A novel ATP dependent dimethylsulfoniopropionate lyase in bacteria that releases dimethyl sulfide and acryloyl-CoA
Figures
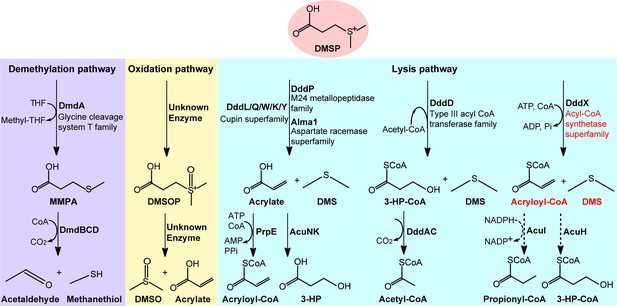
Metabolic pathways for DMSP degradation.
Different pathways are shown in different colors. The demethylation of DMSP by DmdA produces MMPA (in purple). The oxidation of DMSP produces DMSOP (in yellow). In the lysis pathway (in blue), DMSP lyase DddP, DddL, DddQ, DddW, DddK, DddY, or Alma1 converts DMSP to acrylate and DMS, DddD converts DMSP to 3-HP-CoA and DMS, using acetyl-CoA as a CoA donor, and the newly identified DddX in this study converts DMSP to acryloyl-CoA and DMS, with ATP and CoA as co-substrates. Dotted lines represent unconfirmed steps of the DddX DMSP lysis pathway that we propose in this study. The protein families of enzymes involved in the first step of each pathway are indicated. The protein family of DddX and the products of its catalysis are highlighted in red color. THF, tetrahydrofolate; MMPA, methylmercaptopropionate; 3-HP, 3-hydroxypropionate; DMSOP, dimethylsulfoxonium propionate; DMSO, dimethylsulfoxide.
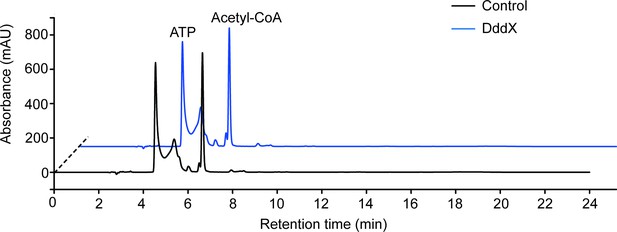
Enzymatic activity analysis of the recombinant DddX using acetyl-CoA as a CoA donor.
ATP and acetyl-CoA were analyzed by HPLC through its ultraviolet absorbance under 260 nm. The result showed that DddX failed to catalyze the degradation of DMSP when acetyl-CoA was used as a CoA donor.
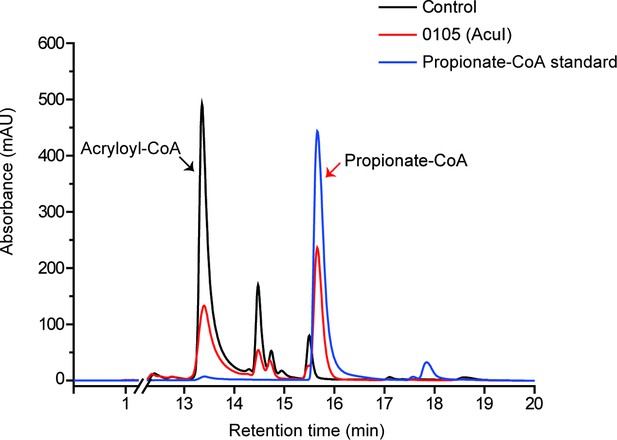
HPLC assay of the enzymatic activity of 0105 protein on acryloyl-CoA at 260 nm.
The peak of acryloyl-CoA was indicated with black arrow and the peak of propionate-CoA was indicated with red arrow. The recombinant 0105 could catalyze the conversion of acryloyl-CoA to propionate-CoA (718.3 ± 59.2 pmol propionate-CoA min–1 mg protein–1). The reaction system without 0105 protein was used as the control.
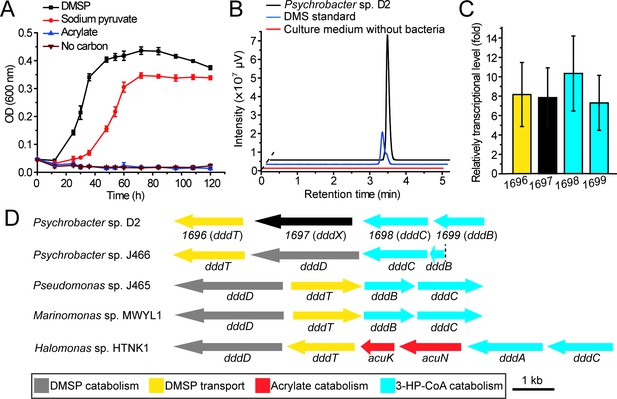
The utilization of DMSP by Psychrobacter sp. D2 and the putative DMSP-catabolizing gene cluster in its genome.
(A) The growth curve of Psychrobacter sp. D2 on DMSP, sodium pyruvate or acrylate as sole carbon source (5 mM) at 15°C. The error bar represents standard deviation of triplicate experiments. (B), GC detection of DMS production from DMSP by strain D2. The culture medium without bacteria was used as the control. The DMS standard was used as a positive control. Psychrobacter sp. D2 could catabolize DMSP and produce DMS (44.8 ± 1.8 nmol DMS min–1 mg protein–1). (C), RT-qPCR assay of the transcriptions of the genes 1696, 1697, 1,698, and 1,699 in Psychrobacter sp. D2 in response to DMSP in the marine broth 2,216 medium. The bacterium cultured without DMSP in the same medium was used as the control. The recA gene was used as an internal reference. The error bar represents standard deviation of triplicate experiments. The locus tags of 1696, 1697, 1,698, and 1,699 are H0262_08195, H0262_08200, H0262_08205, and H0262_08210, respectively. (D), Genetic organization of the putative DMSP-catabolizing gene cluster. Reported DMSP catabolic/transport gene clusters from Psychrobacter sp. J466, Pseudomonas sp. J465, Marinomonas sp. MWYL1, and Halomonas sp. HTNK1 are shown (Todd et al., 2007; Todd et al., 2010; Curson et al., 2010; Curson et al., 2011b). The dashed vertical line indicates a breakpoint in dddB in the cosmid library of Pseudomonas sp. J466 (Curson et al., 2010).
-
Figure 2—source data 1
The growth curve of Psychrobacter sp. D2 on DMSP, sodium pyruvate or acrylate as sole carbon source.
- https://cdn.elifesciences.org/articles/64045/elife-64045-fig2-data1-v2.xlsx
-
Figure 2—source data 2
GC detection of DMS production from DMSP by strain D2.
- https://cdn.elifesciences.org/articles/64045/elife-64045-fig2-data2-v2.xlsx
-
Figure 2—source data 3
RT-qPCR assay of the transcriptions of the genes 1696, 1697, 1,698, and 1,699 in Psychrobacter sp. D2.
- https://cdn.elifesciences.org/articles/64045/elife-64045-fig2-data3-v2.xlsx
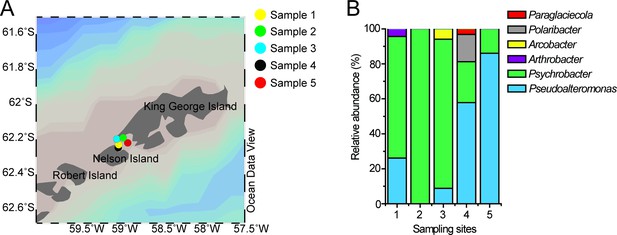
Locations of the sampling sites and the relative abundance of DMSP-catabolizing bacteria isolated from the samples.
(A), Locations of the sampling sites in the Antarctic. Stations were plotted using Ocean Data View (Schlitzer, 2002). (B), The relative abundance of DMSP-catabolizing bacteria isolated from the Antarctic samples. The detailed information of the samples is shown in Supplementary file 1a.
-
Figure 2—figure supplement 1—source data 1
The number of DMSP-catabolizing strains isolated from the Antarctic samples.
- https://cdn.elifesciences.org/articles/64045/elife-64045-fig4-data4-v2.xls
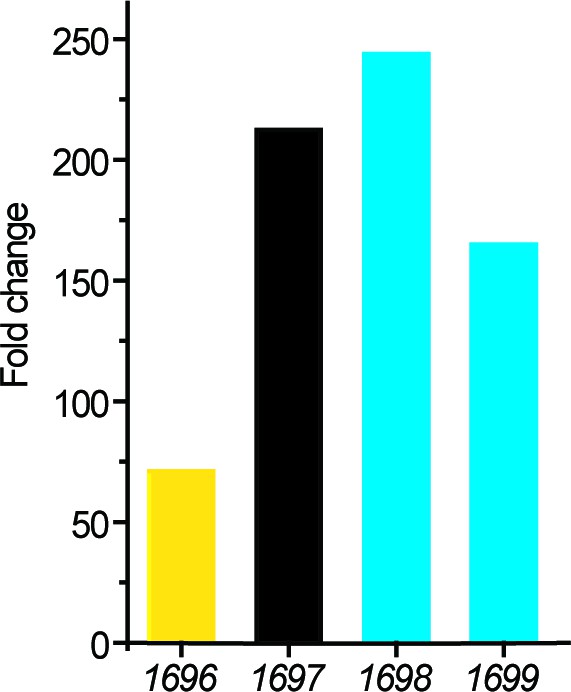
Transcriptomic analysis of the putative genes involved in DMSP metabolism in strain D2.
The transcriptions of four genes in a cluster were significantly upregulated during the growth of strain D2 on DMSP. The fold changes were calculated by comparing to the control (transcriptions of these genes during the strain growth on sodium pyruvate).
-
Figure 2—figure supplement 2—source data 1
Transcriptomic analysis of the putative genes involved in DMSP metabolism in strain D2.
- https://cdn.elifesciences.org/articles/64045/elife-64045-fig5-data5-v2.xls
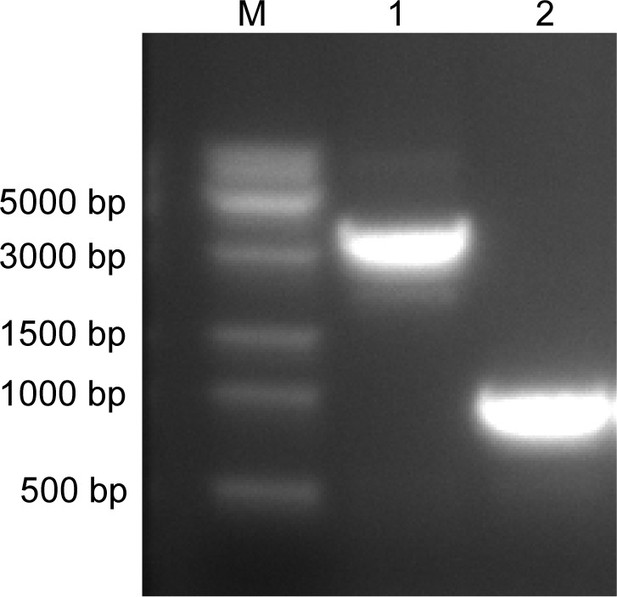
Confirmation of the deletion of the dddX gene from Psychrobacter sp.
D2. Lane M, DNA marker; Lane 1, Wild-type Psychrobacter sp. D2; Lane 2, the ΔdddX mutant. The ΔdddX mutant generated a 1000 bp PCR product using the dddX-1000-F/dddX-1000-R primer set, while the product length was 3247 bp for the wild-type strain.
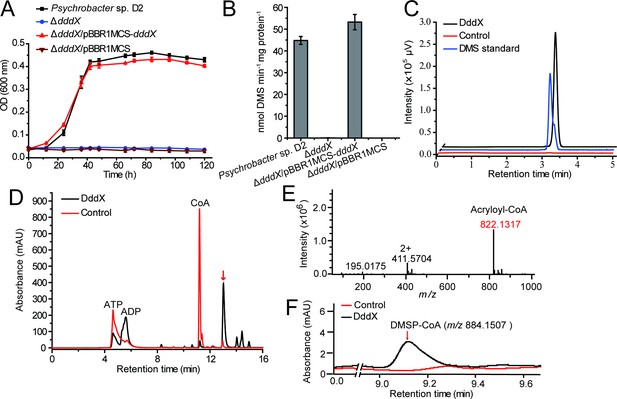
The function of Psychrobacter sp. D2 dddX in DMSP metabolism.
(A) Growth curves of the wild-type strain D2, the ΔdddX mutant, the complemented mutant (ΔdddX/pBBR1MCS-dddX), and the ΔdddX mutant complemented with an empty vector (ΔdddX/pBBR1MCS). All strains were grown with DMSP (5 mM) as the sole carbon source. The error bar represents standard deviation of triplicate experiments. (B), Detection of DMS production from DMSP degradation by the wild-type strain D2, the ΔdddX mutant, the complemented mutant ΔdddX/pBBR1MCS-dddX, and the mutant complimented with an empty vector ΔdddX/pBBR1MCS. The error bar represents standard deviation of triplicate experiments. (C), GC detection of DMS production from DMSP lysis catalyzed by the recombinant DddX. The reaction system without DddX was used as the control. DddX maintained a specific activity of ~8.0 μmol min–1 mg protein–1 at 20°C, pH 8.0.( D), HPLC analysis of the enzymatic activity of the recombinant DddX on DMSP at 260 nm. The peak of the unknown product is indicated with a red arrow. The reaction system without DddX was used as the control. (E), LC-MS analysis of the unknown product. (F), HPLC analysis of the intermediate of DddX catalysis at 260 nm. The HPLC system was coupled to a mass spectrometer for m/z determination. The reaction system without DddX was used as the control.
-
Figure 3—source data 1
Growth curves of the wild-type strain D2, the ΔdddX mutant, the complemented mutant (ΔdddX/pBBR1MCS-dddX), and the ΔdddX mutant complemented with an empty vector (ΔdddX/pBBR1MCS).
- https://cdn.elifesciences.org/articles/64045/elife-64045-fig3-data1-v2.xlsx
-
Figure 3—source data 2
Detection of DMS production from DMSP degradation by the wild-type strain D2, the ΔdddX mutant, the complemented mutant ΔdddX/pBBR1MCS-dddX, and the mutant complimented with an empty vector ΔdddX/pBBR1MCS.
- https://cdn.elifesciences.org/articles/64045/elife-64045-fig3-data2-v2.xlsx
-
Figure 3—source data 3
GC detection of DMS production from DMSP lysis catalyzed by the recombinant DddX.
- https://cdn.elifesciences.org/articles/64045/elife-64045-fig3-data3-v2.xlsx
-
Figure 3—source data 4
HPLC analysis of the enzymatic activity of the recombinant DddX on DMSP.
- https://cdn.elifesciences.org/articles/64045/elife-64045-fig3-data4-v2.xlsx
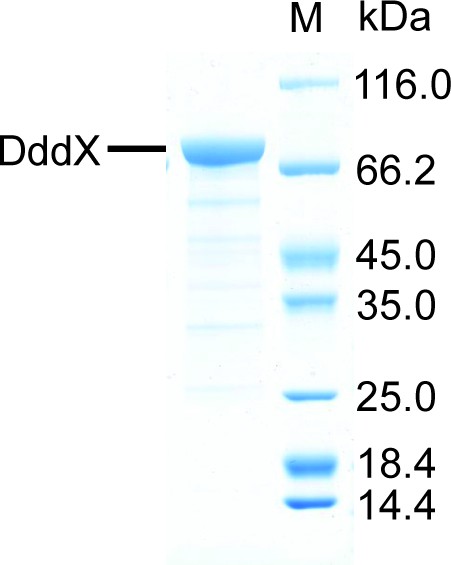
SDS-PAGE analysis of the recombinant DddX.
The predicted molecular mass of the recombinant DddX is 81.62 kDa using the compute MW tool (Gasteiger et al., 2005).
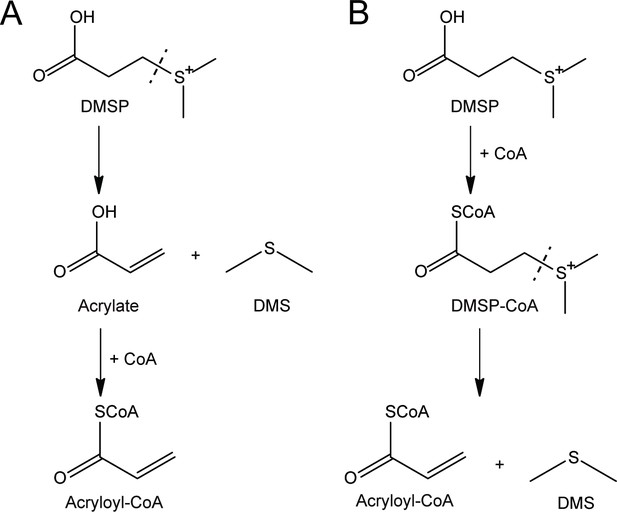
Two alternative mechanisms for DMSP degradation catalyzed by DddX.
(A), DMSP is primarily cleaved to DMS and acrylate. Subsequently, CoA is ligated to acrylate producing acryloyl-CoA. (B), CoA is primarily ligated to DMSP to produce DMSP-CoA, which is then cleaved to DMS and acryloyl-CoA.
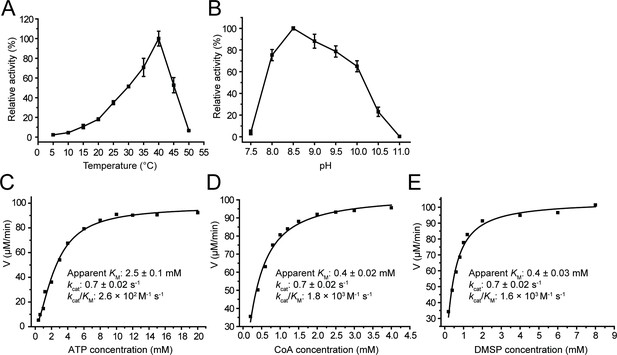
Characterization of recombinant DddX.
The error bar represents standard deviation of triplicate experiments. (A) Effect of temperature on DddX enzyme activity. (B) Effect of pH on DddX enzyme activity. (C) Kinetic parameters of DddX for ATP. (D) Kinetic parameters of DddX for CoA. (E) Kinetic parameters of DddX for DMSP.
-
Figure 3—figure supplement 3—source data 1
Characterization of recombinant DddX.
- https://cdn.elifesciences.org/articles/64045/elife-64045-fig10-data10-v2.xlsx
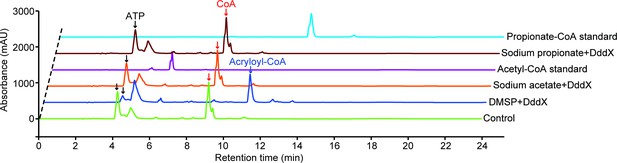
HPLC assay of the enzymatic activity of DddX toward DMSP, sodium acetate, and sodium propionate at 260 nm.
The peaks of ATP were indicated with black arrows, the peaks of CoA were indicated with red arrows, and the peak of acryloyl-CoA was indicated with the blue arrow. The reaction system without DddX was used as the control.
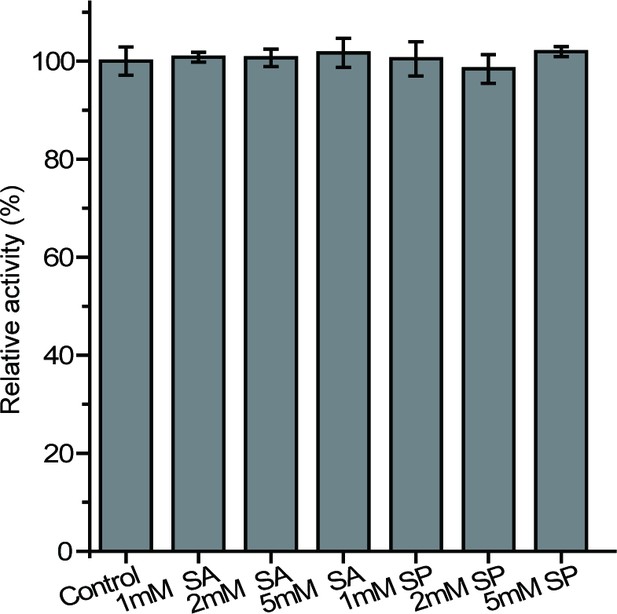
The effects of potential inhibitors on the enzymatic activity of DddX.
SA, sodium acetate; SP, sodium propionate. The activity of DddX with no inhibitor was used as a reference (100%).
-
Figure 3—figure supplement 5—source data 1
The effects of potential inhibitors on the enzymatic activity of DddX.
- https://cdn.elifesciences.org/articles/64045/elife-64045-fig11-data11-v2.xlsx
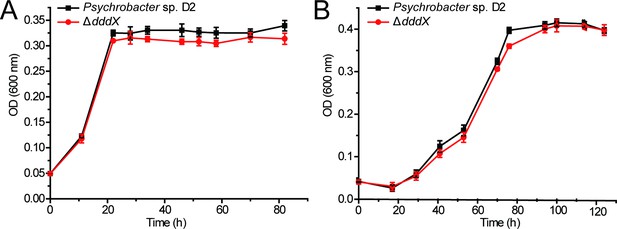
The growth curves of Psychrobacter sp.
D2 and the ΔdddX mutant on sodium acetate (A) or sodium propionate (B) as the sole carbon source (5 mM) at 25°C.
The error bar represents standard deviation of triplicate experiments.
-
Figure 3—figure supplement 6—source data 1
The growth curves of Psychrobacter sp.
D2 and the ΔdddX mutant on sodium acetate or sodium propionate as the sole carbon source.
- https://cdn.elifesciences.org/articles/64045/elife-64045-fig12-data12-v2.xlsx
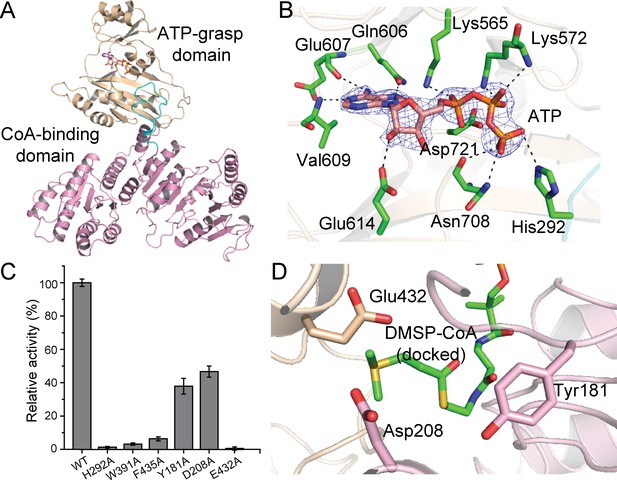
Structural and mutational analyses of DddX.
(A) The overall structure of the DddX monomer. The DddX molecule contains a CoA-binding domain (colored in pink) and an ATP-grasp domain (colored in wheat). The loop region from the CoA-binding domain inserting into the ATP-grasp domain is colored in cyan. The ATP molecule is shown as sticks. (B) Residues of DddX involved in binding ATP. The 2Fo - Fc densities for ATP are contoured in blue at 2.0σ. Residues of DddX involved in binding ATP are colored in green. (C) Enzymatic activities of DddX and its mutants. The activity of WT DddX was taken as 100%. (D) Structural analysis of the possible catalytic residues for the cleavage of DMSP-CoA. The docked DMSP-CoA molecule and the probable catalytic residues of DddX are shown as sticks.
-
Figure 4—source data 1
Enzymatic activities of DddX and its mutants.
- https://cdn.elifesciences.org/articles/64045/elife-64045-fig4-data1-v2.xls
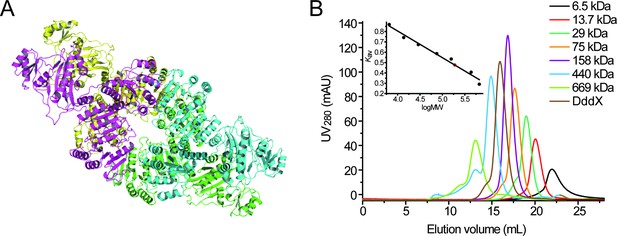
Structural and gel filtration analysis of DddX state of aggregation.
(A), The overall structure of DddX tetramer. Different monomers are displayed in different colors. (B), Gel filtration analysis of DddX. Inset, semilog plot of the molecular mass of all standards used versus their Kav values (black circles). The red spot indicates the position of the Kav value of DddX interpolated in the regression line. DddX monomer has a molecular mass of 81.62 kDa.
-
Figure 4—figure supplement 1—source data 1
Gel filtration analysis of DddX.
- https://cdn.elifesciences.org/articles/64045/elife-64045-fig14-data14-v2.xls
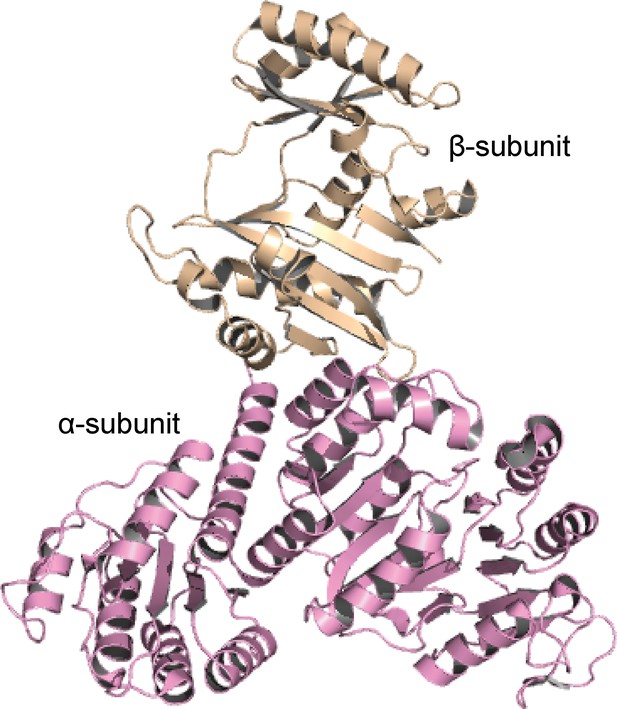
The overall structure of ACD1.
The α-subunit and the β-subunit of ACD1 (PDB code: 4xym) are colored in pink and wheat, respectively.

Sequence alignment of DddX homologs, acetyl-CoA synthetases (ACS), and ATP-citrate lyases (ACLY).
The conserved histidine residue is marked with a red star. The swinging loop of DddX (Gly280-Tyr300) is indicated, which corresponds to the swinging loop reported in acetyl-CoA synthetase ACD1 (Gly242-Val262) (Weiße et al., 2016).
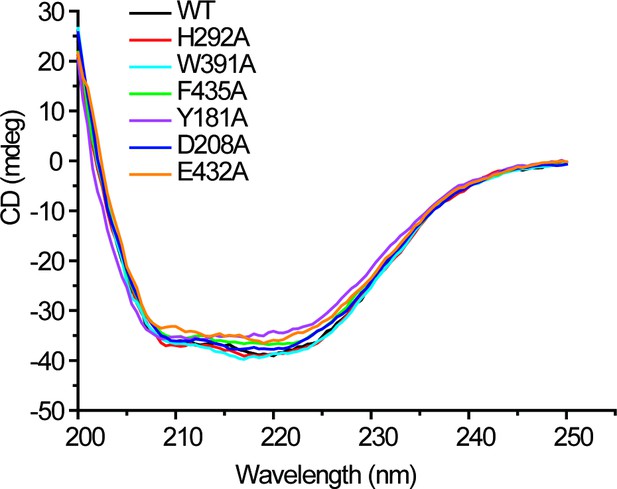
CD spectra of WT DddX and its mutants.
-
Figure 4—figure supplement 4—source data 1
CD spectra of WT DddX and its mutants.
- https://cdn.elifesciences.org/articles/64045/elife-64045-fig15-data15-v2.xls
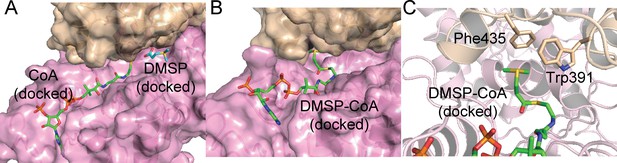
Structural analysis of DddX docked with DMSP and CoA, and DMSP-CoA.
(A). The structure of DddX docked with DMSP and CoA. DMSP and CoA molecules are shown as sticks. The surfaces of two DddX monomers are colored in wheat and pink, respectively. (B). The structure of DddX docked with DMSP-CoA. DMSP-CoA is shown as sticks. The surfaces of two DddX monomers are colored in wheat and pink, respectively. (C). Structural analysis of residues which form cation-π interactions with the sulfonium group of DMSP-CoA. DMSP-CoA and residues Trp391 and Phe435 are shown as sticks.
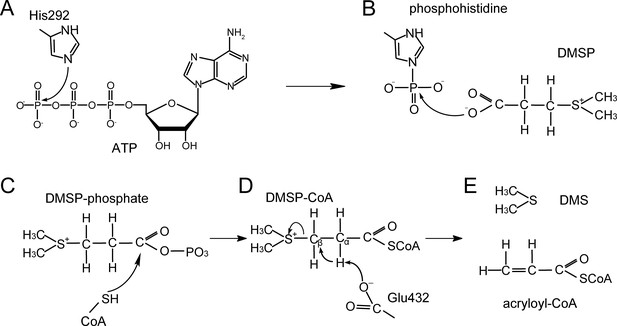
A proposed mechanism for DMSP cleavage to generate DMS and acryloyl-CoA catalyzed by DddX.
(A) The residue His292 attacks the γ-phosphate of ATP. (B), The phosphoryl group is transferred from phosphohistidine to the DMSP molecule. (C), DMSP-phosphate is attacked by CoA. (D), The residue Glu432 acts as a general base to attack DMSP-CoA. (E), DMS and acryloyl-CoA are generated.
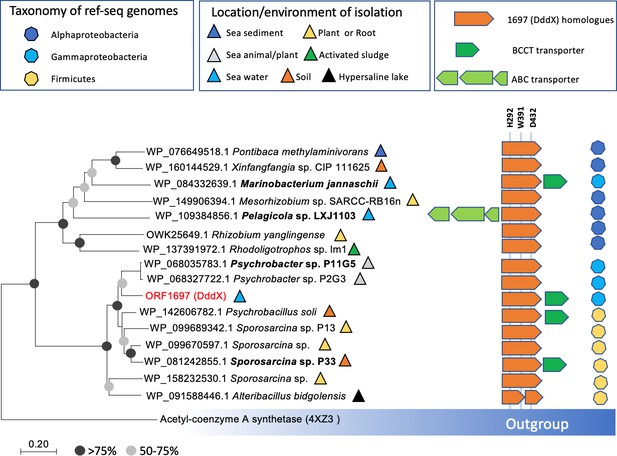
Distribution of DddX in bacterial genomes.
The phylogenetic tree was constructed using neighbor-joining method in MEGA7. The acetyl-coenzyme A synthetase (ACS) (Weiße et al., 2016) was used as the outgroup. Sequence alignment was inspected for the presence of the key histidine residue (His292) involved in histidine phosphorylation that is known to be important for enzyme activity. A conserved Tyr391 is also found which is involved in cation-pi interaction with DMSP. The BCCT-type or ABC-type transporters for betaine-carnitine-choline-DMSP were found in the neighborhood of DddX in several genomes. Those DddX homologs that are functionally characterized (Figure 6—figure supplement 1) are highlighted in bold.
Tables
Reagent type (species) or resource | Designation | Source or reference | Identifiers | Additional information |
---|---|---|---|---|
Strain, strain background (Psychrobacter sp.) | D2 | This study;Zhang Laboratory | Wild-type isolate; Available from Zhang lab | |
Strain, strain background (Psychrobacter sp.) | ΔdddX | This study;Zhang Laboratory | the dddX gene deletion mutant of Psychrobacter sp. D2; Available from Zhang lab | |
Strain, strain background (Psychrobacter sp.) | ΔdddX/pBBR1MCS-dddX | This study;Zhang Laboratory | ΔdddX containing pBBR1MCS-dddX plasmid; Available from Zhang lab | |
Strain, strain background (Psychrobacter sp.) | ΔdddX/pBBR1MCS | This study;Zhang Laboratory | ΔdddX containing pBBR1MCS plasmid; Available from Zhang lab | |
Strain, strain background (Escherichia coli) | WM3064 | Dehio and Meyer, 1997 | Conjugation donor strain | |
Strain, strain background (Escherichia coli) | DH5α | Vazyme Biotech company (China) | Transformed cells for gene cloning | |
Strain, strain background (Escherichia coli) | BL21(DE3) | Vazyme Biotech company (China) | Transformed cells for gene expression | |
Recombinant DNA reagent | pK18mobsacB-Ery | Wang et al., 2015b | Gene knockout vector | |
Recombinant DNA reagent | pK18Ery-dddX | This study;Zhang Laboratory | pK18mobsacB-Ery containing the homologous arms of the dddX gene of Psychrobacter. sp. D2; Available from Zhang lab | |
Recombinant DNA reagent | pBBR1MCS | Kovach et al., 1995 | Broad-host-range cloning vector | |
Recombinant DNA reagent | pBBR1MCS-dddX | This study;Zhang Laboratory | pBBR1MCS containing the dddX gene and its promoter of Psychrobacter. sp. D2; Available from Zhang lab | |
Recombinant DNA reagent | pET-22b-dddX | This study;Zhang Laboratory | Used for dddX expression; Available from Zhang lab | |
Commercial assay or kit | Pierce BCA Protein Assay Kit | Thermo, USA | Protein assay | |
Commercial assay or kit | Bacterial genomic DNA isolation kit | BioTeke Corporation, China | DNA extraction | |
Commercial assay or kit | RNeasy Mini Kit | QIAGEN, America | RNA extraction | |
Commercial assay or kit | PrimeScript RT reagent Kit | Takara, Japan | Reverse transcription | |
Commercial assay or kit | Genome sequencing of Psychrobacter sp. D2 | Biozeron Biotechnology Co., Ltd, China | NCBI: JACDXZ000000000 | |
Commercial assay or kit | Transcriptome sequencing of Psychrobacter sp. D2 | BGI Tech Solutions Co., Ltd, China | NCBI: PRJNA646786 | |
Software, algorithm | HKL3000 program | Minor et al., 2006 | Diffraction data analysis | |
Software, algorithm | CCP4 program Phaser | Winn et al., 2011 | Diffraction data analysis | |
Software, algorithm | Coot | Emsley et al., 2010 | Diffraction data analysis | |
Software, algorithm | Phenix | Adams et al., 2010 | Diffraction data analysis | |
Software, algorithm | PyMOL | Schrödinger, LLC | http://www.pymol.org/ | |
Software, algorithm | MEGA 7 | Kumar et al., 2016 | Phylogenetic analysis |
Additional files
-
Supplementary file 1
Tables listing sampling information, homology alignment results, kinetic parameters, crystallographic data, bacterial strains, plasmids, medium composition, and primers used in this study.
(a) Information of the Antarctic samples used in this study. (b) Homology alignment of proteins in Psychrobacter sp. D2 with known DMSP lyases. (c) Kinetic parameters of DMSP lyases and DMSP demethylase DmdA. (d) Crystallographic data collection and refinement parameters of DddX. (e) Homology alignment of proteins in Psychrobacter sp. D2 with known enzymes involved in acrylate catabolism. (f) Strains and plasmids used in this study. (g) Composition of the basal medium (lacking the carbon source). (h) Primers used in this study.
- https://cdn.elifesciences.org/articles/64045/elife-64045-supp1-v2.docx
-
Transparent reporting form
- https://cdn.elifesciences.org/articles/64045/elife-64045-transrepform1-v2.docx