Gut microbe-targeted choline trimethylamine lyase inhibition improves obesity via rewiring of host circadian rhythms
Figures
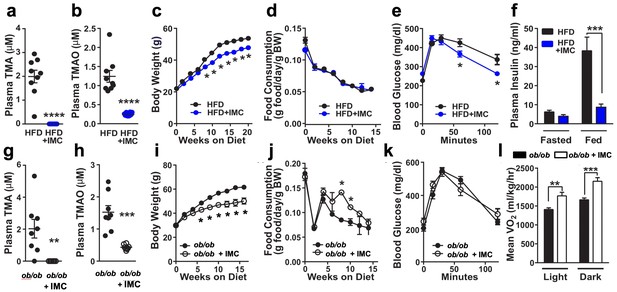
Small molecule choline trimethylamine (TMA) lyase inhibition improves obesity.
Panels (a–f) and (g–l) represent data from control and iodomethylcholine (IMC)-treated high fat diet (HFD)-fed and Lepob/ob mice (chow-fed), respectively. (a) and (g) Plasma TMA levels. (b) and (h) Plasma trimethylamine N-oxide (TMAO) levels. (c) and (i) Biweekly body weights. (d) and (j) Biweekly food consumption. (e) and (k) Glucose tolerance test. (f) Plasma insulin levels. (l) Average oxygen consumption. In panels (a–e) and (g–k), groups were compared using t-tests. In panels (f and l), groups were compared using two-way analysis of variance (ANOVA) with Tukey’s multiple comparisons test. Significance is defined as: *, p < 0.05. **, p < 0.01. ***, p < 0.001, ****, p < 0.0001. n = 6–10 per group.

Iodomethylcholine (IMC) does not confer antiobesogenic properties in a low fat diet (LFD) context.
Wildtype C57BL/6 mice were fed an LFD, LFD + 0.06% IMC in the drinking water, high fat diet (HFD), or HFD + 0.06% IMC in the drinking water starting at 6 weeks of age for a total of 16 weeks on diet. (a) Biweekly body weights and (b) food consumption. (c) Lean and fat mass after 16 weeks on diet represented in grams or (d) the percent body composition, respectively. In panels (c and d), groups were compared using two-way analysis of variance (ANOVA) with Tukey’s multiple comparisons test. Error bars represent mean ± SEM (n = 10 per group).
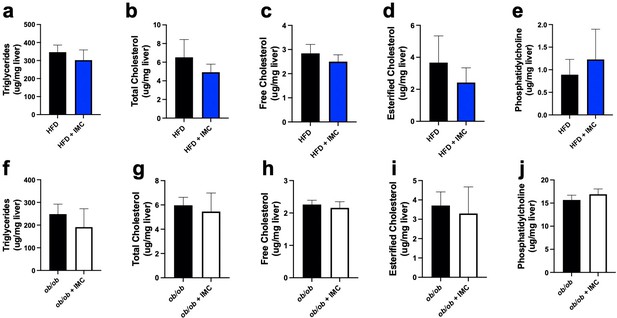
Iodomethylcholine (IMC) treatment does not alter hepatic steatosis in wildtype or genetically obese mice.
Panels (a–e) and (f–j) represent data from control and IMC-treated high fat diet (HFD)-fed and Lepob/ob mice (chow-fed), respectively. (a and f) Hepatic triglyceride levels. (b and g) Hepatic total cholesterol. (c and h) Hepatic-free cholesterol. (d and i) Hepatic esterified cholesterol. (e and j) Hepatic phosphatidylcholine. Panels (a–e) and (f–j) represent data from animals on diet for 20 and 16 weeks, respectively. Error bars represent mean ± SD (n = 6 per group).
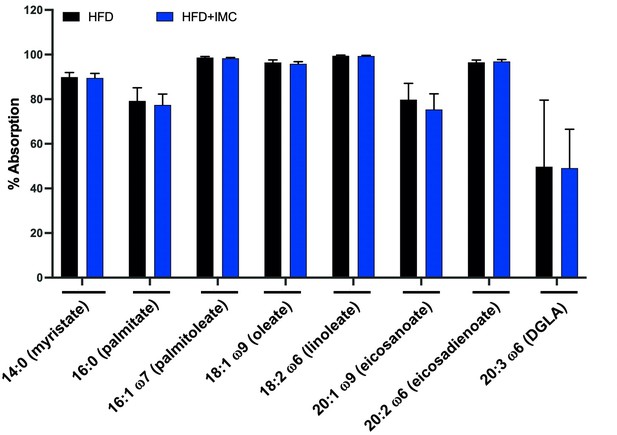
Iodomethylcholine (IMC) treatment does not impact intestinal fat absorption.
After 10 weeks on high fat diet (HFD) alone or HFD-containing IMC, mice were single housed on wire bottom cages and fed 60% HFD with 5% sucrose polybehenate added (Research Diets, D19010910). During this period, mice on the HFD + IMC diet were provided with IMC in their drinking water. All feces was collected at the end of the 72 hr feeding study and dried (n = 15 per group).
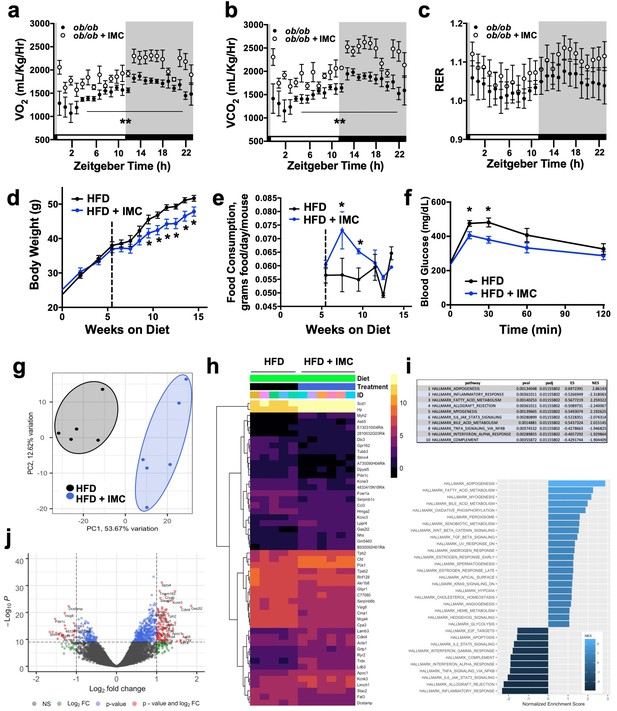
Choline trimethylamine (TMA) lyase inhibition increases energy expenditure and alters gene expression in white adipose tissue.
Related to main text Figure 1. Panels (a–c) represent indirect calorimetry data from control and iodomethylcholine (IMC)-treated Lepob/ob mice. (a) Oxygen consumption. (b) CO2 production. (c) Respiratory exchange ratio collected in the Oxymax CLAMS metabolic cage system. (d–f) C57BL6 mice fed a high fat diet (HFD) for 6 weeks to establish obesity (body weight >35 g); after 6 weeks of diet-induced obesity (DIO) mice were continued on HFD alone of HFD-containing IMC for another 10 weeks to test whether IMC can improve obesity-related phenotypes. (d) Body weight curve. (e) Food consumption after drug administration. (f) Glucose tolerance testing 8 weeks following IMC administration. (g–k) Male C57BL6/J mice were fed an HFD for 20 weeks and then gonadal white adipose tissue was collected for examination of global transcriptome alterations via RNA sequencing. (g) Principal component analysis. (h) Heatmap of most significantly altered mRNA transcripts. (i) Hallmark pathway analysis of differentially expressed genes (DEGs). (j) Volcano plot showing most significantly up- and down-regulated transcripts.
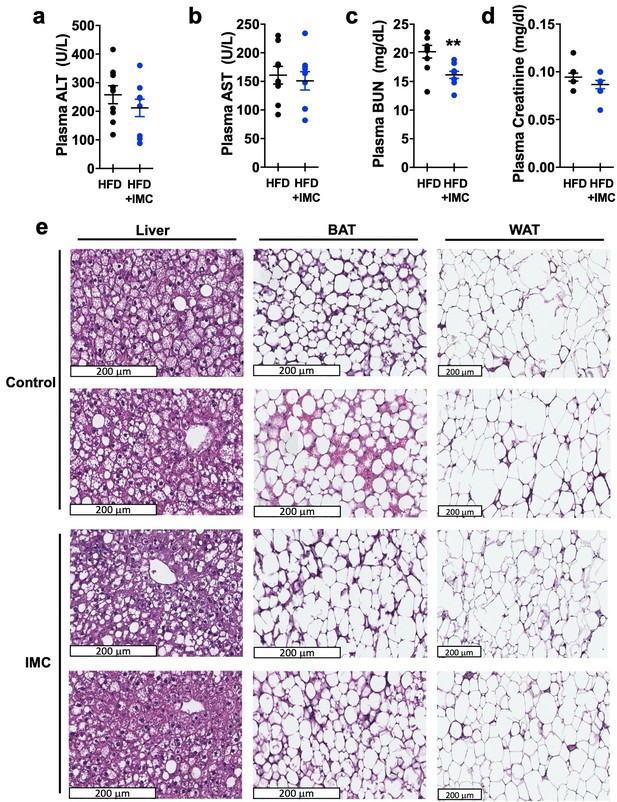
Iodomethylcholine (IMC) supplementation does not lead to morphological changes in liver or adipose tissues and does not promote hepatic or renal toxicity.
Wildtype male C57BL/6 mice were fed a high fat diet (HFD) or HFD + 0.06% IMC starting at 6 weeks of age for a total of 20 weeks on diet. (a–b) Plasma alanine aminotransferase (ALT) and aspartate transaminase (AST) were measured after 20 weeks on diet. (c, d) Plasma blood urea nitrogen (BUN) and creatinine were measured after 20 weeks on diet. (e) Six-week-old male Lepob/ob mice were fed a chow diet or chow + 0.06% IMC for 16 weeks at which point liver, white adipose tissue (WAT), and brown adipose tissue (BAT) were collected and stained with H&E after formalin fixation.

Small molecule trimethylamine (TMA) lyase inhibition alters the gut microbiome.
Panels (a–e) and (f–i) represent data from control and iodomethylcholine (IMC)-treated high fat diet (HFD)-fed and Lepob/ob mice (chow-fed), respectively. (a and f) Principal coordinate analysis plot of microbiota profiles built from weighted Unifrac distances. Each point represents a single sample from a single mouse. Positions of points in space display dissimilarities in the microbiota, with points further from one another being more dissimilar. (b and g) Barplot of cecal microbiota at the phylum level. Each bar represents an individual mouse and each color an individual phylum. (c) Relative abundance of Firmicutes, Bacteroidetes, and the Firmicutes to Baceteroidetes ratio. The boxes represent the 25th and 75th quartiles, and the line displays the median value within each group. Points extending beyond the lines are outliers defined as values greater or less than 1.5 times the interquartile range. (d and h) Linear discriminatory analyses plot of taxa differing significantly with IMC treatment. (e and i) Correlation between taxa and plasma TMA levels, body weight, and glucose tolerance (HFD-fed mice only). Values in both X and Y directions are plotted as mean ± SEM.
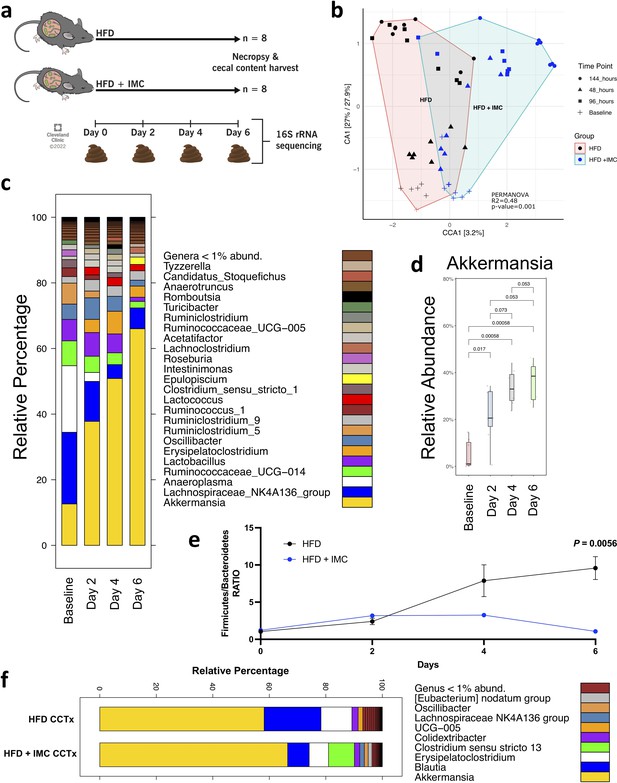
Iodomethylcholine (IMC) supplementation leads to rapid kinetic changes in the gut microbiome community structure.
Wildtype male C57BL/6 mice were fed a high fat diet (HFD) control diet, or HFD + 0.06% IMC for 6 days. Fecal pellets were collected every 48 hr for 6 days for 16S rRNA sequencing analysis. (a) Schematic representation of experimental design. (b) Principal coordinate analysis comparing HFD vs. HFD+ IMC beta diversity of fecal 16S rRNA profiles (n = 7–8). (c) Stacked bar chart representing genera-level relative abundance of the HFD + IMC fecal microbiome over time (n = 7–8). (d) Relative abundance of Akkermansia within the HFD + IMC fecal microbiome over time with statistical analysis performed using analysis of variance (ANOVA) (n = 7–8). (e) Firmicutes to Bacteroidetes ratio over time after cleansing data of statistical outliers using the ROUT method (Q = 1%). Significance between groups was calculated using Student’s t-test with Welch correction and Holm-Šídák method for multiple comparisons. Data are presented as mean with error bars representing SEM (n = 4–7). (f) Stacked bar chart representing the pooled cecal contents of HFD and HFD + IMC mice to be used for gnotobiotic colonization studies (n = 8).

Trimethylamine (TMA) lyase inhibition beneficially improves circadian oscillations in gut microbial communities.
Related to main text Figure 2. Wildtype male C57BL/6J mice were fed chow or chow supplemented with the choline TMA lyase inhibitor iodomethylcholine (IMC) for 7 days. Mice were then necropsied at 4 hr intervals to collect cecum for 16S-based microbiome analyses. (a) Principal coordinate analysis (Bray-Curtis) comparing chow vs. chow + IMC, light cycle vs. dark cycle, and across all zeitgeber (ZT) time points. (b) Identification of cyclic taxa whose relative abundance also differed significantly between groups (highlighted in yellow). FDR-corrected p-values for treatment effect (two-way analysis of variance [ANOVA]) are shown. Circadian rhythmicity of cecal microbial taxa was analyzed using JTK_Cycle. (c) Relative abundance of key IMC-altered taxa that are circadian in nature (n = 7–8); significance between groups at specified ZT time points was compared using Student’s t-test (p < 0.05).
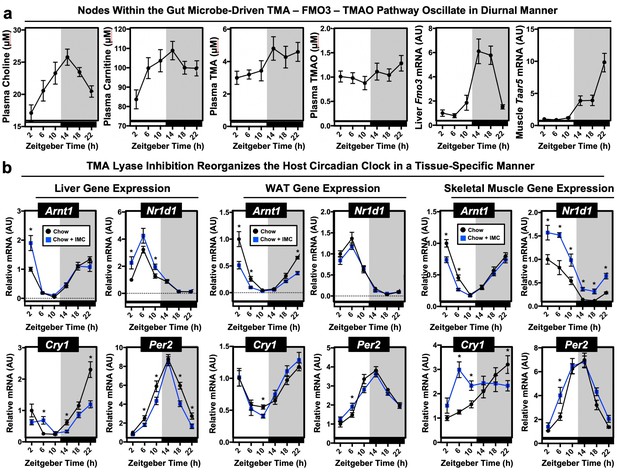
Nodes within the metaorganismal trimethylamine N-oxide (TMAO) pathway show diurnal rhythmicity and choline trimethylamine (TMA) lyase inhibition alters the host circadian clock.
Wildtype male chow-fed C57BL/6J mice were necropsied at 4 hr intervals to collect plasma and tissue over a 24 hr period. (a) Plasma choline, L-carnitine, TMA, TMAO were quantified by liquid chromatography with online tandem mass spectrometry (LC-MS/MS); hepatic expression of Fmo3 mRNA or gastrocnemius muscle expression of Taar5 mRNA was quantified via quantitative PCR (qPCR) (n = 7–8). (b) Wildtype male C57BL/6J mice were fed chow or chow supplemented with the choline TMA lyase inhibitor iodomethylcholine (IMC) for 7 days. Mice were then necropsied at 4 hr intervals to collect liver, gonadal white adipose tissue, or gastrocnemius skeletal muscle. We then performed qPCR to examine the mRNA expression in liver, white adipose, and gastrocnemius skeletal muscle of key circadian clock regulators including aryl hydrocarbon receptor nuclear translocator like (Arntl; BMAL1), nuclear receptor subfamily one group D member 1 (Nr1d1; RevErbα), cryptochrome 1 (Cry1), or period 2 (Per2) (n = 7–8). Significance (p < 0.05) between diet groups at specified zeitgeber (ZT) time points was compared using Student’s t-tests.
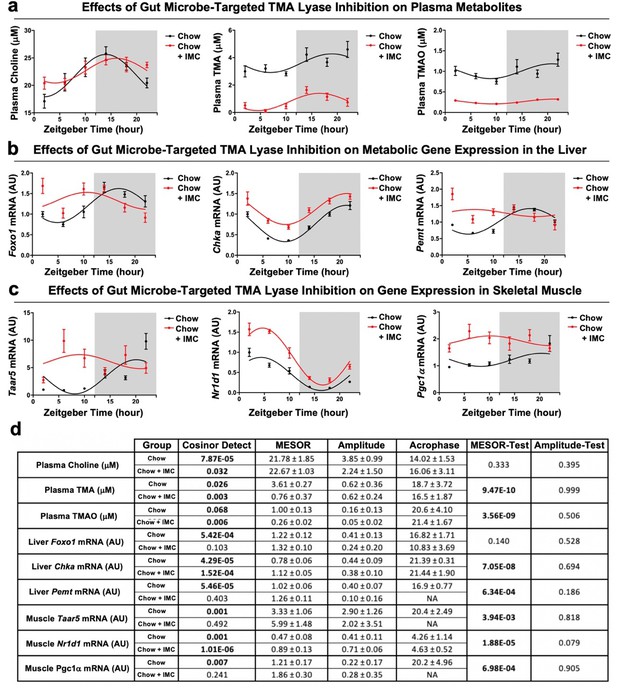
Trimethylamine (TMA) lyase inhibition alters the circadian rhythmicity of host metabolism-associated gene expression.
Related to main text Figure 3. Wildtype male C57BL/6J mice were fed chow or chow supplemented with the choline TMA lyase inhibitor iodomethylcholine (IMC) for 7 days. Mice were then necropsied at 4 hr intervals to collect blood and metabolic tissues (liver and gastrocnemius skeletal muscle). (a) Plasma levels of choline, TMA, and trimethylamine N-oxide (TMAO). (b) The relative mRNA expression of forkhead box protein O1 (Foxo1), choline kinase α (Chkα), phosphatidylethanolamine N-methyltransferase (Pemt) in the liver over a 24 hr period. (c) The relative mRNA expression of the TMA receptor trace amine-associated receptor 5 (Taar5), nuclear receptor subfamily one group D member 1 (Nr1d1; RevErbα), and peroxisome proliferator-activated receptor gamma coactivator 1α (Pgc1α) in the gastrocnemius muscle over a 24 hr period. (d) Circadian parameters (MESOR, amplitude, and acrophase) were determined by cosinor analysis with a 24 hr period in plasma, liver, and skeletal muscle. The overall fit for each model is shown in cosinor detect, and a Wald multivariate test was used to compare circadian parameters between groups. All data represent mean ± SEM (n = 7–8 per group).

Iodomethylcholine (IMC) treatment significantly alters the circadian oscillations in metabolic hormones and gene expression in high fat diet (HFD)-fed mice.
Wildtype C57BL/6J mice were fed a low fat diet (LFD), HFD, or HFD supplemented with the choline trimethylamine (TMA) lyase inhibitor IMC for 5 weeks. Panels (a–c) Plasma insulin (a), leptin (b), and adiponectin (c) levels at different time points after 5 weeks on diet. Panels (d–f) Hepatic mRNA levels for (d) cryptochrome 1 (Cry1), (e) carnitine palmitoyl transferase 1α (Cpt1α), and (f) betaine homocysteine methyltransferase (Bhmt). Panels (g–i) Skeletal muscle mRNA levels for (g) cryptochrome 1 (Cry1), (h) cryptochrome 2 (Cry2), and (i) period 2 (Per2). * = Significantly different (p < 0.05) compared to the HFD control group at specified zeitgeber (ZT) time points was compared using Student’s t-tests (n = 5 per group, per time point).
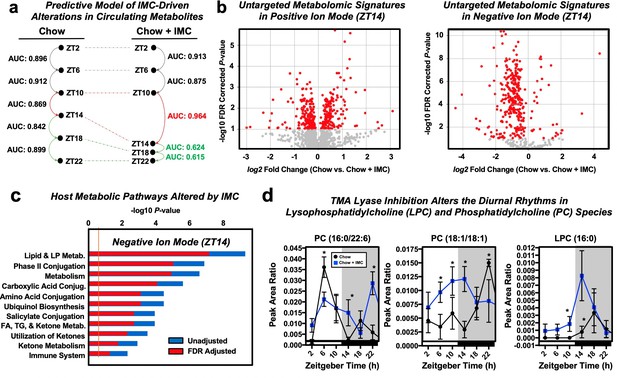
Small molecule inhibition of choline trimethylamine (TMA) lyases impacts circadian oscillations in choline-containing phospholipids in the host circulation.
Wildtype male C57BL/6J mice were fed chow or chow supplemented with the choline TMA lyase inhibitor iodomethylcholine (IMC) for 7 days. Mice were then necropsied at 4 hr intervals to collect blood and tissue, and plasma time points were subjected to liquid chromatography with online tandem mass spectrometry (LC-MS/MS)-based untargeted metabolomics (n = 7–8). (a) Selective paired ion contrast analysis (SPICA) (Lieber et al., 2019) was used to identify time points where the plasma metabolome was most dramatically altered by IMC treatment compared to zeitgeber-matched controls. Pairwise analysis was conducted between all adjacent time points for the control and IMC treatment groups, resulting in a total of 10 comparisons made (five for Chow and five for Chow + IMC). Differences in the global plasma metabolome for each pairwise comparison were quantified via receiver operating characteristic (ROC) curve construction and area under the curve (AUC) calculations via Monte Carlo cross validation procedures in SPICA (Lieber et al., 2019) revealed that IMC most dramatically altered the plasma metabolome during the transition from ZT10 to ZT14. As a result, subsequent data analyses focused on this T10 to T14 transition, which also coincided with the light to dark phase transition and when the mice began to eat. (b) Volcano plots of the significantly altered metabolites (red) in positive and negative ion mode at the ZT14 time point. (c) Pathway analysis of data collected in negative ion mode at ZT14 reveals that IMC alters host lipid and lipoprotein metabolism among other metabolic pathways. (d) Relative plasma levels of phosphatidylcholine (PC) and lysophosphatidylcholine (LPC) species are altered by IMC.
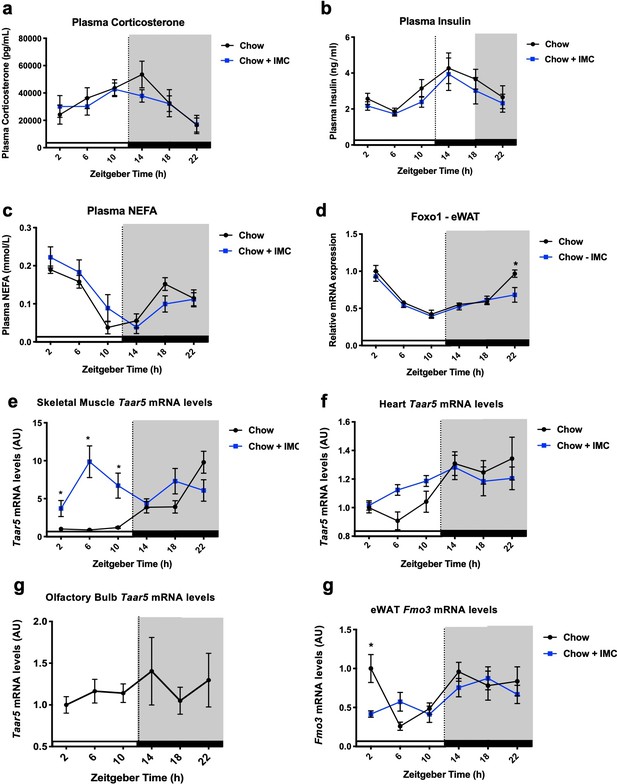
Choline trimethylamine (TMA) lyase inhibition impact on the circadian rhythmicity of host hormone levels and gene expression.
Related to main text Figure 4. Wildtype male C57BL/6J mice were fed chow or chow supplemented with the choline TMA lyase inhibitor iodomethylcholine (IMC) for 7 days. Mice were then necropsied at 4 hr intervals to collect blood/plasma. (a) Plasma corticosterone levels were measured via ELISA. (b) Plasma insulin levels were measured by ELISA. (c) Plasma non-esterified fatty acid (NEFA) levels were measured via enzymatic assay. (d) Foxo1 mRNA levels in epidydimal white adipose tissue (eWAT). (e) Taar5 mRNA levels in gastrocnemius skeletal muscle. (f) Taar5 mRNA levels in the heart. (g) Taar5 mRNA levels in the olfactory bulb in chow-fed mice. (h) Fmo3 mRNA level in eWAT. Significance between groups (n = 7–8) at each zeitgeber time point was compared using Student’s t-test (p < 0.05).
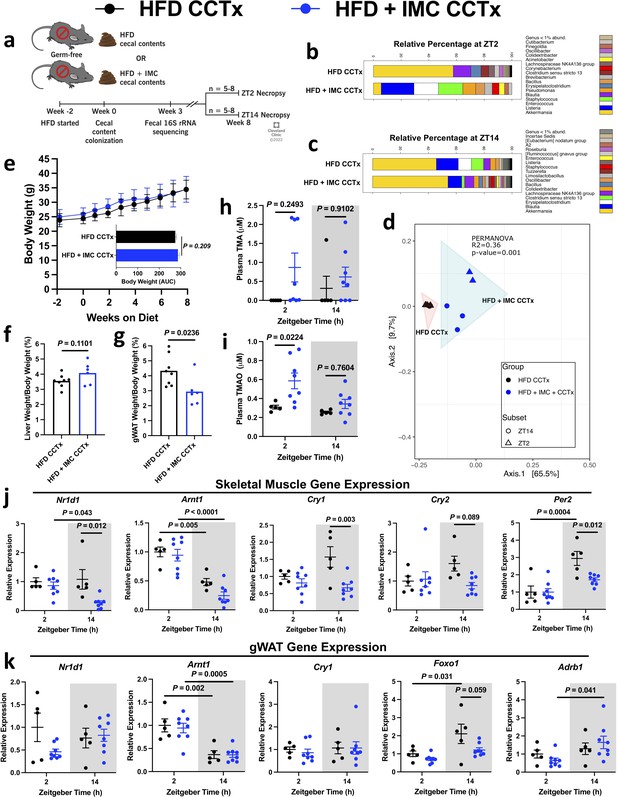
Cecal content transplant into germ-free mice informs adiposity and displays community-level circadian rhythmicity.
Germ-free male B6/N mice were placed on a high fat diet (HFD) 2 weeks prior to cecal content gavage from mice fed either HFD or HFD + iodomethylcholine (IMC) for 6 days. (a) Schematic representation of experimental design. (b) Stacked bar chart representing circadian rhythmicity at ZT2 and (c) ZT14 within the HFD cecal contents transplant (HFD CCTx) and HFD + IMC cecal contents transplant (HFD + IMC CCTx) groups (n = 3–5). (d) Principal component analysis comparing HFD CCTx vs. HFD + IMC CCTx beta diversity of cecal 16S rRNA profiles at ZT2 and ZT14 8 weeks post-colonization (n = 2–5). (e) Weekly body weights with area under the curve (n = 8–10, error bars represent SD). (f) Liver and (g) gWAT weights as expressed as a percentage of total body weight (n = 6–8). (h) Plasma trimethylamine (TMA) and (i) trimethylamine N-oxide (TMAO) concentrations (n = 5–8). (j) Quantitative PCR (qPCR) analysis of key circadian rhythmicity genes in skeletal muscle (n = 5–8). (k) qPCR analysis of key circadian rhythmicity genes in gWAT (n = 5–8). Statistical analysis in panels (e–g) was performed using Student’s two-tailed t-test. Statistical analysis in panels (h–k) was performed using two-way analysis of variance (ANOVA) with Tukey’s multiple comparisons test.
Tables
Reagent type (species) or resource | Designation | Source or reference | Identifiers | Additional information |
---|---|---|---|---|
Strain, strain background (Mus musculus) | C57BL/6J | Jackson Laboratory | Stock #: 000664; RRID:MGI:3028467 | |
Strain, strain background (Mus musculus) | C57BL/6NCrl | Charles River | Stock #: 574 RRID:MGI:2159965 | |
Genetic reagent (Mus musculus) | B6.Cg-Lepob/J | Jackson Laboratory | Stock #: 000632; RRID:MGI:6719537 | |
Sequence-based reagent | Fmo3 | Sigma | F: CCCACATGCTTTGAGAGGAGR: GGAAGAGTTGGTGAAGACCG | |
Sequence-based reagent | Taar5 | Sigma | F: AAAGAAAAGCTGCCAAGAR: AAGGGAAGCCAACACACA | |
Sequence-based reagent | Arntl1 | Sigma | F: CCAAGAAAGTATGGACACAGACAAAR: GCATTCTTGATCCTTCCTTGGT | |
Sequence-based reagent | Nr1d1 | Sigma | F: ATGCCAATCATGCATCAGGTR: CCCATTGCTGTTAGGTTGGT | |
Sequence-based reagent | Cry1 | Sigma | F: TACTGGGAAACGCTGAACCCR: ACCCCAAGCTTGTTGCCTAA | |
Sequence-based reagent | Cry2 | Sigma | F: GCTGGAAGCAGCCGAGGAACCR: GGGCTTTGCTCACGGAGCGA | |
Sequence-based reagent | Per2 | Sigma | F: GCTGACGCACACAAAGAACTR: TAGCCTTCACCTGCTTCACG | |
Sequence-based reagent | Foxo1 | Sigma | F: GAGCCTCCTTCAAACAGAGTAGR: GCAAATGGTAAGAAATGGCAGAG | |
Sequence-based reagent | Adrb1 | Sigma | F: TGGGAGTGTGGCTTAGTAR: AATGCCTGAAGGTGCATTAAAC | |
Sequence-based reagent | Chka | Sigma | F: CTGATAGCATGGCTGGGGTTR: CCCAAATCAAGCTCACACACC | |
Sequence-based reagent | Pemt | Sigma | F: TGTGCTGTCCAGCTTCTATGR: GAAGGGAAATGTGGTCACTCT | |
Sequence-based reagent | Pgc1a | Sigma | F: CCCTGCCATTGTTAAGACR: GCTGCTGTTCCTGTTTTC | |
Sequence-based reagent | CycloA | Sigma | F: GCGGCAGGTCCATCTACGR: GCCATCCAGCCATTCAGTC | |
Sequence-based reagent | Gapdh | Sigma | F: CCTCGTCCCGTAGACAAAATGR: TGAAGGGGTCGTTGATGGC | |
Commercial assay or kit | Ultra Sensitive Mouse Insulin ELISA | Crystal Chem Inc | 90080 | |
Commercial assay or kit | Mouse Leptin ELISA | Crystal Chem Inc | 90030 | |
Commercial assay or kit | Liver Triglycerides | Wako | 994–02891 | |
Commercial assay or kit | Free Cholesterol | Wako | 993–02501 | |
Commercial assay or kit | Phospholipid C | Wako | 433–26301 | |
Commercial assay or kit | Total Cholesterol | Fisher Scientific | TR134321 | |
Commercial assay or kit | Mouse corticosterone kit | Crystal Chem Inc | 80556 | |
Commercial assay or kit | NEFA Kit | Wako | 999–34691, 995–34791, 991–34891, 993–35191, 276–76491 | |
Chemical compound, drug | Iodomethylcholine | This paper | See Materials and methods for synthesis protocol |
Donor Cecal Pool | # Donor OTUs | # Matching Recipient OTUs (ZT2 + ZT14) | % Engraftment Efficiency |
---|---|---|---|
HFD | 30 | 29 | 96.67 |
HFD + IMC | 32 | 26 | 81.25 |