Endoglycan plays a role in axon guidance by modulating cell adhesion
Figures
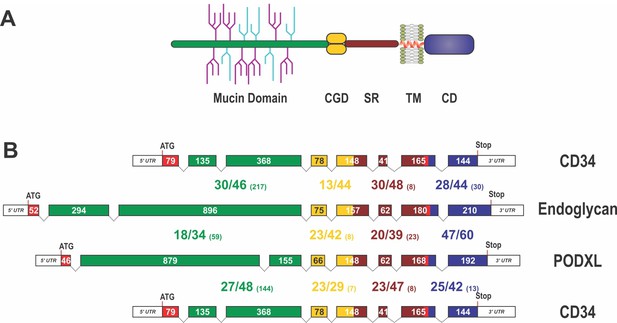
Domain organization, exon alignment, and conservation of the CD34 family of sialomucins.
(A) Schematic drawing of the domain organization of CD34 family members. They all contain an N-terminal, highly glycosylated, mucin domain (green), a cysteine-containing globular domain (CGD, yellow), a juxtamembrane stalk region (SR, brown), as well as a transmembrane alpha-helix (TM, red) and a cytoplasmic domain (CD, blue). O-linked glycosylation sites within the mucin domain are depicted in light blue, whereas further sialylated residues are symbolized in purple. N-linked glycosylation sites are not shown. Note, that the indicated glycosylation sites in this scheme are only symbolizing the extensive amount of glycosylation in sialomucins and are not representing the actual position of glycosylation. (B) Exon organization and domain conservation of sialomucins. Transcripts of CD34 family members are encoded by eight separate exons (colored boxes). While the length of exons coding for the cysteine containing globular domain (yellow), the juxtamembrane stalk region (brown), and the cytoplasmic domain (blue) are more or less conserved (exon sizes are given within the boxes), exons coding for the mucin domain (green) vary markedly in their length and organization. The translational start sites are highlighted by the ATG and the end of the coding sequences are indicated by the given Stop codon. Protein homology between the different chicken sialomucins is depicted by the large numbers between the exon pictograms. All domains were compared separately and the colors used indicate the corresponding domains. The first number indicates identical amino acids between the compared proteins, the second number represents conserved residues and the number in brackets designates the amount of gap positions within the alignment of the domains (e.g. 28/44 (30)). The alignment was done using MUSCLE version 3.7 configured to the highest accuracy (Edgar, 2004). Single gap positions were scored with high penalties, whereas extensions of calculated gaps were less stringent. Using such parameters homologous regions of only distantly related sequences can be identified. Note, that within the mucin domain only some blocks, interspaced by sometimes large gap regions, are conserved between the different proteins.
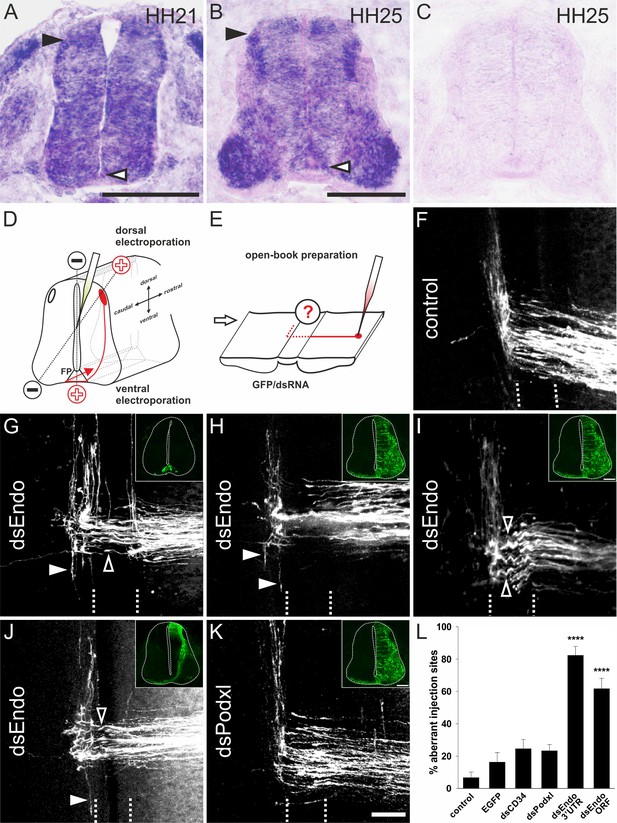
Endoglycan is required for correct turning of post-crossing commissural axons.
(A,B) Endoglycan is expressed in the developing neural tube during commissural axon guidance. Endoglycan is expressed throughout the neural tube at HH21, including the floor plate (white arrowhead) (A). (B) At HH25, Endoglycan is still found in most cells of the spinal cord. High levels are found in motoneurons and interneurons, including the dorsal dI1 neurons (black arrowhead), and in the floor plate (white arrowhead). No staining was found when hybridization was carried out with a sense probe (C). Commissural axon pathfinding was analyzed in ‘open-book’ preparations (D,E; see Materials and methods for details). The positions of the electrodes for dorsal and ventral electroporation are indicated (D). In control embryos at HH26, commissural axons have crossed the floor plate and turned rostrally along the contralateral floor-plate border (F). In contrast, after downregulation of Endoglycan (G–J) commissural axons failed to turn along the contralateral floor-plate border or they turned randomly either rostrally or caudally (arrowheads in G-J). Occasionally, axons were turning already inside the floor plate (open arrowhead in G). A closer look at the morphology of the axons in the floor plate revealed their tortuous, ‘corkscrew'-like trajectory across the midline at many DiI injection sites (open arrowheads in I). To knockdown Endoglycan either in the floor plate or in commissural neurons, the ventral or dorsal spinal cord was targeted as indicated in (D) (see inserts in G and J, respectively). Phenotypes were the same as those observed after targeting one half of the spinal cord including the floor plate (H,I). Pathfinding was normal in embryos electroporated with dsRNA derived from Podocalyxin (K). The quantification of injection sites with pathfinding errors after targeting the floor plate or one half of the spinal cord is shown in (L). Pathfinding errors were seen only at 6.7±3.4% of the injection sites in untreated control embryos (n=10 embryos, 45 injection sites). In control embryos injected and electroporated with the EGFP plasmid alone, pathfinding errors were found at 16.2±6% of the injection sites (n=17 embryos, 92 injection sites). Injection and electroporation of dsRNA derived from CD34 (24.6±5.8%, n=8 embryos, 80 injection sites) and Podocalyxin (23.3±3.9%, n=17 embryos, 147 injection sites) did not affect midline crossing and turning behavior of commissural axons. By contrast, 82.3±5.6% (n=11 embryos, 65 sites) and 61.7±6.4% (n=18, 161 sites) of the injection sites in embryos injected with dsRNA derived from the 3’-UTR or the ORF of Endoglycan, respectively, showed aberrant pathfinding of commissural axons. One-way ANOVA with Tukey’s multiple comparisons test. P values ****<0.0001, compared to EGFP-injected control groups. The two groups electroporated with dsRNA derived from Endoglycan were not different from each other. Values represent average percentage of DiI injection sites per embryo with aberrant axonal navigation ± standard error of the mean. Source data and statistics are available in the Figure 2—source data 1 spreadsheet. Bar: 50 μm.
-
Figure 2—source data 1
Raw data and statistics for Figure 2L.
- https://cdn.elifesciences.org/articles/64767/elife-64767-fig2-data1-v2.xlsx
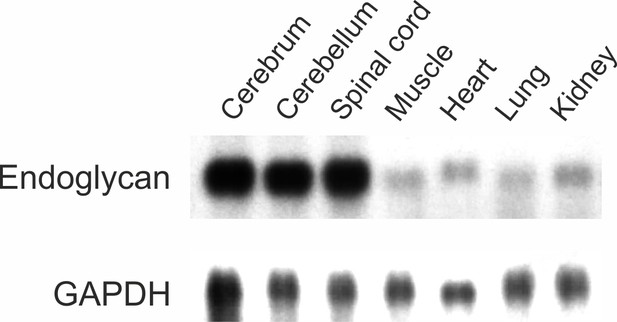
Endoglycan is mainly expressed in the developing nervous system.
Endoglycan is expressed only at low levels in non-neuronal tissues. Northern blot analysis of tissues taken from HH38 chicken embryos revealed its high expression levels in the cerebrum (brain without cerebellum), the cerebellum, and the spinal cord. Only low levels were found in muscle, heart, lung, and kidney. GAPDH was used as a loading control.
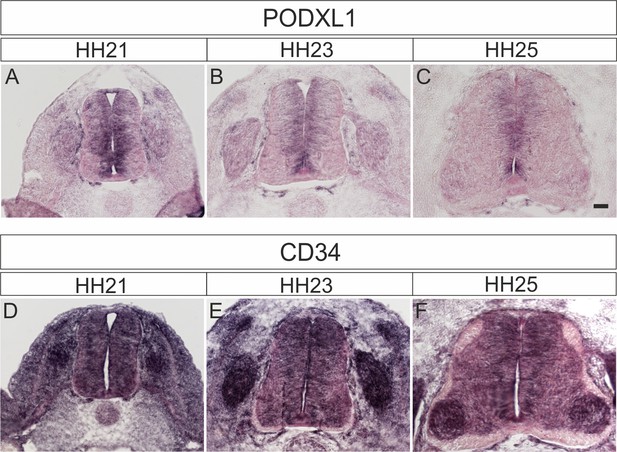
Podocalyxin and CD34 are expressed in the developing spinal cord during midline crossing of dI1 commissural axons.
Podocalyxin1 expression was mainly found in the ventricular zone (A–C). At HH21, expression was found in precursors of dorsal interneurons (A). Expression was also seen in the dorsal root ganglia (DRG). Expression of CD34 (D–F) was more ubiquitous with highest levels in DRG and increasing levels in motoneurons at HH25 (F).
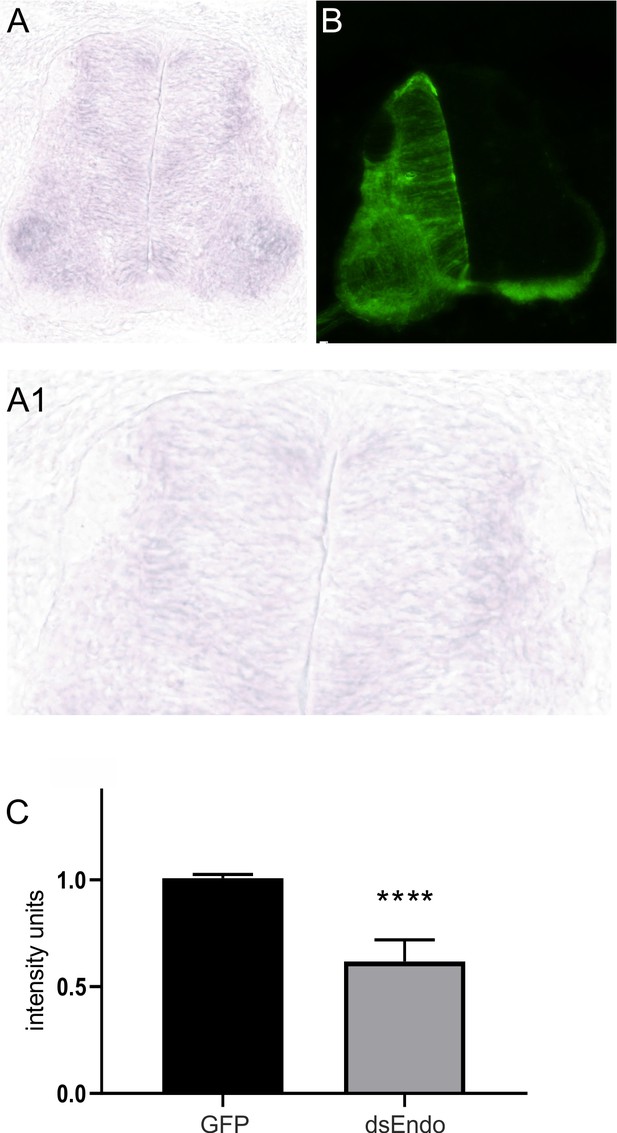
Electroporation of dsRNA derived from Endoglycan effectively downregulates Endoglycan mRNA.
In the absence of antibodies detecting Endoglycan, we had to use in situ hybridization to quantify the efficiency of Endoglycan downregulation (A). When we compared expression levels of Endoglycan between the electroporated and the non-electroporated side of a HH25 spinal cord, we found reduced signal intensities on the electroporated side. (B) Co-electroporation of a plasmid encoding GFP together with dsEndo allows for the identification of the electroporated side. On average, electroporation of dsEndo reduced mRNA levels by 39%. Keep in mind that only about 50% of the cells in the electroporated area are taking up the dsRNA. Therefore, the reduction in Endoglycan is very efficient in the transfected cells. Three sections per embryo and three embryos per group were included in the analysis. Source data and statistics are available in Figure 2—figure supplement 3—source data 1 spreadsheet.
-
Figure 2—figure supplement 3—source data 1
Raw data demonstrating efficient downregulation of Endoglycan.
- https://cdn.elifesciences.org/articles/64767/elife-64767-fig2-figsupp3-data1-v2.xlsx
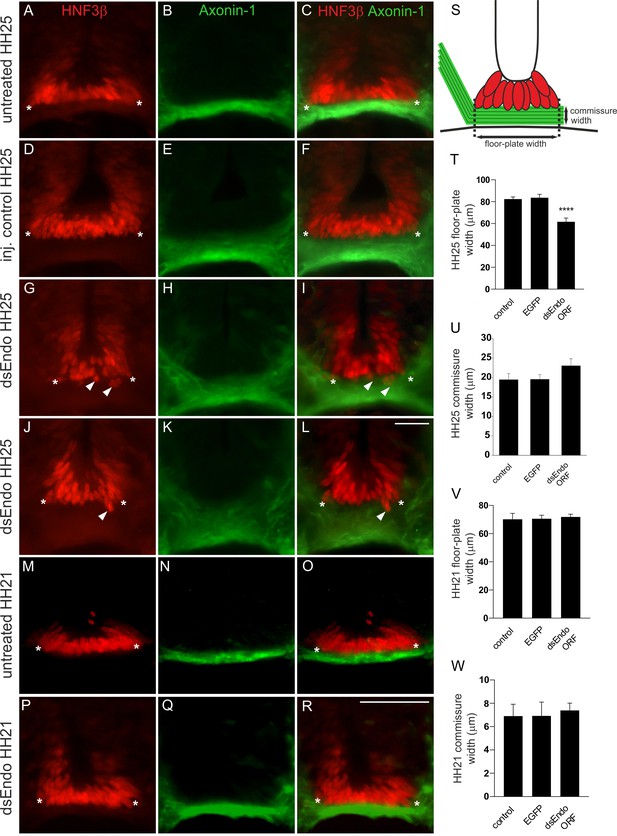
After downregulation of Endoglycan in one half of the spinal cord, the floor-plate morphology is compromised only after axonal midline crossing.
In untreated (A-C) and control-treated embryos (D-F), the floor plate is of triangular shape with floor-plate cells precisely aligned at the ventral border. There is no overlap between the floor plate (visualized by HNF3β staining; red) and the commissure (visualized by anti-Axonin1 staining; green). The shape of the floor plate is no longer triangular in embryos lacking Endoglycan (G-L). The floor-plate cells are not aligned ventrally (arrowheads in G, I, J, and L) and the floor plate appears to have gaps. This change in morphology is only seen at HH25, when midline crossing is completed. When the floor-plate morphology was analyzed at HH21, there was no difference between control (M-O) and experimental embryos electroporated with dsRNA derived from Endoglycan (P-R). Note that some more ventral commissural axon populations have crossed the floor plate at this stage. But overall, the number of axons that form the commissure at HH21 is still very small. The width of the floor plate (indicated by asterisks) was measured (S,T). There was no significant difference in spinal cord width at HH25 (400.2 ± 54.5 µm in untreated controls, 438.2 ± 30.3 µm in EGFP-expressing controls, and 394 ± 12.0 µm in dsEndo embryos), but floor plates were significantly narrower in embryos lacking Endoglycan (T; 61.6 ± 2.9 µm; n = 7 embryos; p<0.001) compared to untreated (82.4 ± 1.8 µm; n = 6 embryos) and EGFP-injected control embryos (83.6 ± 2.6 µm; n = 6 embryos). One-way ANOVA with Tukey’s multiple comparisons test. The commissure had a tendency to be wider in experimental compared to control embryos but the effect was not statistically significant (U). The width of the floor plate was not different between groups when measured at HH21 (V) with 70.1 ± 4.2 µm (n = 3) for untreated and 70.6 ± 2.5 µm for EGFP controls (n = 4), compared to 71.8 ± 2.0 µm for experimental embryos (n = 3). No difference was seen in the width of the commissure. Two-tailed T-test. ****p<0.0001. Mean ± SEM are given. Electroporation was targeted to one side of the spinal cord as shown in the insert in Figure 2H. Bar: 50 µm. Source data and statistics are available in Figure 3—source data 1 spreadsheet.
-
Figure 3—source data 1
Raw data and statistics for Figure 3.
- https://cdn.elifesciences.org/articles/64767/elife-64767-fig3-data1-v2.xlsx
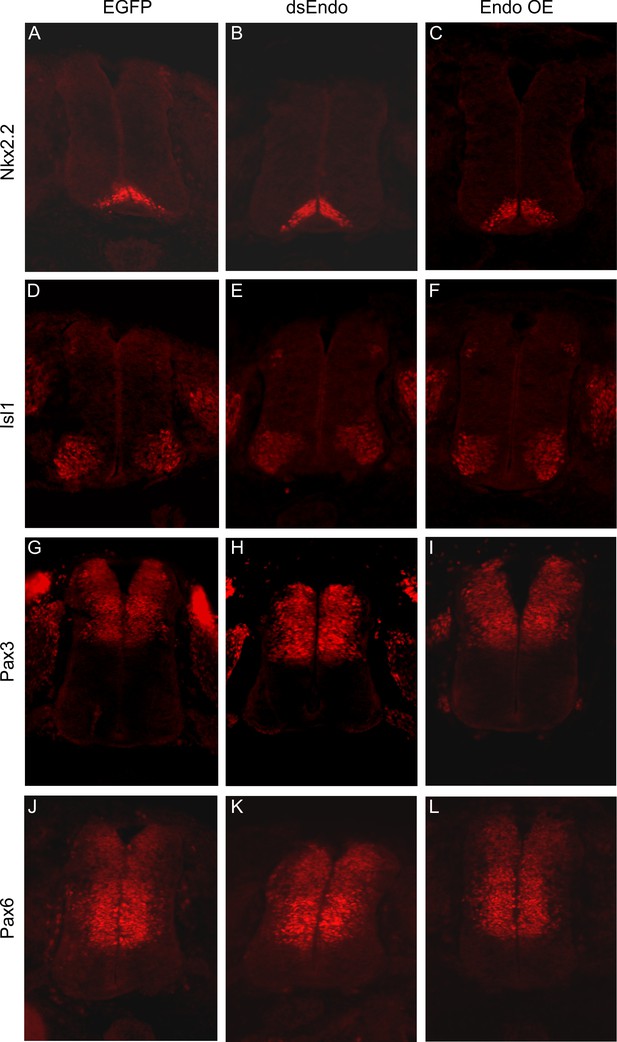
Downregulation or overexpression of Endoglycan does not affect spinal cord patterning.
To check whether the effect of Endoglycan perturbation on axonal pathfinding was indirect due to changes in spinal cord patterning, we used a series of antibodies to stain sections taken from control embryos expressing GFP (A,D,G,J), embryos electroporated with dsEndo (B,E,H,K), or embryos overexpressing Endoglycan (C,F,I,L). We found no evidence for aberrant patterning, when we compared sections stained with Nkx2.2 (A–C), Islet1 (D–F), Pax3 (G–I), or Pax6 (J–L).
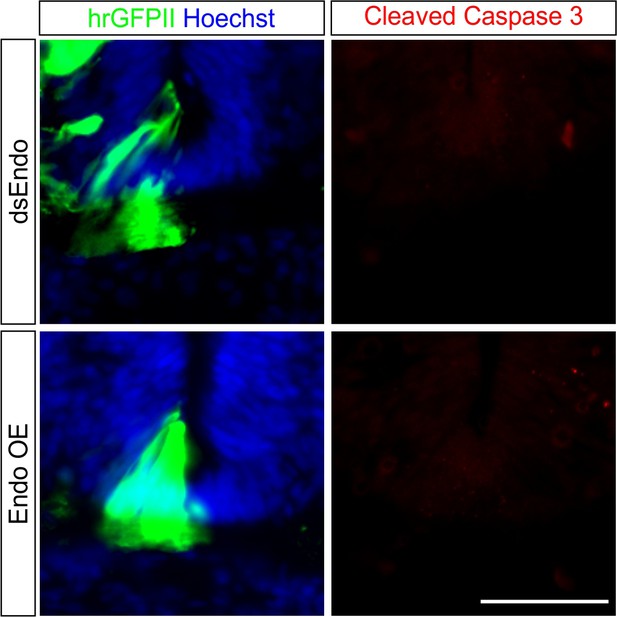
Experimental manipulation of Endoglycan levels does not induce cell death in the floor plate.
To exclude that changes in floor plate morphology were caused by apoptosis as a consequence of lowering levels of Endoglycan by the electroporation of dsEndo (upper row) or increasing levels of Endoglycan by expressing a plasmid encoding chicken Endoglycan (lower row), we stained sections taken from HH25 embryos with antibodies against cleaved caspase-3. We did not see any staining in either of the experimental groups nor in untreated control embryos (not shown). Therefore, apoptosis can be ruled out as an explanation for the changes in floor-plate morphology. Bar: 50 µm.
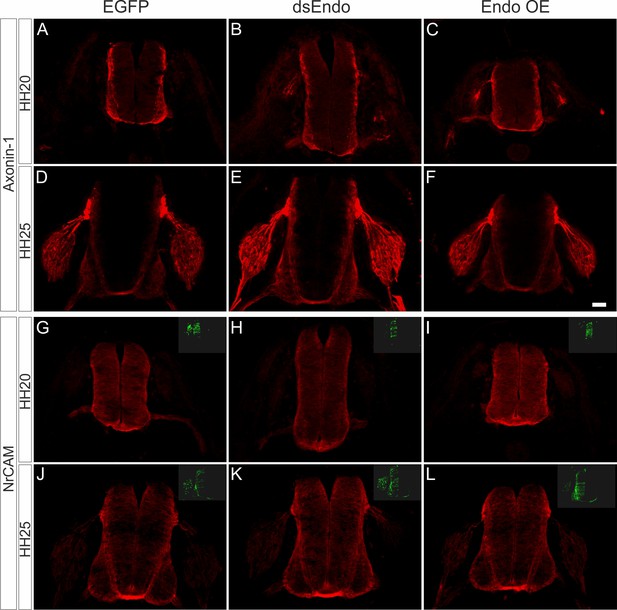
The errors in commissural axon pathfinding seen after perturbation of Endoglycan levels are not due to changes in the expression of known guidance cues for dI1 axons.
To exclude that the changes in axonal pathfinding seen after silencing or overexpression of Endoglycan were explained by an effect on the expression of known axon guidance cues for dI1 axons, Axonin-1/Contactin-2 (A–F) or NrCAM (G–L), we compared sections taken from control embryos electroporated with a plasmid encoding EGFP (A,D,G,J), embryos electroporated with dsEndo (B,E,H,K), or embryos overexpressing Endoglycan (C,F,I,L). We found no differences in expression of Axonin-1 and NrCAM. We compared sections taken from embryos sacrificed at HH20 (A–C, G–I) and HH25 (D–F, J–L). Bar: 50 µm.
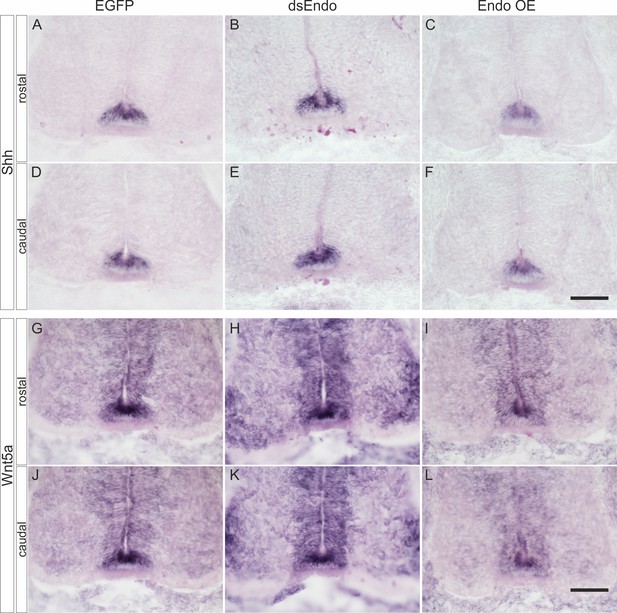
Perturbation of Endoglycan expression does not affect guidance of post-crossing commissural axons indirectly by changing Shh or Wnt5a expression.
We did not find any changes in the expression of Shh (A–F) or Wnt5a (G–L) compared to control embryos expressing EGFP (A,D,G,J) after silencing Endoglycan (B,E,H,K) or after overexpression of Endoglycan (C,F,I,L). Shh was found at higher levels in the caudal compared to the rostral floor plate (A–F), as reported previously (Bourikas et al., 2005). Wnt5a levels did not differ between rostral and caudal sections taken from the lumbar part of the spinal cord, as reported earlier (Domanitskaya et al., 2010).
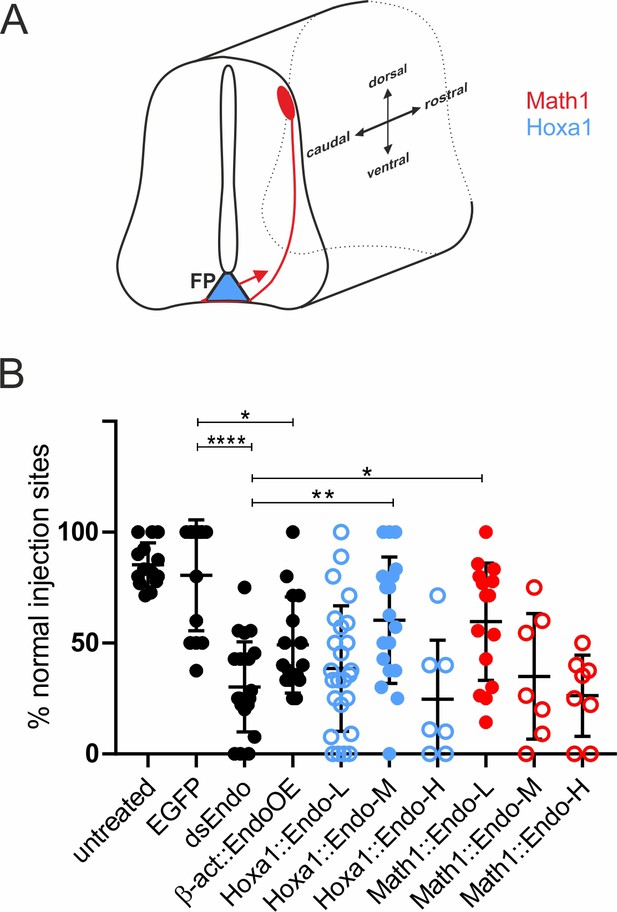
Too much or too little Endoglycan causes aberrant axon guidance.
Because silencing Endoglycan either in commissural neurons or in the floor plate caused the same type of axon guidance defects, we wanted to test the idea that the presence of an adequate amount, but not the source of Endoglycan was important. We therefore downregulated Endoglycan by transfection of dsRNA derived from the 3’UTR of Endoglycan into one half of the spinal cord. We then tried to rescue the aberrant axon guidance phenotype by co-electroporation of the Endoglycan ORF specifically in dI1 neurons (using the Math1 enhancer, red) or in the floor plate (using the Hoxa1 enhancer; blue, A and B). The rescue constructs were used at a concentration of 150 (L = low), 300 (M = medium), and 750 (H = high) ng/µl, respectively. In both cases, rescue was only possible with one concentration: the medium concentration of the Endoglycan plasmid driven by the Hoxa1 promoter and the low concentration of the plasmid driven by the Math1 promoter. The lowest concentration of the Hoxa1-driven construct and the two higher concentrations of the Math1-driven constructs were not able to rescue the aberrant phenotype. Note that the amounts of Endoglycan cannot be compared between the Math1- and the Hoxa1 enhancers, as they differ in their potency to drive expression. However, we can conclude a response in a dose-dependent manner in both cases. Statistical analysis by one-way ANOVA: *p<0.05, **p<0.01, ***p<0.001. Values are shown ± standard deviation. See Table 1 for values. Source data and statistics are available in Figure 4—source data 1 spreadsheet.
-
Figure 4—source data 1
Raw data and statistics for rescue experiments.
- https://cdn.elifesciences.org/articles/64767/elife-64767-fig4-data1-v2.xlsx
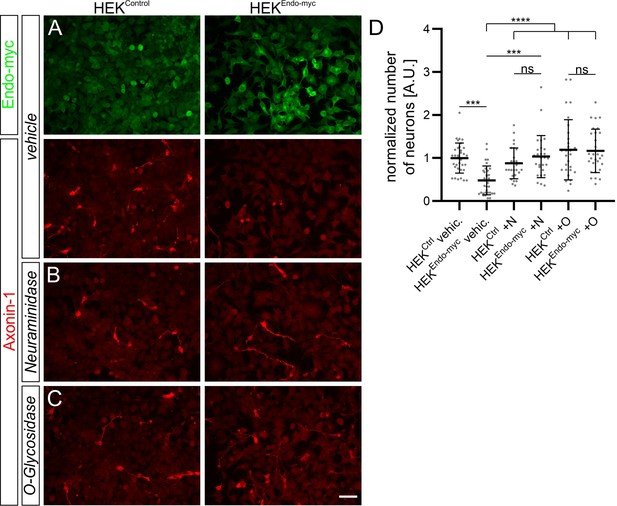
Endoglycan expression reduces adhesion of commissural neurons in vitro.
(A-C) Commissural neurons dissected from HH25/26 chicken embryos were cultured on a layer of control HEK cells or HEK cells stably expressing human Endoglycan. Neurons were allowed to attach for 16 hr. Staining for Axonin-1 revealed a pronounced decrease in the number of commissural neurons on HEK cells expressing Endoglycan compared to control HEK cells (A and D). For each replicate, the number of neurons attached to HEK cells expressing Endoglycan was normalized to the number of cells attached to control HEK cells (D). The number of commissural neurons attached to control HEK cells was more than twice the number on Endoglycan-expressing HEK cells (only 0.47 ± 0.34 compared to 1 ± 0.35). Treatment with Neuraminidase (+N) or O-glycosidase (+O) abolished the difference. See Table 2 for values. N(replicates)=4. ns (not significant), ***p<0.001, ****p<0.0001, one-way ANOVA with Tukey’s multiple comparisons test. Error bars represent standard deviations. O, O-glycosidase; N, Neuraminidase; vehic, vehicle; ctrl, control. Scale bar: 50 µm. Source data and statistics are available in Figure 5—source data 1 spreadsheet.
-
Figure 5—source data 1
Commissural neuron counts on HEK cells expressing Endoglycan.
- https://cdn.elifesciences.org/articles/64767/elife-64767-fig5-data1-v2.xlsx
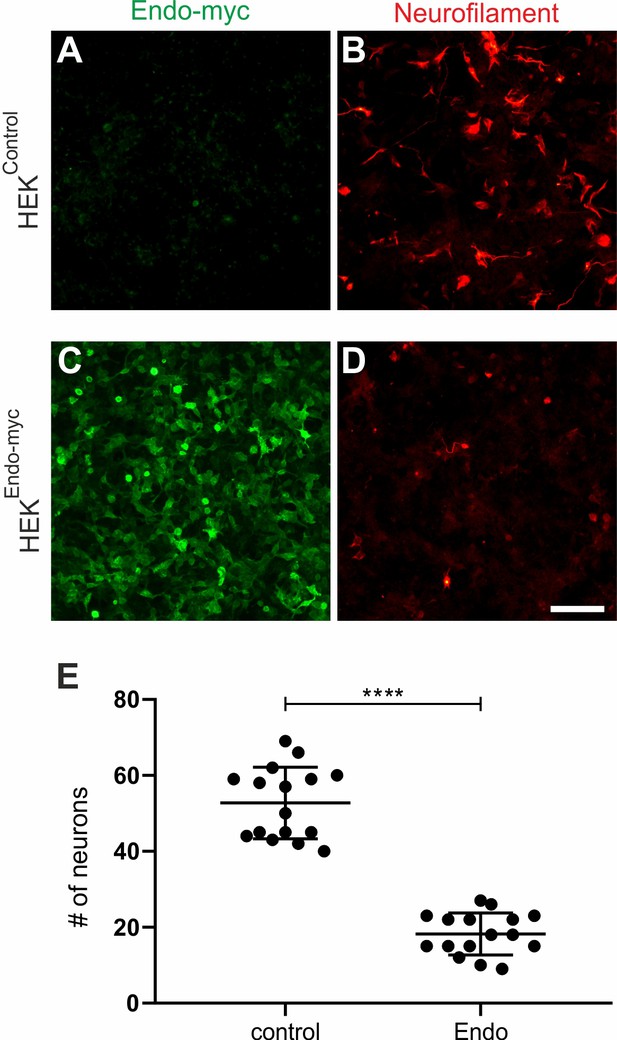
Endoglycan expression reduces adhesion of motor neurons in vitro.
Dissociated motoneurons dissected from HH26 chicken embryos were cultured on control HEK cells (A) or HEK cells stably expressing human Endoglycan (C). Neurons were allowed to attach for 40 hr. Staining for Neurofilament revealed a pronounced decrease in the number of motoneurons on HEK cells expressing Endoglycan (D) compared to control HEK cells (B). On control HEK cells (A), we found 52.8 ± 2.4 motoneurons per view field. On a layer of HEK cells expressing Endoglycan, only 18.3 ± 1.4 motoneurons were counted. Similar results were obtained in five independent experiments. Bar: 100 μm. ****p<0.0001, unpaired t test, two-tailed. Source data and statistics are available in Figure 5—figure supplement 1—source data 1 spreadsheet.
-
Figure 5—figure supplement 1—source data 1
Motoneuron counts on HEK cells expressing Endoglycan.
- https://cdn.elifesciences.org/articles/64767/elife-64767-fig5-figsupp1-data1-v2.xlsx
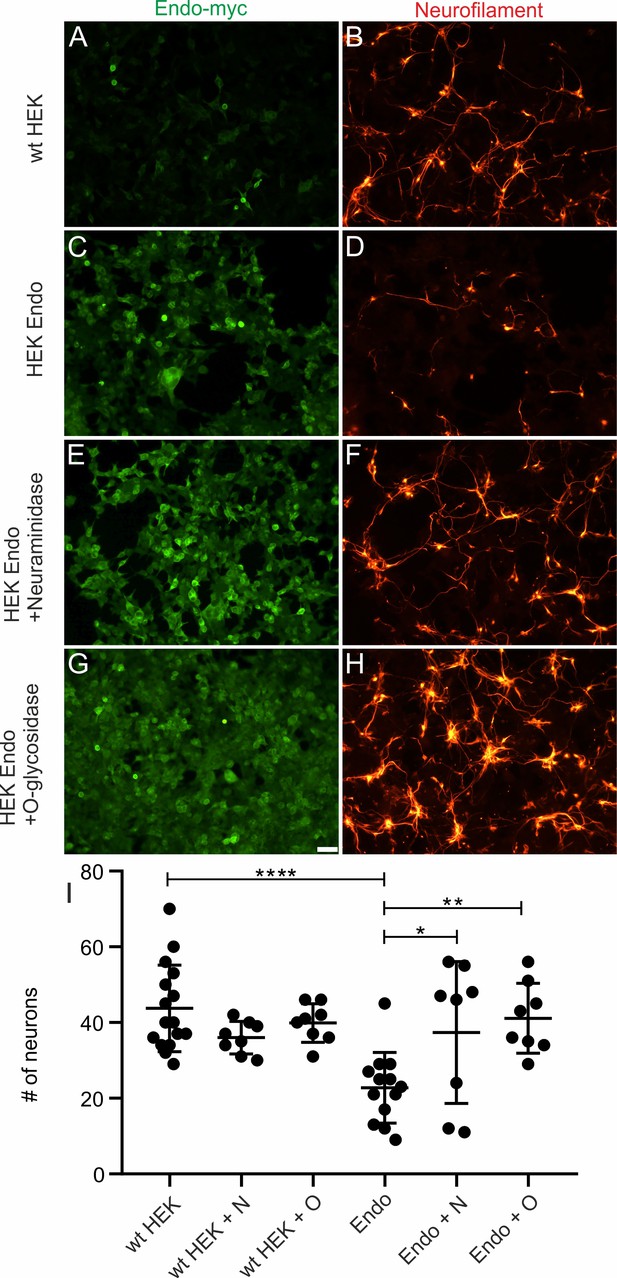
Post-translational modification of Endoglycan is required for its anti-adhesive effects on motoneurons.
Motoneurons (stained with anti-neurofilament antibodies in B,D,F,H) were cultured on control HEK cells (A–B) or Endoglycan-expressing HEK cells (C–H). Before adding the motoneurons, HEK cells were either left untreated (A–D), or treated with neuraminidase (E,F) or O-glycosidase (G,H) for 2 hr. Attached motoneurons were counted after 40 hr. Removal of sialic acid by neuraminidase (E,F) or O-glycosidase (G,H) abolished the anti-adhesive effect of Endoglycan (C,D). Quantification of the number of attached motoneurons under the different conditions is shown in (I). ns p>0.05, *p<0.05, **p<0.01, ****p<0.0001. One-way ANOVA with Tukey’s multiple comparisons test. Source data and statistics are available in Figure 5—figure supplement 2—source data 1 spreadsheet.
-
Figure 5—figure supplement 2—source data 1
Motoneuron counts on HEK cells expressing Endoglycan treated with Neuraminidase and O-Glycosidase.
- https://cdn.elifesciences.org/articles/64767/elife-64767-fig5-figsupp2-data1-v2.xlsx
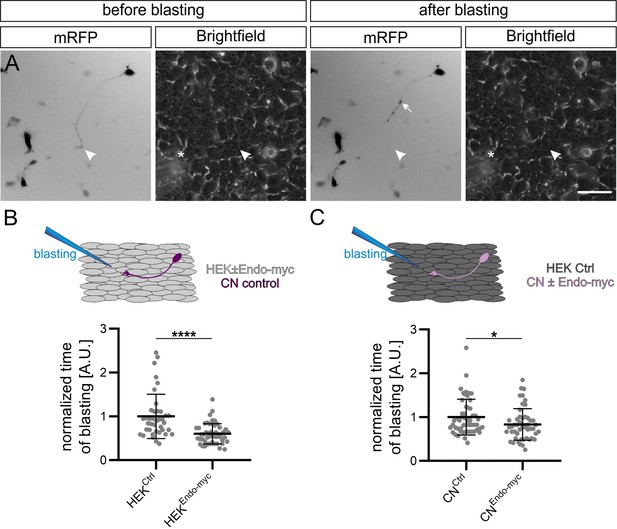
Endoglycan reduces the adhesive strength of commissural neuron growth cones.
(A) Example of snapshots taken before and after growth cone blasting of mRFP-positive commissural neurons cultured on a HEK cell layer (shown in the brightfield channel). White arrowheads and asterisks show the location of the growth cone and the approximate location of the micropipette tip, respectively. (B) Control mRFP-transfected commissural neurons were plated either on a layer of control HEK cells or HEK cells expressing Endoglycan. The time it took to detach a growth cone from the HEK cells was taken as a measure for the adhesive strength. The adhesion of growth cones to HEK cells expressing Endoglycan was significantly reduced (0.59 ± 0.26 compared to 1 ± 0.61 on control HEK cells). N(replicates)=3, n(growth cones)=40 (HEKCtrl) and 50 (HEKEndo-myc). (C) Similar observations were made when commissural neurons were transfected with Endoglycan instead of the HEK cells. Detachment was faster for growth cones of mRFP-transfected neurons co-transfected with Endoglycan and plated on control HEK cells (0.83 ± 0.33) compared to control neurons transfected only with mRFP (1 ± 0.41). N(replicates)=6, n(growth cones)=52 (CNCtrl) and 51 (CNEndo-myc). *p<0.05, ****p<0.0001, Two tailed Mann-Whitney test. Error bars represent standard deviation. CN, commissural neuron; Ctrl, control. Scale bar: 50 μm. Source data and statistics are available in Figure 6—source data 1 spreadsheet.
-
Figure 6—source data 1
Raw data of growth cone blasting experiments.
- https://cdn.elifesciences.org/articles/64767/elife-64767-fig6-data1-v2.xlsx
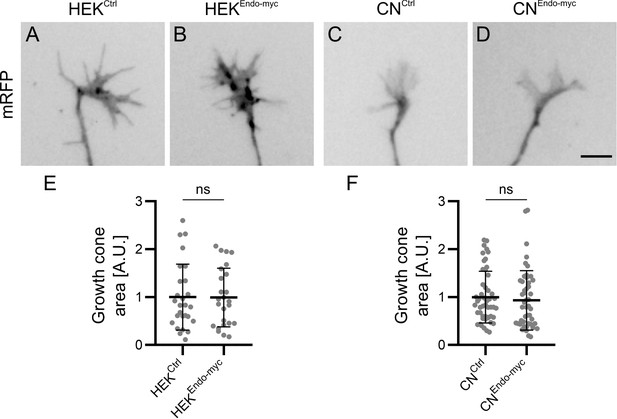
Reduced attachment of growth cones in the presence of higher levels of Endoglycan cannot be explained by changes in growth cone area.
To exclude that the observed differences in adhesive strength between growth cones and HEK cells were influenced by growth cone size, we measured growth cone areas by tracing the edge of the mRFP-positive growth cones from images taken before blasting with a x20 objective. Note that the numbers of growth cones in the growth cone blasting experiment (Figure 6) and the area measurements shown here differ because in two replicates in ~50% of the measured growth cones only videos were taken but no still images. Therefore, these growth cones are not included in the size measurement. The area of each growth cone was measured in imageJ using the tracing tool and the value was normalized to the average growth cone area of the control condition (either HEKCtrl or CNCtrl) for each replicate. A.U., arbitrary unit. Values were 1 ± 0.69 for HEKCtrl (A) versus 0.99 ± 0.61 for HEKEndo-myc (B), and 1 ± 0.54 for CNCtrl (C) versus 0.93 ± 0.62 for CNEndo-myc (D; mean ± standard deviation). Statistical analysis: E, p=0.9694 (ns, unpaired T-test), N (replicates) = 3; n (growth cones)=27 (HEKCtrl) and 25 (HEKEndo-myc); F, p=0.3261 (ns, Mann Whitney test), N (replicates) = 6; n (growth cones)=50 (CNCtrl) and 49 (CNEndo-myc). Bar: 10 µm. Source data and statistics are available in Figure 7—source data 1 spreadsheet.
-
Figure 7—source data 1
Raw data of growth cone area measurements.
- https://cdn.elifesciences.org/articles/64767/elife-64767-fig7-data1-v2.xlsx
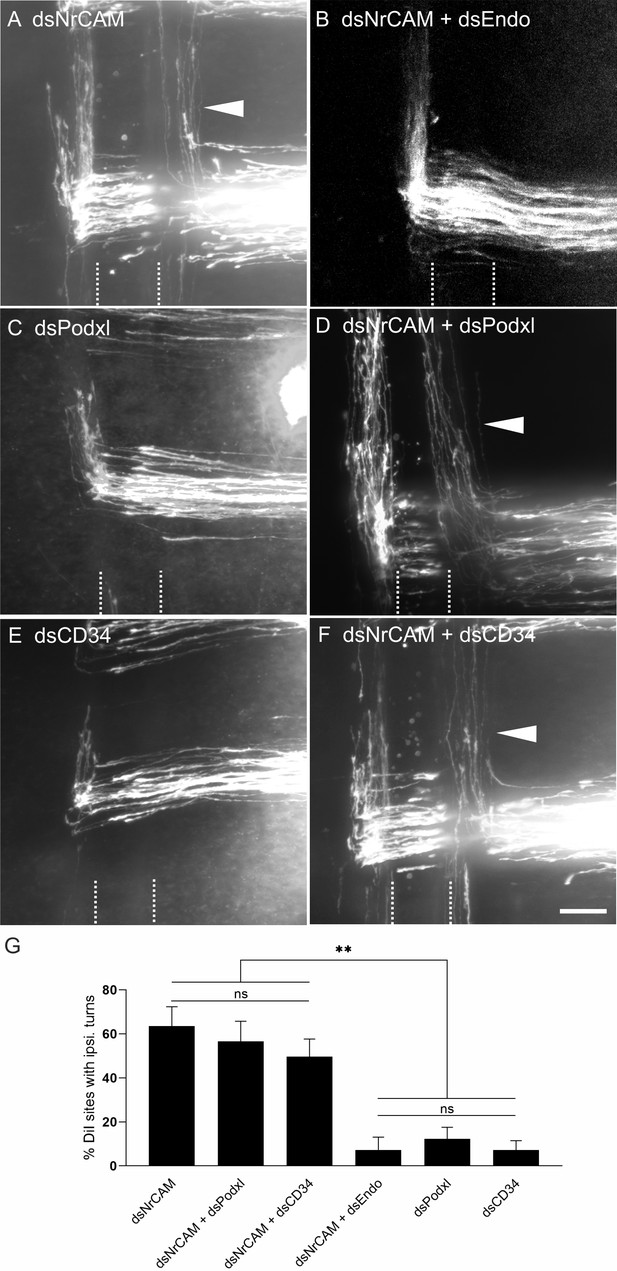
Downregulation of Endoglycan, but not its family members, rescues the axon guidance phenotype induced by downregulation of NrCAM.
The perturbation of axon/floor-plate contact by downregulation of NrCAM resulted in the failure of commissural axons to enter the floor-plate area and caused their premature turns along the ipsilateral floor-plate border (arrowhead in A) at 63.6 ± 8.8% of the injection sites (n = 7 embryos, 90 injection sites; G). These results are in line with previous reports (Stoeckli and Landmesser, 1995; Philipp et al., 2012). When both NrCAM and Endoglycan were downregulated, the number of ipsilateral turns was reduced to control levels (B,G; 7.3 ± 5.8%, n = 10 embryos; 78 injection sites), consistent with the idea that a decrease in adhesion due to a lack of NrCAM can be balanced by an increase in adhesion between floor plate and growth cones due to a lack of Endoglycan. Downregulation of either Podocalyxin (C) or CD34 (E) did not impair axon guidance (see also Figure 2; 12.3 ± 5.2% (n = 9) and 7.25 ± 4.3% (n = 8), respectively). In contrast to Endoglycan, neither concomitant downregulation of Podocalyxin (D) nor CD34 (F) could rescue the NrCAM-induced ipsilateral turns, as aberrant axon behavior was still observed at 56.7 ± 9.2% (n = 8 embryos, 77 injection sites) and 49.6 ± 8.1% (n = 10 embryos, 100 injection sites), respectively. The floor plate is indicated by dashed lines. For statistical analysis, one-way ANOVA followed by Tukey’s multiple comparisons test was used, **p<0.01 or lower, see source data and statistics in Figure 8—source data 1 spreadsheet; (ns) p≥0.05. Bar: 50 µm.
-
Figure 8—source data 1
Quantification of ipsilateral turns - raw data.
- https://cdn.elifesciences.org/articles/64767/elife-64767-fig8-data1-v2.xlsx
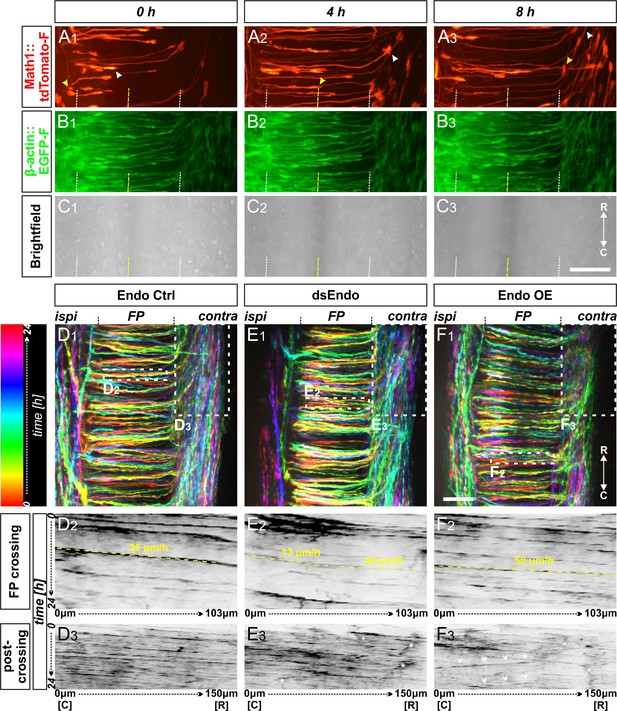
Live imaging of cultured intact spinal cords revealed major impacts of different Endoglycan levels on midline crossing.
(A-C) Live imaging allowed tracing and quantitative analysis of dI1 axons’ trajectories in cultured intact chicken spinal cords. (A1-3) The behavior and trajectory of single tdTomato-positive dI1 axons could be tracked over time when they crossed the floor plate and turned rostrally (yellow and white arrowheads). (B-C) EGFP-F expression under the β-actin promoter and brightfield images helped to visualize the floor-plate boundaries (white dashed line) and the midline (yellow dashed line). (D1, E1, F1) Temporally color-coded projections of 24 hr time-lapse movies (Video 2). Kymograph analysis of the regions of interest selected in the floor plate of each condition shown in (D1,E1,F1) was used to calculate growth cone speed during floor-plate crossing (D2, E2, F2) and after turning into the longitudinal axis (D3, E3, F3). Yellow dashed lines outline a representative example of the slope (velocity) of a single axon crossing the floor plate in each condition. TdTomato-positive axons in control-injected spinal cords (D1-3) crossed the floor plate at a steady speed of 26 µm/h (D2) and turned rostrally in a highly organized manner (D3). In contrast, growth cone speed in the first half of the floor plate that was electroporated with dsRNA derived from Endoglycan (dsEndo) was markedly slowed down to only 13 µm/hr. In the second, non-electroporated half of the floor plate, axons electroporated with dsEndo were faster than control axons (44 µm/hr; E2). Axons overexpressing Endoglycan were faster in both halves of the floor plate (50 µm/hr; F2). Downregulation or overexpression of Endoglycan clearly impacted the rostral turning behavior visualized by less organized patterns (D3-F3). Asterisks mark axons stalling and thus causing a ‘smeared’ pattern in the kymographs. Arrowheads indicate caudally turning axons. R, rostral; C, caudal; ipsi, ipsilateral; contra, contralateral; FP, floor plate. Scale bars: 50 µm.
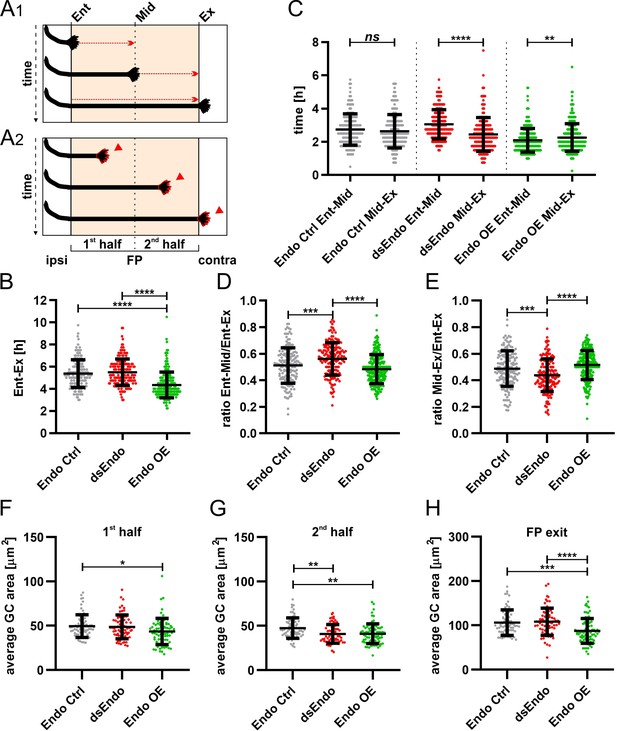
Too much or too little of Endoglycan impaired the timing and morphology of single dI1 growth cones migrating in the floor plate.
Data at the single axon level extracted from 24 hr time-lapse recordings of tdTomato-positive dI1 axons crossing the floor plate. (A1) The time of floor-plate crossing was measured for the entire floor plate, for the first and for the second half for each condition. (A2) The average growth cone area was measured in the first half, the second half and at the exit site of the floor plate for each condition. (B) Overexpression of Endoglycan significantly decreased the time axons needed to cross the entire floor plate compared to control and dsEndo conditions (Kruskal-Wallis test with Dunn’s multiple-comparisons test). (C) The average time of crossing the first half and the second half of the floor plate was compared. Interestingly, there was a highly significant difference in the spinal cords unilaterally electroporated with dsEndo, as axons spent much longer in the first compared to the second half of the floor plate. There was no difference between the two halves of the floor plate in the control condition, but there was a significant decrease in growth cone speed between the first (electroporated) half of the floor plate and the second half, where only axons were overexpressing Endoglycan (Wilcoxon test). (D) and (E) The ratios of the time axons spent in the first half (D) or the second half (E) of the floor plate divided by the time they needed to cross it entirely were compared between conditions. Unilateral knockdown of Endoglycan resulted in a significant increase of the ratio in the first half and a decrease in the second half compared to both control and overexpression conditions (one-way ANOVA with Sidak’s multiple-comparisons test). (F-H) The average dI1 growth cone area at each position of the floor plate (as depicted in A2) was compared across all conditions. (F) Overexpression of Endoglycan induced a significant reduction in the average growth cone area compared to the control condition (Kruskal-Wallis test with Dunn’s multiple-comparisons test) but not compared to Endoglycan knockdown (p value = 0.08). (G) In the second half of the floor plate, the average growth cone area was reduced in both Endoglycan knockdown and overexpression condition compared to control (one-way ANOVA with Sidak’s multiple-comparisons test). (H) At the floor-plate exit site, overexpression of Endoglycan induced a significant decrease in the average growth cone area compared to both control and knockdown conditions (one-way ANOVA with Sidak’s multiple-comparisons test). Error bars represent standard deviation. p<0.0001 (****), p<0.001 (***), p<0.01 (**), p<0.05 (*), and p≥0.05 (ns) for all tests. N(embryos)=3 for each condition; n(axons, panel C-E)=161 (Endo Ctrl), 168 (dsEndo), 234 (Endo OE); n(axons, panel F-H)=70 (Endo Ctrl), 78 (dsEndo), 83 (Endo OE). See Table 2 for detailed results. Ent, entry; Mid, midline; Ex, exit; ipsi, ipsilateral; contra, contralateral; FP, floor plate; GC, growth cone. Source data and statistics are available in Figure 10—source data 1 spreadsheet.
-
Figure 10—source data 1
Raw data of live imaging experiments.
- https://cdn.elifesciences.org/articles/64767/elife-64767-fig10-data1-v2.xlsx
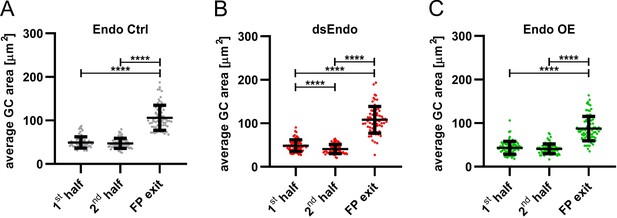
Growth cone size is enlarged at the floor-plate exit site.
Data at the single axon level extracted from 24 hr time-lapse recordings of dI1 axons crossing the floor plate. The average growth cone area was measured in the first half, the second half and at the exit site of the floor plate for each condition. (A) No difference in the area was detected between growth cones in the first half and the second half of the floor plate in controls. However, growth cones were found to be much enlarged at the exit site compared to when they were in the floor plate (Friedman test with Dunn’s multiple-comparisons test). (B) Unilateral down regulation of Endoglycan induced a significant decrease in the average growth cone area in the second part of the floor plate compared to the first part. However, dI1 growth cones still got much larger when exiting the floor plate compared to when they were located in the floor plate (one-way ANOVA with Sidak’s multiple-comparisons test). (C) After unilateral overexpression of Endoglycan, no difference in growth cone area was detected between the first and the second half of the floor plate. Like in all other conditions, they were found to be much enlarged at the exit site (Friedman test with Dunn’s multiple-comparisons test). Error bars represent standard deviation. p<0.0001 (****) for all tests. N(embryos)=3 for each condition; n(axons)=70 (Endo Ctrl), 78 (ds Endo), 83 (Endo OE). See Table 2 for detailed results. Source data and statistics are available in Figure 11—source data 1 spreadsheet.
-
Figure 11—source data 1
Raw data of growth cone size measurements.
- https://cdn.elifesciences.org/articles/64767/elife-64767-fig11-data1-v2.xlsx
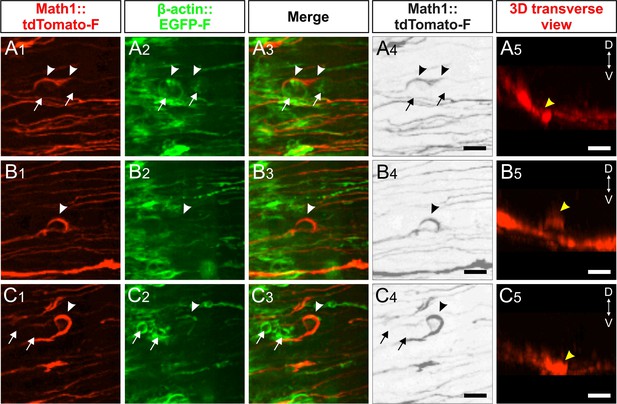
Live imaging of dI1 axons after perturbation of Endoglycan expression explains ‘corkscew-like’ phenotypes by aberrant interactions between axons and floor-plate cells.
(A-C) 'Corkscrew'-like phenotypes of dI1 axons expressing farnesylated tdTomato were observed by live imaging in the first half of the floor plate (electroporated half) only after Endoglycan was silenced (see also Videos 5 and 6). (A) Dislocated EGFP-positive cells (arrows) caused dI1 axons to deviate from a smooth trajectory inducing a 'corkscrew'-like morphology (arrowheads, A1-4). These axons and cells were located in the commissure as shown in a coronal view (yellow arrowhead, A5 and Video 5). (B) Some axons were found to form a loop (arrowheads, B1-4) invading the layer of the floor plate where somata of floor-plate cells are located as shown in the transverse view (yellow arrowheads, B5 and Video 6). (C) Clusters of dislocated roundish EGFP-positive cells (arrows) seemed to retain growth cones in the first half of the floor plate (arrowheads). These axons and cells were located in the commissure as shown in a coronal view (yellow arrowhead, C5 and Video 6). D, dorsal; V, ventral. Scale bars: 10 µm.
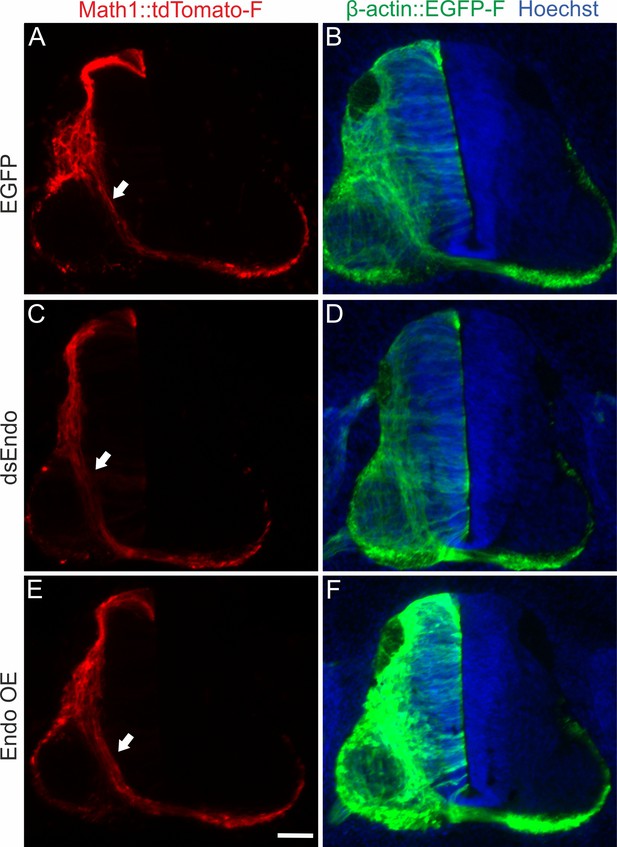
Endoglycan does not affect pre-crossing commissural axons.
We found no differences in timing or trajectories of pre-crossing commissural axons labeled by the co-electroporation of Math1::tdTomato-F, when we compared control embryos electroporated with an EGFP plasmid (A,B) with embryos electroporated with dsEndo (C,D) or embryos overexpressing Endoglycan (E,F).
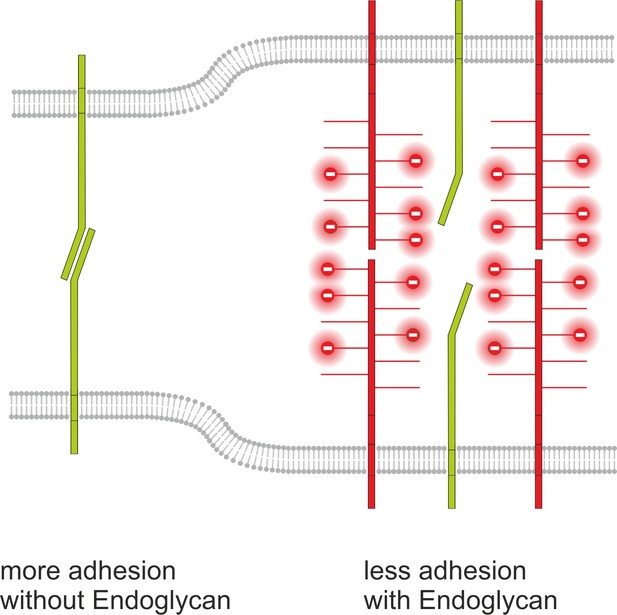
Endoglycan modulates cell-cell contact by interference with adhesive strength.
Based on our in vivo and in vitro studies, we postulate a model for Endoglycan function in neural circuit formation that suggests an ‘anti-adhesive’ role by modulation of many specific molecular interactions due to decreasing cell-cell contact. This model is consistent with our rescue experiments demonstrating that the source of Endoglycan did not matter, but the expression level did, as aberrant phenotypes were prevented, when Endoglycan was expressed either in the axon or in the floor plate.
Videos
Example of growth cone blasting assay.
Snapshots taken from a video showing the detachment of an mRFP-positive commissural neuron growth cone (shown in black, indicated by white arrowhead) on a layer of HEK cells (bright-field view) before and after detachment. The position of the tip of the glass micropipette is indicated by asterisk.
24 hr time-lapse recordings of tdTomato-positive dI1 axons (shown in black) in control (Endo control), Endoglycan knockdown (dsEndo), and Endoglycan overexpression (Endo OE) conditions.
Dashed lines represent floor plate boundaries. R, rostral; C, caudal.
Representative examples of the floor plate crossing of tdTomato-positive dI1 axons (shown in black) taken from 24 hr time-lapse recordings from control (Endo control), Endoglycan knockdown (dsEndo), and Endoglycan overexpression (Endo OE) conditions.
An arrowhead in each condition points at the migrating growth cone. Dashed lines represent floor-plate boundaries.
Example of a 24 hr time-lapse recordings of tdTomato-positive dI1 axons (shown in black) showing guidance defects at the exit site of the floor plate in Endoglycan overexpression condition.
Stalling growth cones are shown by arrowheads and caudally turning growth cones by arrows. Rostral is up. Dashed line represents the floor-plate exit site.
The trajectory of single dI1 axons in the first half of the floor plate (electroporated half) in the absence of Endoglycan was aberrant and showed a 'corkscrew'-like phenotype.
Example of a tdTomato-positive dI1 axon taken from a 24 hr time-lapse recording of an ‘Endoglycan-knockdown’ spinal cord. Arrowheads show how the growth cone is migrating within the first half (electroporated half) of the floor plate and enters in contact with mislocated EGFP-positive cells (arrows) that induced a 'corkscrew' like morphology of the shaft. The 3D coronal rotation clearly shows that the axon and cells are located within the commissure (arrowhead).
The trajectory of single dI1 axons in the first half of the floor plate (electroporated half) in the absence of Endoglycan was aberrant and showed a 'corkscrew'-like phenotype.
Example of tdTomato-farnesylated-positive dI1 axons taken from 24 hr time-lapse recordings of an ‘Endoglycan-knockdown’ spinal cord. Arrowheads show how two growth cones are migrating within the first half (electroporated half) of the floor plate. The first one made a loop within the floor-plate cell layer (arrowheads), as shown by the 3D coronal rotation. The second one was attracted toward mislocalized EGFP-positive cells (arrow), made contact with them and then carried out a U-turn toward them before continuing its migration in the direction of the midline. The 3D coronal view confirmed that the second growth cone during its U-turn and the mislocalized cells were located within the commissure (arrowhead).
Tables
The amount, but not the source of Endoglycan matters.
Treatment | No of embryos | No of inj. sites | % inj sites normal PT | p-Value | % inj sites stalling | % inj sites no turn |
---|---|---|---|---|---|---|
Untreated | 14 | 111 | 85.3 ± 2.6 | 0.999 | 0.8 ± 0.8 | 14.6 ± 2.7 |
EGFP | 14 | 85 | 80.5 ± 6.7 | 1 | 5.1 ± 2.4 | 18.6 ± 7.4 |
dsEndo | 21 | 161 | 30.2 ± 4.4 | <0.0001 | 31.6 ± 5 | 50.1 ± 3.9 |
β-actin::EndoOE | 16 | 91 | 49.1 ± 5.4 | 0.0177 | 20 ± 5.1 | 30 ± 5 |
Hoxa1::Endo-L | 25 | 234 | 38.5 ± 5.7 | <0.0001 | 24.1 ± 4.8 | 44.5 ± 5 |
Hoxa1::Endo-M | 18 | 136 | 60.3 ± 6.7 | 0.3686 | 10.9 ± 4.2 | 32.1 ± 5 |
Hoxa1::Endo-H | 7 | 72 | 24.6 ± 10.1 | <0.0001 | 40.0 ± 11.3 | 44.1 ± 7 |
Math1::Endo-L | 15 | 157 | 59.6 ± 6.8 | 0.3738 | 12.3 ± 3.5 | 30.9 ± 5.4 |
Math1::Endo-M | 7 | 86 | 35 ± 10.7 | 0.0031 | 31.7 ± 7.2 | 42.4 ± 9.3 |
Math1::Endo-H | 8 | 86 | 26.3 ± 6.5 | <0.0001 | 50.8 ± 6.4 | 40.5 ± 6.1 |
-
Concomitant expression of Endoglycan could rescue the aberrant axon guidance phenotype induced by the downregulation of Endoglycan throughout the spinal cord. It did not matter whether Endoglycan was expressed under the Hoxa1 enhancer for specific expression in floor-plate cells, or under the Math1 enhancer for specific expression in dI1 neurons. However, the rescue effect was dose-dependent. Too little, or too much Endoglycan was inducing axon guidance defects. For rescue, Endoglycan cDNA under the control of the Hoxa1 enhancer (Hoxa1::Endo) or the Math1 enhancer (Math1::Endo) were injected at 150 ng/µl (low, L), 300 ng/µl (medium, M), or 750 ng/µl (high, H). The same criteria for quantification were applied as for the results shown in Figure 2. There was no fundamental difference between too much or too little stickiness. In both conditions, we found stalling in the floor plate and failure to turn into the longitudinal axis. The only difference was that we did not find any ‘corkscrew’ phenotypes after overexpression of Endoglycan. The number of embryos and the number of DiI injection sites analyzed per group are indicated. The average % of injection sites with normal axon guidance phenotypes (± standard error of the mean) and the p-value for the comparison between the respective group and the control-treated (EGFP-expressing) group are given. The last two columns list the average values for injection sites with the majority of axons stalling in the floor plate and the majority of axons not turning into the longitudinal axis. Because some injection sites can have both phenotypes, the values do not add up to 100%.
Quantification of the number of commissural neurons adhering to a layer of HEK cells and midline crossing of dI1 axons using live imaging.
Figure 5 | |||||
---|---|---|---|---|---|
Part | Name | Value | Stdev | n(pictures) | N(replicates) |
D | HEKctrl vehic. | 1.00 | 0.35 | 34 | 4 |
D | HEKEndo-myc vehic. | 0.47 | 0.34 | 34 | 4 |
D | HEKctrl vehic.+N | 0.88 | 0.36 | 28 | 4 |
D | HEKEndo-myc vehic.+N | 1.03 | 0.49 | 28 | 4 |
D | HEKctrl vehic.+O | 1.19 | 0.70 | 29 | 4 |
D | HEKEndo-myc vehic.+O | 1.17 | 0.50 | 29 | 4 |
Figure 10 | |||||
Part | Name | Value | Stdev | n(axons) | N(embryos) |
B | Endo Ctrl | 5.38 | 1.25 | 161 | 3 |
B | dsEndo | 5.50 | 1.20 | 168 | 3 |
B | Endo OE | 4.35 | 1.38 | 234 | 3 |
C | Endo Ctrl Ent-Mid | 2.74 | 0.94 | 161 | 3 |
C | Endo Ctrl Mid-Ex | 2.64 | 1.01 | 161 | 3 |
C | dsEndo Ent-Mid | 3.06 | 0.88 | 168 | 3 |
C | dsEndo Mid-Ex | 2.45 | 1.02 | 168 | 3 |
C | Endo OE Ent-Mid | 2.10 | 0.71 | 234 | 3 |
C | Endo OE Mid-Ex | 2.26 | 0.83 | 234 | 3 |
D | Endo Ctrl | 0.51 | 0.13 | 161 | 3 |
D | dsEndo | 0.56 | 0.12 | 168 | 3 |
D | Endo OE | 0.48 | 0.11 | 234 | 3 |
E | Endo Ctrl | 0.49 | 0.13 | 161 | 3 |
E | dsEndo | 0.44 | 0.12 | 168 | 3 |
E | Endo OE | 0.52 | 0.11 | 234 | 3 |
F | Endo Ctrl | 49.49 | 12.84 | 70 | 3 |
F | dsEndo | 48.67 | 13.47 | 68 | 3 |
F | Endo OE | 43.50 | 14.84 | 83 | 3 |
G | Endo Ctrl | 47.45 | 11.58 | 70 | 3 |
G | dsEndo | 40.87 | 10.44 | 68 | 3 |
G | Endo OE | 41.09 | 11.18 | 83 | 3 |
H | Endo Ctrl | 106.05 | 28.72 | 70 | 3 |
H | dsEndo | 108.08 | 30.59 | 68 | 3 |
H | Endo OE | 87.52 | 28.01 | 83 | 3 |
Figure 11 | |||||
A | 1 st half | 49.49 | 12.84 | 70 | 3 |
A | 2nd half | 47.45 | 11.58 | 70 | 3 |
A | FP exit | 106.05 | 28.72 | 70 | 3 |
B | 1 st half | 48.67 | 13.47 | 68 | 3 |
B | 2nd half | 40.87 | 10.44 | 68 | 3 |
B | FP exit | 108.08 | 30.59 | 68 | 3 |
C | 1 st half | 43.50 | 14.84 | 83 | 3 |
C | 2nd half | 41.09 | 11.18 | 83 | 3 |
C | FP exit | 87.52 | 28.01 | 83 | 3 |
Reagent type (species) or resource | Designation | Source or reference | Identifiers | Additional information |
---|---|---|---|---|
Antibody | Anti-digoxigenin-AP antibody (rabbit polyclonal) | Roche | RRID:AB_514497 | ISH (1:10000) |
Antibody | Anti-myc (rabbit polyclonal) | Abcam | RRID: AB_307014 | IF (1:500) |
Antibody | Anti-Hnf3β (mouse monoclonal) | DSHB | RRID: AB_2278498 | IF (supernatant) |
Antibody | Anti-Axonin-1 (goat polyclonal) | Stoeckli and Landmesser, 1995 | N/A | IF (1:500) |
Antibody | Anti-Axonin-1 (rabbit polyclonal) | Stoeckli and Landmesser, 1995 | N/A | IF (1:1000) |
Antibody | Anti-neurofilament-M (RMO-270, mouse monoclonal) | ThermoFisher Scientific | RRID: AB_2532998 | IF (1:200) |
Antibody | Anti-GFP-FITC (goat polyclonal) | Rockland | RRID: AB_218187 | IF (1:400) |
Antibody | Anti-NrCAM(mouse monoclonal) | Fitzli et al., 2000 | N/A | IF (1:1000) |
Antibody | Anti-Islet1 (mouse monoclonal) | DSHB | RRID: AB_528315 | IF (supernatant) |
Antibody | Anti-Nkx2.2 (mouse monoclonal) | DSHB | RRID: AB_531794 | IF (supernatant) |
Antibody | Anti-Pax3 (mouse monoclonal) | DSHB | RRID: AB_528426 | IF (supernatant) |
Antibody | Anti-Pax6 (mouse monoclonal) | DSHB | RRID: AB_528427 | IF (supernatant) |
Antibody | Cleaved Caspase-3 (rabbit polyclonal) | Cell Signaling | RRID: AB_2341188 | IF (1:200) |
Cell line (H. sapiens) | HEK293T | American Type Culture Collection | RRID: CVCL_0063 | |
Cell line (H. sapiens) | HEK293T-PODXL2-myc(Endo-myc) | This paper | N/A | |
Recombinant DNA reagent | Math1::chEndoglycan (plasmid) | This paper | N/A | |
Recombinant DNA reagent | Hoxa1::chEndoglycan (plasmid) | This paper | N/A | |
Recombinant DNA reagent | β-actin::chEndoglycan (plasmid) | This paper | N/A | |
Recombinant DNA reagent | β-actin::EGFP-F (plasmid) | This paper | N/A | |
Recombinant DNA reagent | β-actin::mRFP (plasmid) | Wilson and Stoeckli, 2011 | N/A | |
Recombinant DNA reagent | Math1::tdTomato-F (plasmid) | Wilson and Stoeckli, 2011 | N/A | |
Chemical compound, drug | O-glycosidase and Neuraminidase | NEB | Cat# E0540S | |
Software, algorithm | Fiji/ImageJ | Schindelin et al., 2012 | RRID:SCR_002285 | |
Biological sample (Gallus gallus) | Hubbard JA57 strain | Brüterei Stöckli, Ohmstal | N/A |