The role of sigma 1 receptor in organization of endoplasmic reticulum signaling microdomains
Figures
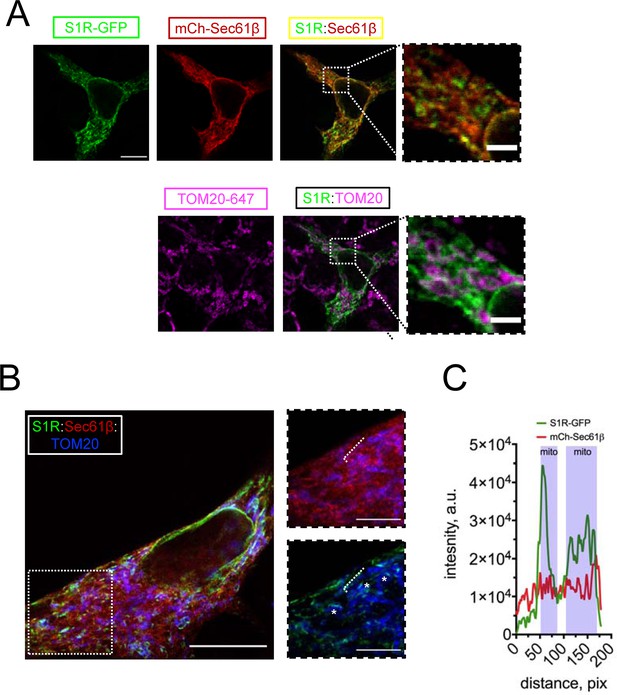
S1R targeting to mitochondria-associated membranes (MAMs) in HEK293 cells.
(A) Intracellular distribution of wild-type S1R-GFP (green) was visualized in HEK293 cells by co-expressing an endoplasmic reticulum (ER) marker mCherry-Sec61β (red) and by immunostaining with a mitochondrial marker TOM20 (magenta). Scale bar = 10 μm; insets = 2.5 μm. (B) Specimen was processed according to the protein retention expansion microscopy procedure, with S1R-GFP in green, mCherry-Sec61β in red, and TOM20 staining in blue. Insets show double staining of mCherry-Sec61β and TOM20 (top), S1R-GFP and TOM20 (bottom). Putative MAM compartments are labeled with asterisks. Scale bars = 5 μm; insets = 1.5 μm (real space). (C) Intensity profiles for S1R-GFP (green) and mCherry-Sec61β (red) channels along the ER tubule (labelled with a white dashed line on B). Mitochondrial proximity regions (shaded blue) were identified based on the intensity of TOM20.
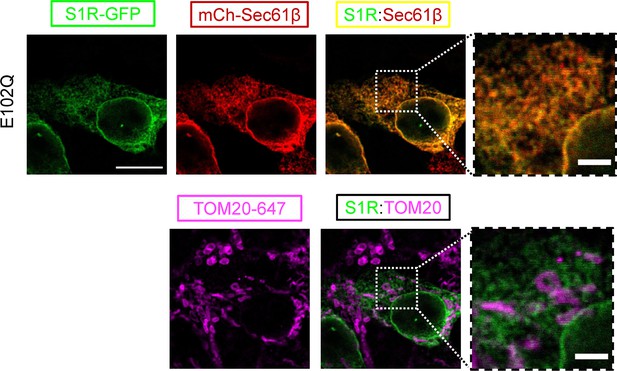
Intracellular distribution of the S1R-E102Q mutant.
Intracellular distribution of an ALS mutant protein S1R-E102Q was visualized in HEK293 cells by co-expressing an endoplasmic reticulum marker mCherry-Sec61β (red) and by immunostaining with a mitochondrial marker TOM20 (magenta).
Scale bar = 10 μm; insets = 2.5 μm.
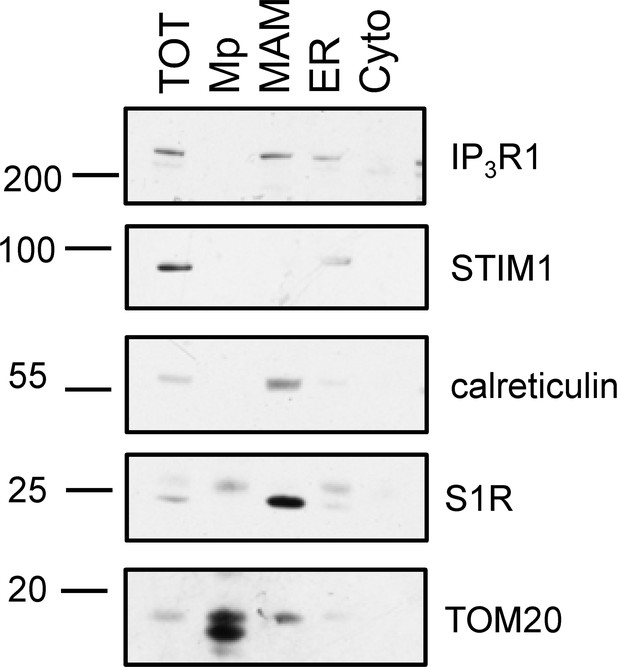
Purification of MAMs from mice liver.
Subcellular fractions were isolated as described in Wieckowski et al., 2009 and immunostained with the following antibodies: TOM20 (a mitochondrial marker), S1R, calreticulin (an ER/MAM marker), STIM1 (an ER marker), and IP3R1 (an ER channel enriched in MAMs). TOT: total tissue lysate; Mp: purified mitochondrial fraction; MAM: mitochondria-associated membranes; ER: microsomal fraction; Cyto: cytosol.
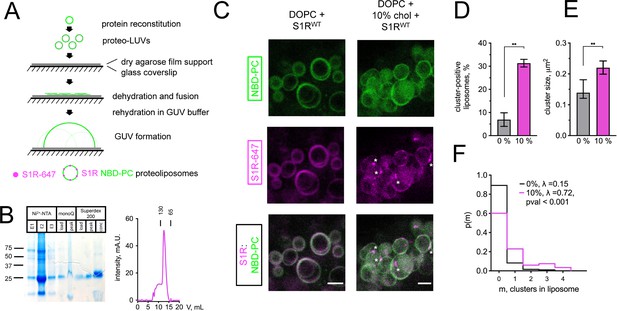
Cholesterol-dependent clustering of S1R in giant unilamellar vesicles (GUVs).
(A) The GUV formation procedure. Purified S1R was labeled with Alexa647 and reconstituted into large unilamellar vesicles (LUVs) to form proteoliposomes. After controlled dehydration of proteoliposomes on an agarose-covered coverslip, films were rehydrated in a salt buffer, which resulted in the formation of micrometer-size GUVs. (B) Biochemical characterization of purified S1R by SDS-PAGE analysis (left) and size-exclusion chromatography (right). (C) Distribution of S1R-647 in cholesterol-free DOPC liposomes (left) and in the presence of 10 mol % cholesterol (right), with membrane dye NBD-labeled phosphatidylcholine (NBD-PC) shown in green and S1R-647 in magenta. S1R clusters are marked with asterisks. Scale bars = 5 μm. (D) Average fraction of cluster-positive liposomes. In each experiment, 7–20 fields of view were quantified blindly. Data is mean ± SEM from n = 3 independent experiments (0% condition: 506 liposomes, 10% condition: 685 liposomes). **p=0.003 based on two-tailed t-test. (E) Distribution of the S1R cluster size in GUV in the absence and presence of cholesterol. Data is mean ± 95% CI. **p=0.002 based on two-tailed t-test. (F) Distribution of the number of clusters per liposome in the absence (black) and presence of 10 mol % cholesterol (magenta). Data was fitted with Poisson distribution to estimate the average number of clusters per liposome, λ (N1 = 194 liposomes for 0%, N2 = 201 for 10 mol % cholesterol experimental condition). ****p-value<0.001 calculated based on Whitehead’s and C-test statistical tests.
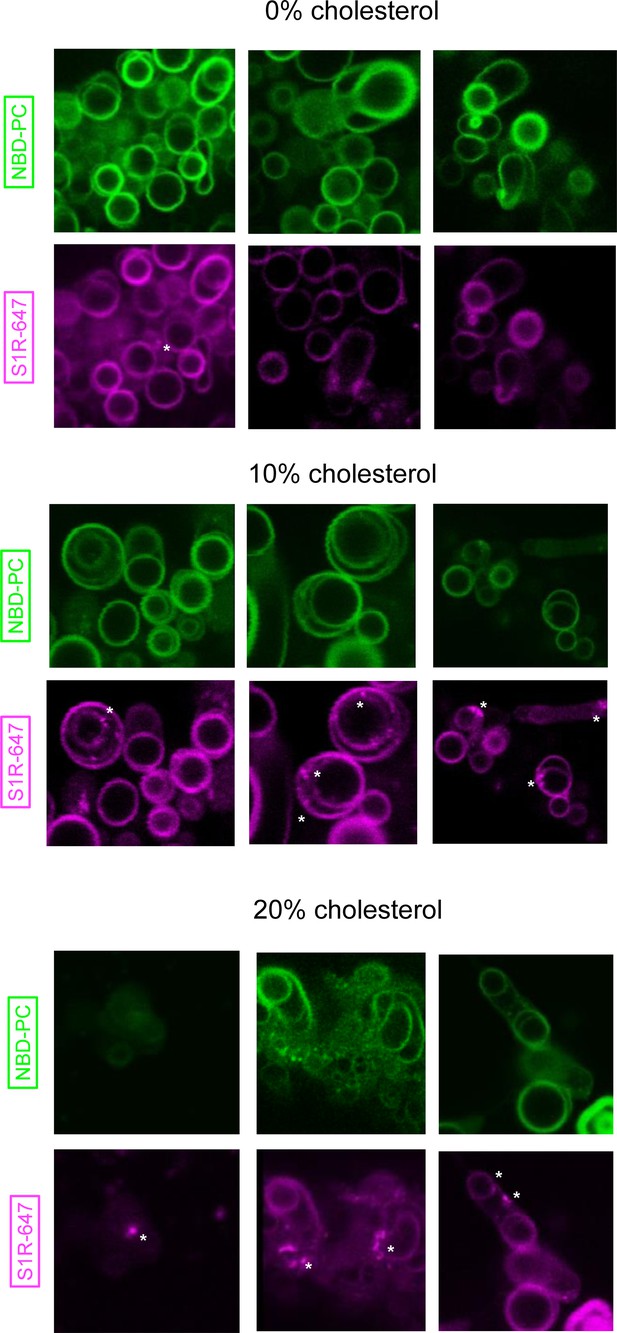
Additional examples of S1R distribution in DOPC giant unilamellar vesicles at 0, 10, and 20 mol % cholesterol.
Membrane dye NBD-labeled phosphatidylcholine (NBD-PC) is shown in green, and S1R-647 is shown in magenta.
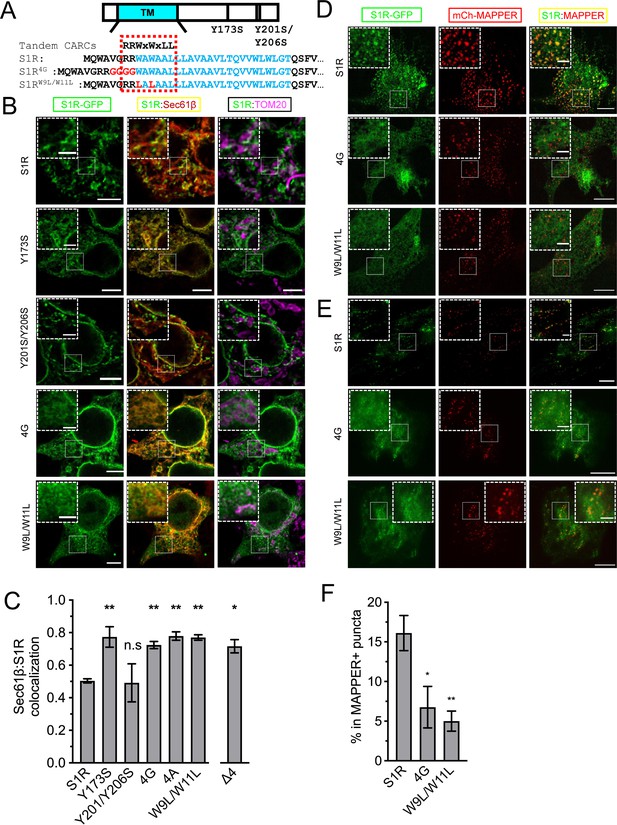
Cholesterol-binding motifs in the S1R sequence.
(A) A schematic representation of the S1R primary sequence with its transmembrane helix (TM) in cyan. Previously proposed cholesterol-binding residues (Y173, Y201/Y206) (Palmer et al., 2007) are marked. Sequence analysis identified tandem CARC binding motifs (indicated by a dashed red line) in the TM region (marked in cyan font). Mutations were introduced either by insertion of the four-glycine repeat (4G) or by mutation of the two critical tryptophan residues to leucines (W9L/W11L). (B) Intracellular distribution of the WT receptor and cholesterol-binding mutants in HEK293 cells, S1R-GFP in green, mCherry-Sec61β in red, and anti-TOM20 in magenta. Scale bars = 5 μm, insets = 2.5 μm. (C) Quantification of the Mander’s colocalization coefficient between the mCherry-Sec61β and S1R-GFP for WT receptor and mutant forms. Data is mean ± SEM from n = 3 independent experiments (n = 2 for Y173S, Y201S/Y206S, 4A). p-values (n.s. p>0.05, *p<0.05, **p<0.01): Y173S vs. WT: p=0.007, Y201S/Y206S vs. WT: p>0.999, 4G vs. WT: p=0.014, 4A vs. WT: p=0.006, W9L/W11L vs. WT: p=0.003, Δ4 vs. WT: p=0.017, based on ANOVA test with Dunnett’s post hoc test. (D) Distribution of the WT S1R-GFP, S1R-4G, and S1R-W9L/W11L mutants (in green) in HEK293 cells in the confocal plane near the plasma membrane (PM). Endoplasmic reticulum (ER)-PM junctions were visualized with a genetically encoded marker MAPPER (in red). Scale bars = 5 μm, insets = 1.5 μm. (E) Distribution of the WT S1R-GFP, S1R-4G, and S1R-W9L/W11L mutants (in green) in HEK293 cells visualized using total internal reflection fluorescence microscopy, with ER-PM junctions labeled with MAPPER (in red). Scale bars = 5 μm, insets = 1.5 μm. (F) Fraction of S1R residing in the MAPPER-positive puncta, calculated from data shown in (E). p-values (*p<0.05, **p<0.01): 4G vs. WT: p=0.025, W9L/W11L vs. WT: p=0.008, based on ANOVA test with Dunnett’s post hoc test.
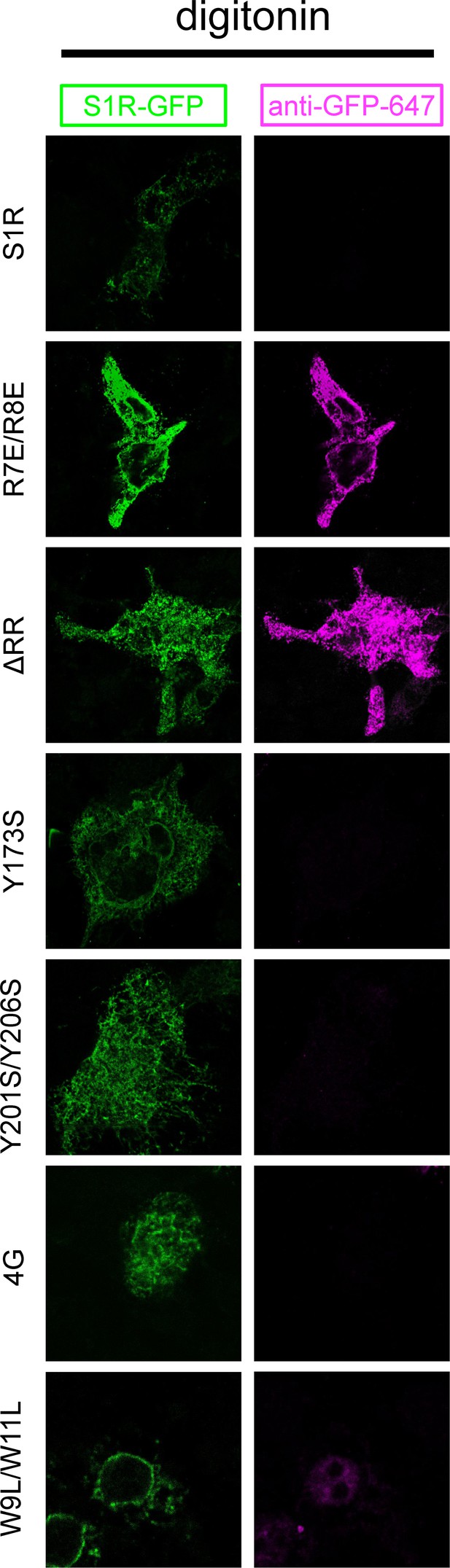
Membrane topologies of the S1R-GFP mutants.
HEK293 cells expressing WT S1R-GFP and mutants (in green) were permeabilized with digitonin and stained with primary anti-GFP antibody and Alexa647-conjugated secondary antibody (in magenta).
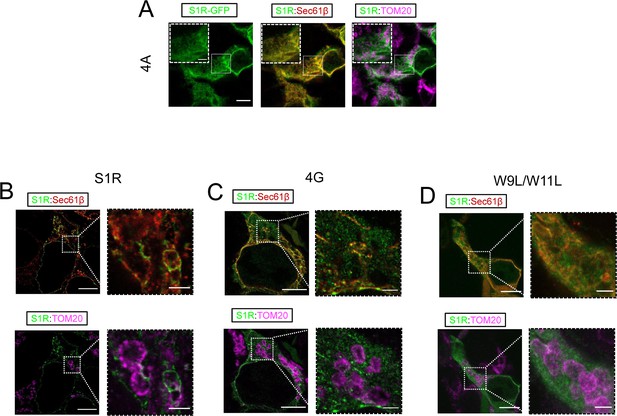
Additional characterization of the distribution of S1R-GFP mutants in cells.
(A) Intracellular distribution of S1R-4A in HEK293 cells, S1R-4A in green, mCherry-Sec61β in red, and anti-TOM20 in magenta. Scale bars = 10 μm, insets = 2.5 μm. (B) Distribution of WT S1R-GFP, S1R-4G (C), and S1R-W9L/W11L (D) mutants was visualized using the protein retention expansion microscopy in HEK293 cells. Double staining showing distribution of S1R-GFP (in green) and mCherry-Sec61β (in red) (top panels), S1R-GFP and anti-TOM20 (in magenta) (bottom panels). Scale bars = 5 μm, zoomed areas = 1 μm (real space).
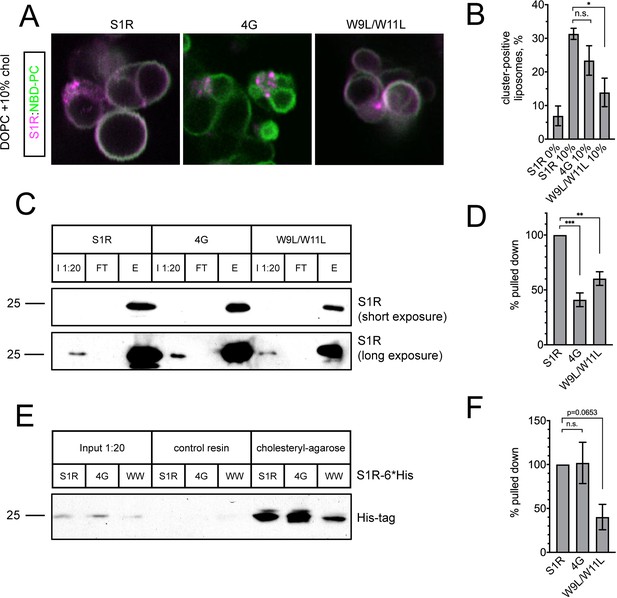
Cholesterol binding of the S1R CARC mutants.
(A) Distribution of purified S1R-4G and S1R-W9L/W11L in cholesterol-containing giant unilamellar vesicles (GUVs) with S1R-647 in magenta and NBD-labeled phosphatidylcholine (NBD-PC) in green. (B) Average fraction of cluster-positive liposomes for S1R, S1R-4G, and S1R-W9L/W11L. Cluster-positive liposomes were quantified as in Figure 2D. Data (mean ± SEM) is from n = 3 independent experiments (WT 10% condition: 685 liposomes; 4G: 539 liposomes; W9L/W11L: 638 liposomes). p-values (n.s.: non-significant, *p-value<0.05): WT 10% vs. 4G 10%: p=0.31, W9L/W11L 10% vs. WT 10%: p=0.02 based on ANOVA test with Dunnett’s post hoc test. (C) Cholesterol-coupled agarose pulldown with recombinant S1R protein. I 1:20: input; FT: flow-through; E: eluted protein. Proteins were analyzed with western blot analysis using anti-S1R antibodies. (D) Quantification of western blot results shown in (C). Data (mean ± SEM) is from n = 3 independent experiments. Measured band intensities of eluted proteins were divided by the measured band intensities of the inputs and normalized to S1R-WT. p-values (**p-value<0.01, ***p-value<0.001): 4G vs. WT: p=0.0003, W9L/W11L vs. WT: p=0.003 based on ANOVA test with Dunnett’s post hoc test. (E) Cholesterol agarose pulldown with S1R-6His proteins exogenously expressed in HEK293 cells. Proteins were analyzed using western blot analysis with anti-His-tag antibodies. (F) Quantification of western blot results shown in (E). Data (mean ± SEM) is from n = 3 independent experiments. Measured band intensities of eluted proteins were divided by the measured band intensities of the inputs and normalized to S1R-WT. p-values: 4G vs. WT: p=0.995, W9L/W11L vs. WT: p=0.065 based on ANOVA test with Dunnett’s post hoc test.
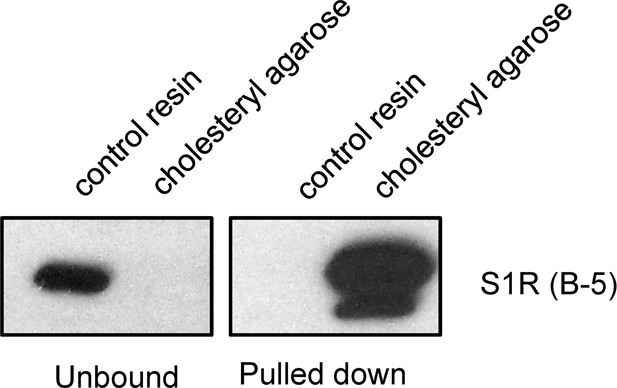
Binding of recombinant S1R to control resin (CarboxyLink agarose) and cholesterol-coupled agarose.
Left panel: unbound fraction after incubation with resins; right panel: pulled down proteins.
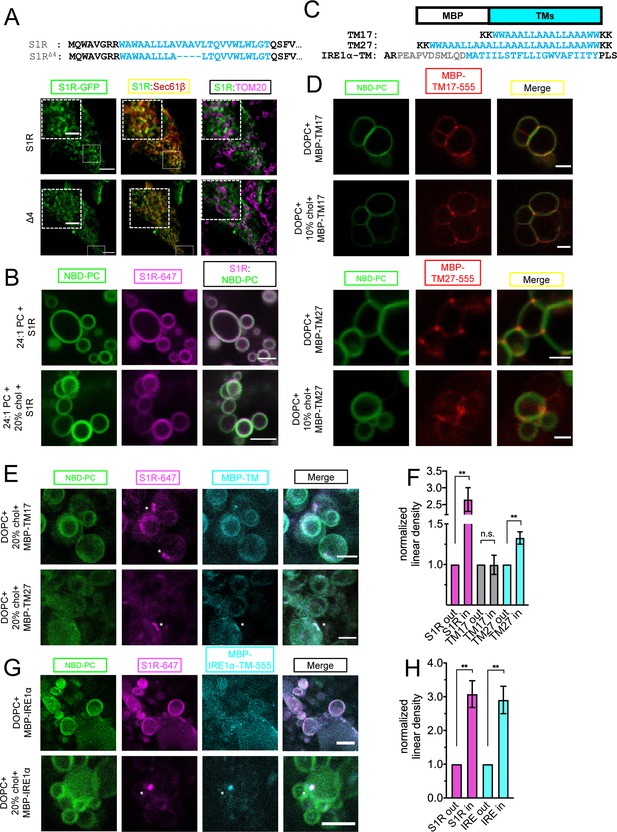
Importance of membrane bilayer thickness for S1R cluster formation.
(A) Deletion of the four amino acid stretch from the S1R transmembrane (TM) domain (top) and intracellular localization of full-length (WT) and shortened mutant (S1R-Δ4) in HEK293 cells. S1R-GFP in green, mCherry-Sec61β in red, and anti-TOM20 in magenta. Scale bars = 10 μm, insets = 2.5 μm. Mander’s colocalization coefficient for the Δ4 mutant in plotted on Figure 3C. (B) Distribution of S1R-647 (magenta) in 24:1 phosphatidylcholine (PC) giant unilamellar vesicles (GUVs) (NBD-PC in green) in the absence (top) or presence of 20 mol % cholesterol (bottom). Scale bars = 10 μm. (C) Construct design and primary amino acid sequences of MBP-TM17, -TM27, and -IRE1α-TM proteins. Synthetic TM domain in shown in cyan. Construct design of MBT-TMs was based on Kaiser et al., 2011. TM helix of IRE1α is shown in cyan and the adjacent amphipathic helix is in gray. Construct design of MBP-IRE1α-TM was based on Cho et al., 2019. (D) Distribution of purified MBP-TM17-555 (top six panels) and MBP-TM27-555 (lower six panels) in DOPC GUVs in the absence or presence of 10 mol % cholesterol.MBP-TMs are shown in red and NBD-PC in green. Scale bars = 5 μm (MBP-TM17 panels) and 2.5 μm (MBP-TM27 panels). (E) Distribution of S1R-647 (magenta) co-reconstituted together with MBP-TM17-555 (cyan, top panel) or MBP-TM27-555 (bottom panel) in DOPC GUVs in the presence of cholesterol (NBD-PC in green). S1R clusters are labeled with asterisks. Scale bars = 5 μm (MBP-TM17) and 2.5 μm (MBP-TM27). (F) Linear density of S1R-647 (magenta), MBP-TM17-555 (gray), and MBP-TM27-555 (cyan) outside and inside S1R clusters. Data is mean ± SEM. p-values (n.s. p>0.05, ** p-value<0.01): S1R in vs. S1R out (n = 9): p-value=0.002, TM17 in vs. TM17 out (n = 5): p-value=0.975, TM27 in vs. TM27 out (n = 8): p-value=0.004 based on two-tailed t-test. (G) Distribution of S1R-647 (magenta) co-reconstituted together with MBP-IRE1α-TM-555 (cyan) in GUV (NBD-PC in green) in the absence (top) and presence (bottom) of 20 mol % cholesterol. S1R cluster is labeled with an asterisk. Scale bars = 10 μm (top panels) and 5 μm (bottom panels). (H) Linear density of S1R-647 (magenta) and MBP-IRE1α-TM-555 (cyan) outside and inside S1R clusters. Data is mean ± SEM. p-values: S1R in vs. S1R out (n = 6): **p-value=0.003, IRE1α in vs. IRE1α out (n = 6): ** p-value=0.005 based on two-tailed t-test.
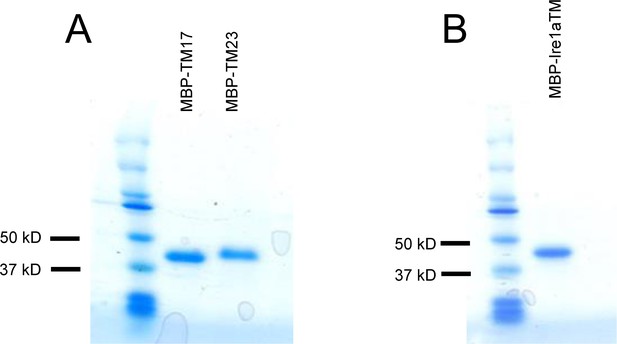
Purification of MBP-TM17, -TM27 and IRE1α-TM.
(A) SDS-PAGE analysis of purified MBP-TM17 and MBP-TM27 proteins. (B) SDS-PAGE analysis of purified MBP-IRE1α-TM protein.
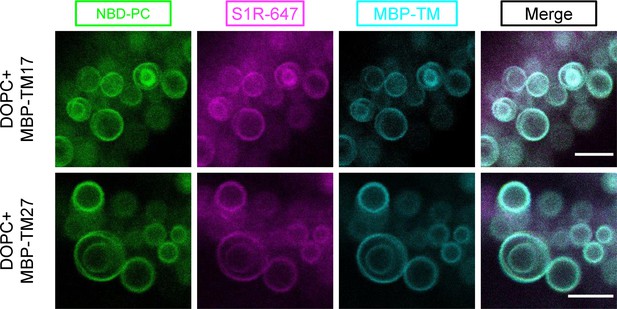
Distribution of S1R-647 and MBP-TMs in the absence of cholesterol.
S1R-647 (magenta) was co-reconstituted together with MBP-TM17-555 (cyan, top panel) or MBP-TM27-555 (bottom panel) in DOPC giant unilamellar vesicles in the absence of cholesterol (NBD-labeled phosphatidylcholine [NBD-PC] in green).
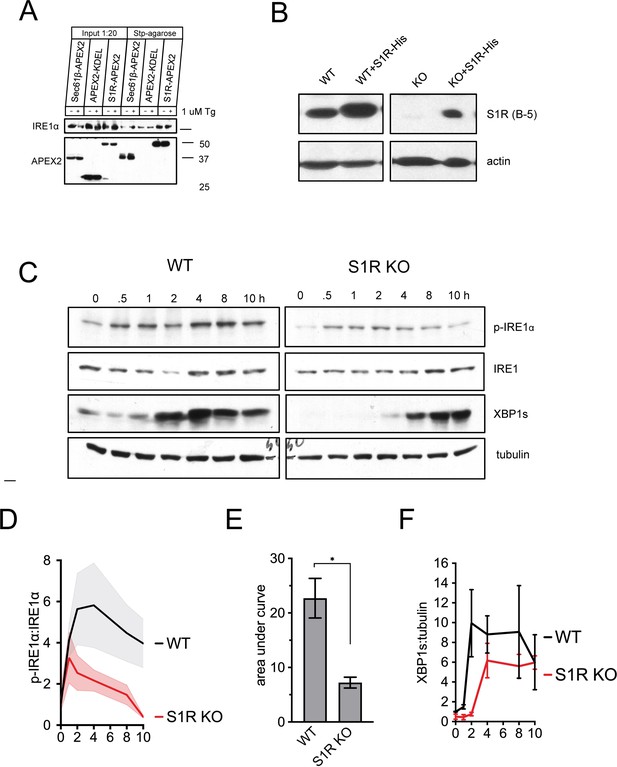
Impairement of the IRE1α signaling in S1R KO HEK293 cells.
(A) Proximity labeling of IRE1α. HEK293 cells expressed S1R-APEX2 and Sec61β-APEX2 or APEX2-KDEL as controls. Cells were incubated with biotin-phenol, and proteins were labeled by a 1 min addition of H2O2. Biotinylated proteins were pulled down and analyzed by western blot. (B) Western blot analysis of lysates prepared from control HEK293 cells, S1R KO HEK293 cells, and both cell lines transfected with S1R-6His construct as indicated. (C) Time-dependence of the thapsigargin (Tg)-induced IRE1α response in WT and S1R KO HEK293 cells. Western blot is representative of n = 3 independent experiments. (D) Quantification results of the western blot shown above. p-IRE1α levels were normalized to the total level of IRE1α and plotted as mean (solid line) ± SEM (shaded) from n = 3 independent experiments for WT (black) and S1R KO cells (red). (E) Quantification results for area under the curve of the Tg-induced p-IRE1α response. p-value=0.015 based on t-test. (F) Quantification results of the western blot results for XBP1s. Intensities of protein bands were normalized to tubulin and plotted as mean ± SEM from n = 3 independent experiments for WT (black) and S1R KO cells (red).
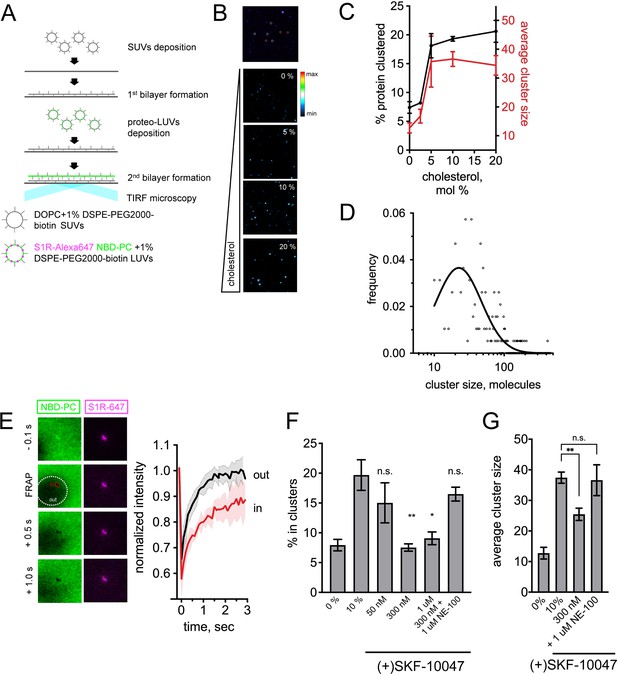
Visualization of S1R clusters in double supported lipid bilayers (DLSBs).
(A) The DSLB technique. Small unilamellar vesicles (SUVs) prepared from DOPC and 1% PEGylated lipids are deposited on a glass surface. S1R-Alexa647 (magenta) is reconstituted into large unilamellar vesicles (LUVs) doped with 1% PEGylated lipids and NBD-labeled phosphatidylcholine (NBD-PC) dye (green). Proteoliposomes are deposited on a pre-formed first bilayer. Bilayer is imaged using total internal reflection fluorescence (TIRF) microscopy. (B) Detection of S1R clusters in DSLB (top image). Trimeric receptor (orange circles), bigger assemblies (white circles), and large clusters (magenta) can be identified in DSLBs. Cholesterol-dependent clustering of S1R-647 in DSLB (bottom images). Heat map coloring scheme was used for better visibility. (C) Fraction of the clustered receptor(black, left Y-axis) versus membrane cholesterol concentration. In each well, at least 10 fields of view were quantified, and experiments were repeated independently n = 3 times (n = 1 for 2.5%, n = 2 for 5.0%). Average cluster size was calculated (red, right Y-axis) for 10 fields of view at each cholesterol concentration. (D) Size distribution of higher-order S1R clusters (more than 10 molecules) in DSLB at 20 mol% cholesterol based on their fluorescent intensity (open circles) and lognormal fit of the data (solid line). (E) Lipid dynamics in S1R clusters measured by fluorescence recovery after photobleaching (FRAP). A small area of the bilayer was bleached, and fluorescence recovery of NBD-PC dye was monitored outside (out, white area) and inside (in, red area) of a S1R cluster. Normalized FRAP curves (red within the cluster, black for outside bilayer) are plotted from n = 3 FRAP experiments. (F) Ligand effects of S1R agonist and antagonist on S1R clustering in DSLB. Increasing concentrations (50 nM to 1 μM) of a selective S1R agonist (+)-SKF-10047 were added to the wells during the second bilayer formation step (2 hr) and clustered fraction was quantified. S1R antagonist NE-100 at 1 μM concentration was added together with 300 nM (+)-SKF-100047. Data are mean ± SEM. In each experiment, at least 10 fields of view were analyzed. p-values (n.s. p>0.05, *p-value<0.05, **p<0.01): 0% vs. 10%: p=0.005, 50 nM vs. 10%: p=0.974, 300 nM vs. 10%: p=0.003, 1 μM vs. 10%: p=0.136, 300 nM +1 μM NE-100: p=0.985 based on one-way ANOVA with Tukey’s post hoc test. (G) Ligand effects of (+)-SKF-10047 on the size of the protein clusters. Average cluster size calculated for at least 10 fields of view for each experimental condition. Data is mean ± SEM. (n.s. p>0.05, **p<0.01): 0% vs. 10%: p=0.0001, 10% vs. 300 nM: p=0.004, 10% vs. 300 nM + 1 μM NE-100: p>0.999 based on one-way ANOVA with Tukey’s post hoc test.
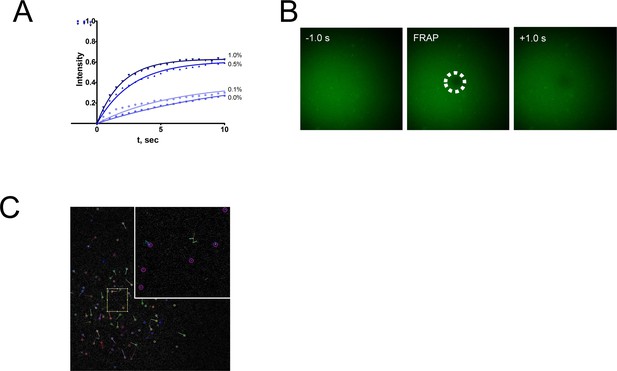
Characterization of the double supported lipid bilayer technique.
(A) Fluorescence recovery after photobleaching curves of the NBD-PC in the second bilayer as a function of DSPE-PEG2000-biotin concentration. (B) Recovery of the NBD-PC in the second bilayer after photobleaching, at 1.0% DSPE-PEG2000-biotin concentration. (C) Single-molecule detection of highly diluted S1R-647 at lipid-to-protein ratio of 20,000:1.
Tables
Reagent type (species) or resource | Designation | Source or reference | Identifiers | Additional information |
---|---|---|---|---|
Gene (Homo sapiens) | SIGMAR1 | GenBank | NM_005866 | |
Gene (synthetic gene) | TM17 | GenScript | AAGAAATGG TGGGCTGCG GCGCTGTTA GCTGCGGCG TTACTGGCTG CGGCGTGGT GGAAGAAATAA | |
Gene (synthetic gene) | TM27 | GenScript | AAGAAATGG TGGGCTGCG GCGCTGTTAG CTGCGGCGTT ACTGGCTGCG GCGCTGCTTG CTGCGGCGTT ATTAGCTGCG GCGTGGTGGA AGAAATAA | |
Gene, fragment (Homo sapiens) | IRE1a-TM | GenScript | GCGCGTCCGG AGGCGCCGGT GGACAGCATGC TGCAGGATATG GCGACCATCAT TCTGAGCACCT TCCTGCTGATC GGTTGGGTTGC GTTTATCATTAC CTACCCGCTGA GCAAG | |
Strain, strain background (Escherichia coli) | BL21(DE3) | Thermo Fisher | C600003 | Chemical competent cells |
Cell line (Homo sapiens) | HEK293T | ATCC | 3216 | |
Cell line (Homo sapiens) | HEK293 S1R KO | This study | Generated by co-transfection with Cas9 and guide plasmids, following by antibiotic selection with puromycin and blasticidin | |
Biological sample (Mus musculus) | Liver | The Jackson Laboratory | 000664 | Freshly isolated from C57/B6 mouse strain |
Antibody | Anti-phospho-IRE1a (rabbit polyclonal) | Novus Biologicals | NB100-2323 | WB (1:10,000) |
Antibody | Anti-IRE1a (rabbit monoclonal) | Cell Signaling | 14C10 | WB (1:1000) |
Antibody | Anti-XBP1s (rabbit monoclonal) | Cell Signaling | E9V3E | WB (1:1000) |
Antibody | Anti-tubulin (mouse monoclonal) | DSHB | E7 | WB (1:500) |
Antibody | Anti-APX2-HRP (rabbit polyclonal) | Abcam | ab192968 | WB (1:1000) |
Antibody | Anti-S1R (mouse monoclonal) | Santa Cruz | B-5 | WB (1:300) |
Antibody | Anti-His-tag (mouse monoclonal) | Millipore | HIS.H8 | WB (1:1000) |
Antibody | Anti-STIM1 (rabbit polyclonal) | Cell Signaling | 4916 | WB (1:1000) |
Antibody | Anti-calreticulin (rabbit polyclonal) | Abcam | Ab2907 | WB (1:5000) |
Antibody | Anti-IP3R1 (rabbit polyclonal) | Home made | WB (1:1000) | |
Antibody | Anti-TOM20 (rabbit monoclonal) | Cell Signaling | D8T4N | WB (1:1000) IF (1:250) |
Antibody | Anti-GFP (chicken polyclonal) | Abcam | ab13970 | IF (1:1000–1:500) |
Antibody | Anti-mCherry (rat monoclonal) | Invitrogen | 16D7 | IF (1:1000–1:500) |
Antibody | Anti-TOM20 (rabbit polyclonal) | Santa Cruz | FL-145 | IF (1:500) |
Antibody | Anti-mouse-HRP (goat polyclonal) | Jackson ImmunoResearch | 115-035-146 | WB (1:3000) |
Antibody | Anti-rabbit-HRP (goat polyclonal) | Jackson ImmunoResearch | 115-035-144 | WB (1:3000) |
Antibody | Anti-chicken-488 (goat polyclonal) | Invitrogen | A11039 | IF (1:1000) |
Antibody | Anti-rat-594 (donkey polyclonal) | Invitrogen | A21209 | IF (1:1000) |
Antibody | Anti-rabbin-Atto647N (goat polyclonal) | Sigma-Aldrich | 40839 | IF (1:1000) |
Recombinant DNA reagent | pFast-Bac-HTA | Invitrogen | 10584027 | |
Recombinant DNA reagent | Lentivector | Addgene | 14883 | |
Recombinant DNA reagent | pEGFP-N2 | Clontech | PR29968 | |
Recombinant DNA reagent | LentiGuide-Puro | Addgene | 52963 | |
Recombinant DNA reagent | Lenti-Cas9-Blast | Addgene | 52962 | |
Recombinant DNA reagent | S1R-GFP | This study | Fusion gene of S1R and GFP in pEGFP-N2 vector | |
Recombinant DNA reagent | S1R-R7ER8E-GFP | This study | Fusion gene of S1R and GFP in pEGFP-N2 vector with introduced R7ER8E mutation | |
Recombinant DNA reagent | S1R-ΔRR-GFP | This study | Fusion gene of S1R and GFP in pEGFP-N2 vector with introduced ΔRR mutation | |
Recombinant DNA reagent | S1R-4G-GFP | This study | Fusion gene of S1R and GFP in pEGFP-N2 vector with introduced 4G mutation | |
Recombinant DNA reagent | S1R-4A-GFP | This study | Fusion gene of S1R and GFP in pEGFP-N2 vector with introduced 4A mutation | |
Recombinant DNA reagent | S1R-W9L/W11L-GFP | This study | Fusion gene of S1R and GFP in pEGFP-N2 vector with introduced W9L/W11L mutations | |
Recombinant DNA reagent | S1R-Y173S-GFP | This study | Fusion gene of S1R and GFP in pEGFP-N2 vector with introduced Y173S mutation | |
Recombinant DNA reagent | S1R-Y201S/Y206S-GFP | This study | Fusion gene of S1R and GFP in pEGFP-N2 vector with introduced Y201S/Y206S mutations | |
Recombinant DNA reagent | S1R-Δ4-GFP | This study | Fusion gene of S1R and GFP in pEGFP-N2 vector with introduced Δ4 mutation | |
Recombinant DNA reagent | mCherry-Sec61b | Addgene | 49155 | Zurek et al. Traffic. 2011 |
Recombinant DNA reagent | mCherry-MAPPER | This study | mCherry-MAPPER was generated by replacing GFP with mCherry in the original construct from Chang et al. Cell Reports, 2013 | |
Recombinant DNA reagent | His-S1R | This study | His-tagged S1R in pFastBac-HTA vector | |
Recombinant DNA reagent | His-S1R-4G | This study | His-tagged S1R in pFastBac-HTA vector with introduced 4G mutation | |
Recombinant DNA reagent | His-S1R-W9L/W11L | This study | His-tagged S1R in pFastBac-HTA vector with introduced W9L/W11L mutations | |
Recombinant DNA reagent | S1R-6His | This study | His-tagged S1R in lentivector | |
Recombinant DNA reagent | S1R-4G-6His | This study | His-tagged S1R in lentivector with introduced 4G mutation | |
Recombinant DNA reagent | S1R-W9L/W11L-6His | This study | His-tagged S1R in lentivector with introduced W9L/W11L mutations | |
Recombinant DNA reagent | MBP-TM17 | This study | Generated by cloning TM17 into pMAL-c5x vector | |
Recombinant DNA reagent | MBP-TM27 | This study | Generated by cloning TM27 into pMAL-c5x vector | |
Recombinant DNA reagent | MBP-IRE1a-TM | This study | Generated by cloning IRE1a-TM into pMAL-c5x vector | |
Recombinant DNA reagent | S1R-APEX2 | This study | Generated by cloning S1R-APEX2 fusion gene into lentivector | |
Recombinant DNA reagent | Sec61b-APEX2 | This study | Generated by cloning Sec61b-APEX fusion gene (RRID:Addgene_83411) into lentivector | |
Recombinant DNA reagent | APEX2-KDEL | This study | Generated by adding KDEL-encoding sequence to the APEX2 gene and cloning into lentivector | |
Sequence-based reagent | Guide sequence targeting exon 1 of human S1R | This study | GCGCGAAGAG ATAGCGCAGT | |
Sequence-based reagent | Guide sequence targeting LacZ | Platt et al. Cell, 2014 | GTGCGAATAC GCCCACGCGAT | |
Commercial assay or kit | Q5 site-directed mutagenesis kit | NEB | E0554 | |
Chemical compound, drug | 18:1 PC (DOPC) | Avanti Polar Lipids | 850375 | |
Chemical compound, drug | 24:1 PC (DNPC) | Avanti Polar Lipids | 850399 | |
Chemical compound, drug | NBD-PC | Avanti Polar Lipids | 810130 | |
Chemical compound, drug | Cholesterol | Avanti Polar Lipids | 700000 | |
Chemical compound, drug | DSPE-PEG(2000)-biotin | Avanti Polar Lipids | 880129 | |
Chemical compound, drug | DGS-NTA-Ni | Avanti Polar Lipids | 790404 | |
Chemical compound, drug | Egg PC | Avanti Polar Lipids | 241601 | |
Chemical compound, drug | LDAO | Anatrace | D360 | |
Chemical compound, drug | OG | Anatrace | O311 | |
Chemical compound, drug | DDM | Anatrace | D310 | |
Chemical compound, drug | Cholesteryl hemisuccinate | Anatrace | CH210 | |
Chemical compound, drug | CarboxyLink agarose | Thermo Scientific | 20266 | |
Chemical compound, drug | Alexa647 NHS ester | Invitrogen | A37573 | |
Chemical compound, drug | Alexa555 NHS ester | Invitrogen | A37571 | |
Chemical compound, drug | C2 maleimide Alexa647 | Invitrogen | A20347 | |
Chemical compound, drug | (+)-SKF-10047 | Sigma-Aldrich | A114 | |
Chemical compound, drug | NE-100 hydrochloride | Tocris | 3133 | |
Software, algorithm | MATLAB_R2019b | MATLAB | ||
Software, algorithm | GraphPad Prism 8 | GraphPad | ||
Other | Liposomal mini-extruder with 0.1 mkm polycarbonate filters | Avanti Polar Lipids | 610000 |