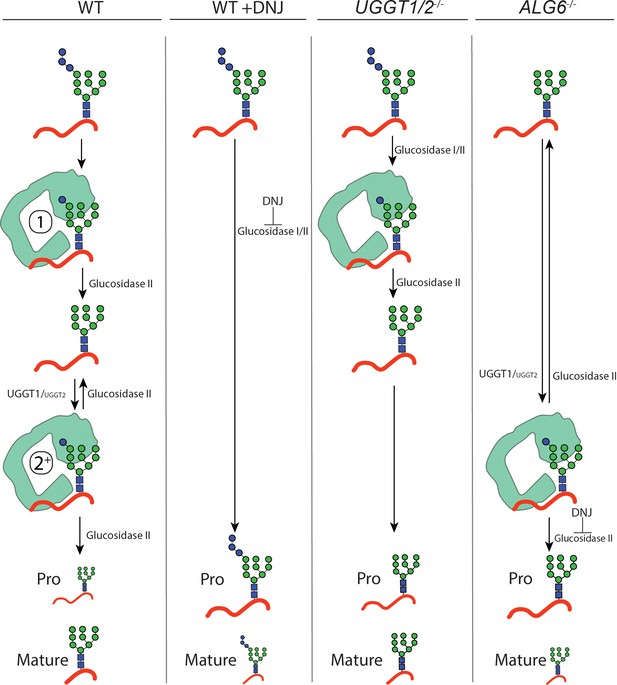
Model for IGF-1R engagement by the lectin chaperone cycle.
In wild-type (WT) cells, N-glycans with three terminal glucoses are appended to IGF-1R. Trimming of two terminal glucoses by glucosidases I/II generates a monoglucosylated protein that supports an initial round of interaction with calreticulin (calnexin not shown, denoted by a 1). Trimming of the final glucose by glucosidase II yields a non-glucosylated N-glycan. If recognized as non-native primarily by UDP-glucose:glycoprotein glucosyltransferase (UGGT)1, and to a lesser extent UGGT2, IGF-1R may then be reglucosylated, supporting a second round of interaction with calreticulin (denoted by a 2+). Multiple rounds of trimming, reglucosylation, and binding to calnexin or calreticulin can occur until proper folding and trafficking. Under this system, IGF-1R is efficiently trafficked from the ER and mature IGF-1R accumulates. When glucosidase I/II activity is inhibited by treatment with deoxynojirimycin (DNJ) in WT cells, all rounds of binding to the lectin chaperones are ablated and IGF-1R is retained in the ER, yielding primarily pro IGF-1R. In UGGT1/2-/- cells, initial binding to calnexin or calreticulin directed by glucosidases I/II trimming is maintained but rebinding via reglucosylation does not occur. Under this system, IGF-1R is inefficiently trafficked from the ER. In ALG6-/- cells, N-glycans are transferred without glucoses, eliminating the initial round of binding to calnexin or calreticulin by glucosidases trimming. Only the second round of binding is supported by UGGT1, and to a lesser extend UGGT2, mediated reglucosylation. Upon treatment with DNJ, reglucosylated IGF-1R may persistently interact with the lectin chaperones resulting in ER retention.
Tables
Reagent type (species) or resource | Designation | Source or reference | Identifiers | Additional information |
---|---|---|---|---|
Strain, strain background (Escherichia coli) | Top10 | Thermo Fisher | Cat# C404003 | Chemically competent |
Cell line (H. sapiens) | Hek293-EBNA1-6E | This paper | (RRID:CVCL_HF20) | Experimental results |
Cell line (H. sapiens) | Hek293-EBNA1-6E ALG6-/- | This paper | Experimental results | |
Cell line (H. sapiens) | Hek293-EBNA1-6E ALG6/UGGT1-/- | This paper | Experimental results | |
Cell line (H. sapiens) | Hek293-EBNA1-6E ALG6/UGGT2-/- | This paper | Experimental results | |
Cell line (H. sapiens) | Hek293-EBNA1-6E ALG6/UGGT1/2-/- | This paper | Experimental results | |
Cell line (H. sapiens) | Hek293-EBNA1-6E UGGT1-/- | This paper | Experimental results | |
Cell line (H. sapiens) | Hek293-EBNA1-6E UGGT2-/- | This paper | Experimental results | |
Cell line (H. sapiens) | Hek293-EBNA1-6E UGGT1/2-/- | This paper | Experimental results | |
Antibody | IGF-1 receptor β (D23H3) (rabbit monoclonal) | Cell Signaling | Cat # 9750 | WB (1:1000) IP (1:1000) |
Antibody | IGF-IIR/CI-M6PR (D3V8C) (rabbit monoclonal) | Cell Signaling | Cat# 14364 | WB (1:1000) |
Antibody | β-hexosaminidase subunit β (EPR7978) (rabbit monoclonal) | Abcam | Cat# (ab140649) | WB (1:500) |
Antibody | BiP (C50B12) (rabbit monoclonal) | Cell Signaling | Cat# 3177 | WB (1:1000) |
Antibody | ENPP1 (N2C2) (rabbit polyclonal) | Genetex | Cat# GTX103447 | WB (1:500) |
Antibody | UGGT1 (rabbit polyclonal) | Genetex | Cat# GTX66459 | WB (1:1000) |
Antibody | Glyceraldehyde 3-Phosphate (mouse monoclonal) | Millipore Sigma | Cat# (MAB374) | WB (1:1000) |
Recombinant DNA reagent | pGEX-3X-GST-CRT (plasmid) | Baksh and Michalak, 1991 | Available from the Hebert lab upon request | |
Recombinant DNA reagent | pGEX-3X-GST-CRT-Y109A (plasmid) | This paper | Available from the Hebert lab upon request | |
Recombinant DNA reagent | gh260 | Narimatsu et al., 2018 | RRID:Addgene_106851 | gRNA for ALG6-/- |
Recombinant DNA reagent | gh172 | Narimatsu et al., 2018 | RRID:Addgene_106833 | gRNA for UGGT1-/- |
Recombinant DNA reagent | gh173 | Narimatsu et al., 2018 | RRID:Addgene_106834 | gRNA for UGGT2-/- |
Recombinant DNA reagent | Cas9-GFP CAS9PBKS | Lonowski et al., 2017 | RRID:Addgene_68371 | Cas9 for CRISPR-mediated knockout |
Sequence-based reagent | CRT-Y109A_F | This paper | PCR primers | GGGGGCGGCGCCGTGAAGCT |
Sequence-based reagent | CRT-Y109A_R | This paper | PCR primers | CCGGAAACAGCTTCACGTAGCCGC |
Commercial assay or kit | TMT10plex, 0.8 mg | Thermo Fisher | Cat# 90110 | |
Commercial assay or kit | TMT6plex, 0.8 mg | Thermo Fisher | Cat# 90061 | |
Commercial assay or kit | BCA protein quantification kit | Pierce | Cat# 23227 | |
Commercial assay or kit | C18 tips | Pierce | Cat # 87784 | |
Commercial assay or kit | Quantitative colorimetric peptide assay | Pierce | Cat # 23275 |
Additional files
-
Source data 1
Number of glycans per 100 amino acids for UGGT substrates and N-glycoproteome.
- https://cdn.elifesciences.org/articles/63997/elife-63997-data1-v2.xlsx
-
Source data 2
Protein feature analysis of UGGT substrates and N-glycoproteome.
- https://cdn.elifesciences.org/articles/63997/elife-63997-data2-v2.xlsx
-
Supplementary file 1
UGGT1 and UGGT2 expression.
- https://cdn.elifesciences.org/articles/63997/elife-63997-supp1-v2.xlsx
-
Supplementary file 2
mRNA expression analysis of UGGT1 and UGGT2 substrates.
- https://cdn.elifesciences.org/articles/63997/elife-63997-supp2-v2.xlsx
-
Supplementary file 3
Beta-hexosminidase subunit beta expression trafficking and hypoglycosylation and CI-M6PR hypoglycosylation.
- https://cdn.elifesciences.org/articles/63997/elife-63997-supp3-v2.xlsx
-
Supplementary file 4
mRNA expression of lysosomal preferential UGGT2 substrates.
- https://cdn.elifesciences.org/articles/63997/elife-63997-supp4-v2.csv
-
Transparent reporting form
- https://cdn.elifesciences.org/articles/63997/elife-63997-transrepform-v2.docx