Miro1-dependent mitochondrial dynamics in parvalbumin interneurons
Figures
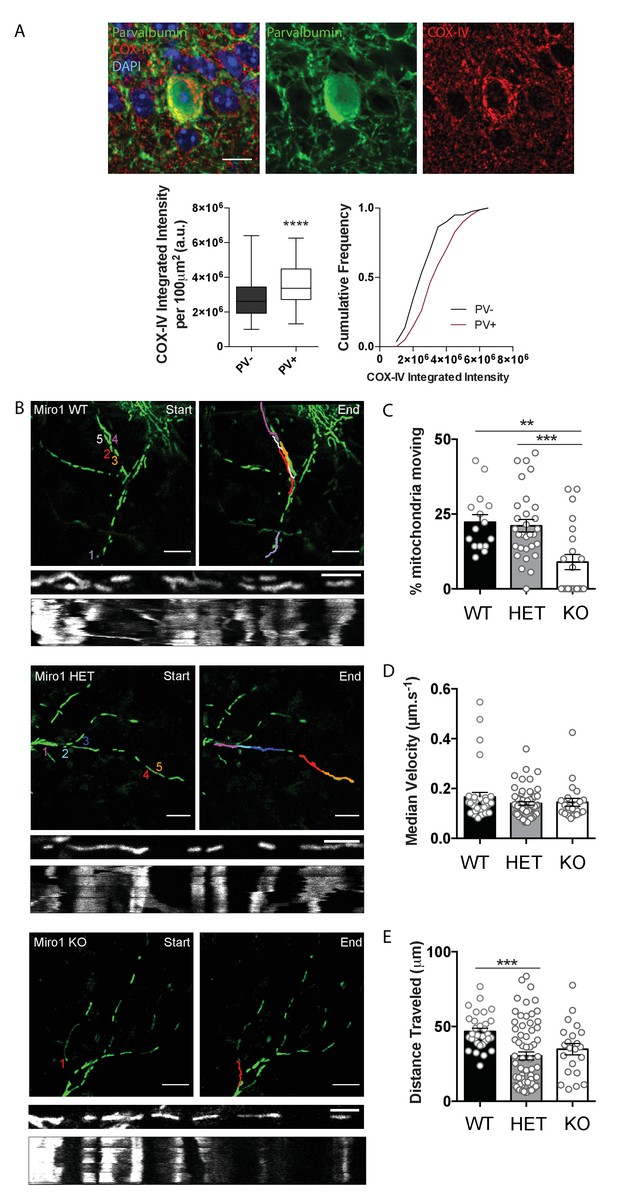
Cell-type specific removal of Miro1 from parvalbumin interneurons impairs mitochondrial trafficking.
(A) COX-IV levels in PV+ interneurons. The fluorescence signal of COX-IV is increased in PV immuno-positive cells in the hippocampus. Scale bar = 10 μm. Boxplot and cumulative distribution show the quantification of the mean integrated intensity of the COX-IV fluorescent signal in PV immuno-positive and -negative regions (n = 81 cells, 11 slices, three animals). (B) Miro1-directed mitochondrial trafficking in organotypic brain slices. Representative images from a 500 s two-photon movie of MitoDendra+ mitochondria in PV+ interneurons in ex vivo organotypic hippocampal slices from WT, ΗΕT, and Miro1 KO animals. The colored numbers/lines denote tracks of individual mobile mitochondria during the movie. Scale bar = 10 μm. The kymographs represent the mitochondrial displacement over time. Scale bar = 5 μm. (C) Quantification of the percentage of moving mitochondria (nWT = 15 movies, nine slices, four animals, nΗΕT = 32 movies, 10 slices, four animals, nKO = 22 movies, eight slices, four animals). (D) Quantification of the median velocity (nWT = 35 mitochondria, nΗΕT = 65 mitochondria, nKO = 22 mitochondria). (E) Quantification of the distance traveled (nWT = 31 mitochondria, nΗΕT = 66 mitochondria, nKO = 22 mitochondria).
-
Figure 1—source data 1
Source data for COX-IV levels and mitochondrial trafficking in PV+ interneurons.
- https://cdn.elifesciences.org/articles/65215/elife-65215-fig1-data1-v2.xlsx
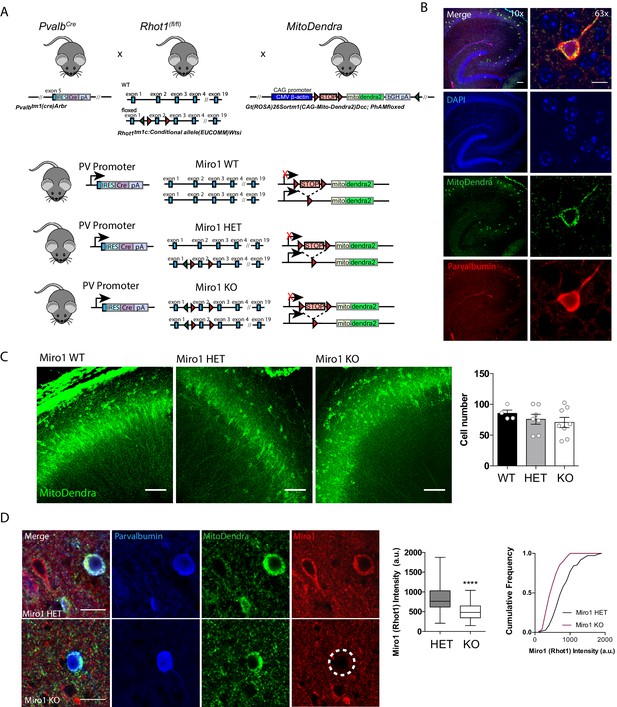
Generation of the PvalbCre Rhot1flox/flox MitoDendra transgenic mouse line and conditional removal of Miro1 from MitoDendra-expressing parvalbumin interneurons.
(A) Schematic diagram of the genetic recombination for the expression of MitoDendra and simultaneous conditional removal of Miro1. When the Cre recombinase is expressed, under the PV+ promoter, the stop-floxed codon in the Rosa26 locus is excised allowing for the downstream expression of MitoDendra. Additionally, the second exon of the Rhot1 gene is found between two loxP sites and therefore removed selectively in PV+ interneurons. (B) Cell-type specific expression of MitoDendra in PV+ interneurons. Left: Representative low magnification (10×) confocal image demonstrating the PV+ interneuron distribution in the acute brain slices of the hippocampus in the PvalbCre MitoDendra mouse. Scale bar = 100 μm. Right: Representative high-magnification (63×) image of a MitoDendra-expressing cell that is also PV immuno-positive. Scale bar = 10 μm. (C) Loss of Miro1 does not affect the viability of MitoDendra-expressing parvalbumin interneurons. Max-projected confocal images from the CA3 area of the hippocampus of WT, ΗΕT and Miro1 KO animals. Scale bar = 100 μm. The bar chart shows the number of MitoDendra+ cells (nWT = 4 slices from two animals, nΗΕT = 7 slices from four animals, nKO = 8 slices from four animals). (D) Loss of Miro1 from PV+ expressing interneurons. Representative confocal image from hemi-floxed (ΗΕT) and conditional knock-out (Miro1 KO) animals. Scale bar = 10 μm. The fluorescent signal for Miro1 is specifically reduced in PV immuno-positive cells (white dotted circle shows the PV immuno-positive cell with low Miro1 fluorescence). The boxplot and cumulative frequency distribution of the Rhot1 (Miro1) fluorescent intensity signal (nΗΕT = 109 neurons, four slices, two animals, nKO = 108 neurons, four slices, two animals).
-
Figure 1—figure supplement 1—source data 1
Source data for cell viability and Miro1 levels.
- https://cdn.elifesciences.org/articles/65215/elife-65215-fig1-figsupp1-data1-v2.xlsx
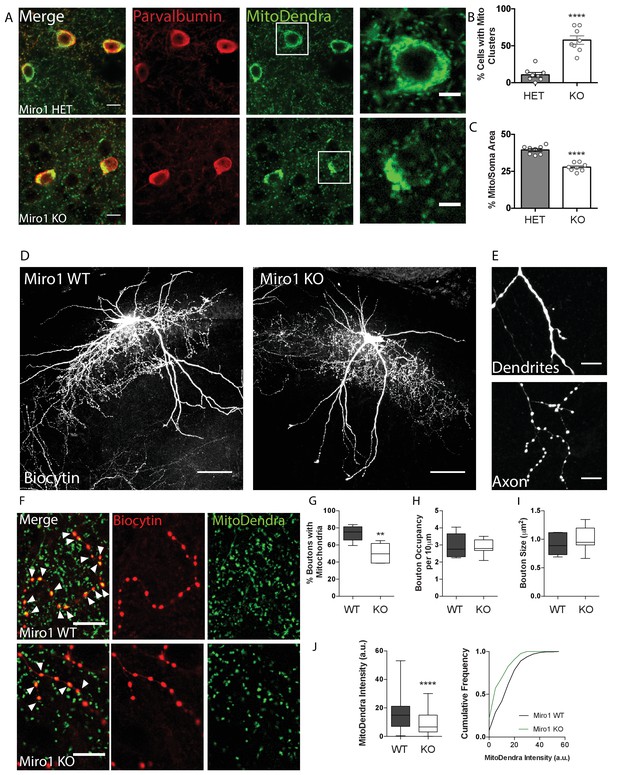
Loss of Miro1 results in an accumulation of mitochondria in the soma and depletion from axonal presynaptic terminals.
(A) Loss of Miro1 results in MitoDendra+ clustering in the somata of PV+ interneurons. Confocal images from ΗΕT and Miro1 KO PV immuno-positive cells in fixed brain tissue. Scale bar = 10 μm. Cells in the white box are zoomed as illustrated in the images on the right, to clearly depict the mitochondrial clusters in the Miro1 KO cells. Scale bar = 5 μm. (B) Bar chart shows the quantification for the percentage of cells that contain mitochondrial clusters (nΗΕT = eight slices, three animals and nKO = eight slices, three animals). (C) Quantification for the percentage area that mitochondria occupy within the PV immuno-positive soma. Due to the presence of mitochondrial clusters the %Mito/Soma area is significantly decreased in the Miro1 KO neurons (nΗΕT = eight slices, three animals and nKO = eight slices, three animals). (D) Representative max-projected confocal stack of biocytin-filled PV+ interneurons in the hippocampus of 350 μm fixed acute brain slices. Scale bar = 100 μm. (E) Example of the distinct distribution of biocytin in dendritic and axonal compartments. Biocytin is diffused in dendrites (top) and clustered in axonal terminals (bottom). (F) Loss of Miro1 depletes mitochondria from axonal presynaptic terminals. Representative images of biocytin-filled synaptic boutons (red) and mitochondria (green) in WT and Miro1 KO neurons. Arrows point to boutons that contain mitochondria. Scale bar = 5 μm. (G) Boxplots for the quantification of the percentage of boutons that contain mitochondria (nWT = five neurons, four slices, two animals and nKO = seven neurons, six slices, three animals). (H) Boxplots for the quantification of the occupancy of boutons in axonal segments (nWT = five neurons, four slices, two animals and nKO = seven neurons, six slices, three animals). (I) Quantification of the mean size of boutons (nWT = 625 boutons, five neurons, four slices, two animals, nKO = 526 boutons, seven neurons, six slices, three animals). (J) Boxplot and cumulative distribution for the quantification of the mean MitoDendra fluorescent intensity within biocytin-filled puncta (nWT = 246 boutons, nKO = 173 boutons).
-
Figure 2—source data 1
Source data for MitoDendra+ mitochondria in the soma and axonal presynaptic terminals of PV+ interneurons.
- https://cdn.elifesciences.org/articles/65215/elife-65215-fig2-data1-v2.xlsx
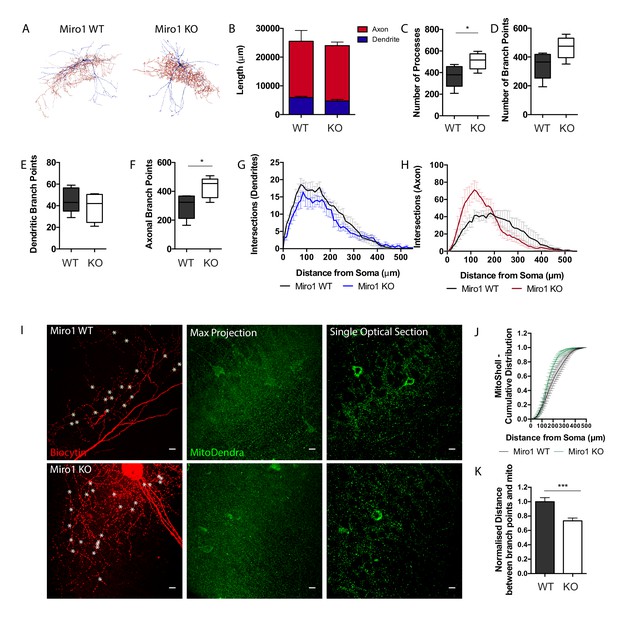
Loss of Miro1 results in increased axonal branching in hippocampal parvalbumin interneurons.
(A) Max-projected reconstruction of the neurons in Figure 2D from Neuromantic software. Dendrites are depicted in blue and axons in red. (B) Quantification of the neuronal length with the dendritic and axonal contribution depicted in blue and red respectively. (C) Boxplot for the quantification of the total number of processes. (D) Boxplot for the quantification of the total number of branch points. (E) Box plot for the quantification of the number of dendritic branch points. (F) Box plot for the quantification of the number of axonal branch points. (G) Number of intersections between dendritic branches and Sholl rings are plotted at distances away from the soma. (H) Number of intersections between axonal branches and Sholl rings are plotted at distances away from the soma. (nWT = five neurons, three slices, two animals, nKO = five neurons, four slices, three animals). (I) Loss of Miro1 results in mitochondria being found closer to points of axonal branching. High-magnification (63×) max-projected confocal stacks of biocytin in a 350 μm hippocampal slice. Analysis was performed in 3D in single optical sections. White stars denote branch points. Scale bar = 10 μm. (J) Cumulative distribution of the MitoDendra+ mitochondrial network (MitoSholl) in individual PV+ interneurons (nWT = five neurons, three slices, two animals, nKO = four neurons, four slices, three animals). (K) Bar graph shows the normalized minimum distance between a branch point and a mitochondrion in the confocal stack (nWT = 122 branch points, five neurons, four slices, two animals and nKO = 171 branch points, seven neurons, six slices, three animals).
-
Figure 3—source data 1
Source data for PV+ interneuron morphology and mitochondrial distribution.
- https://cdn.elifesciences.org/articles/65215/elife-65215-fig3-data1-v2.xlsx
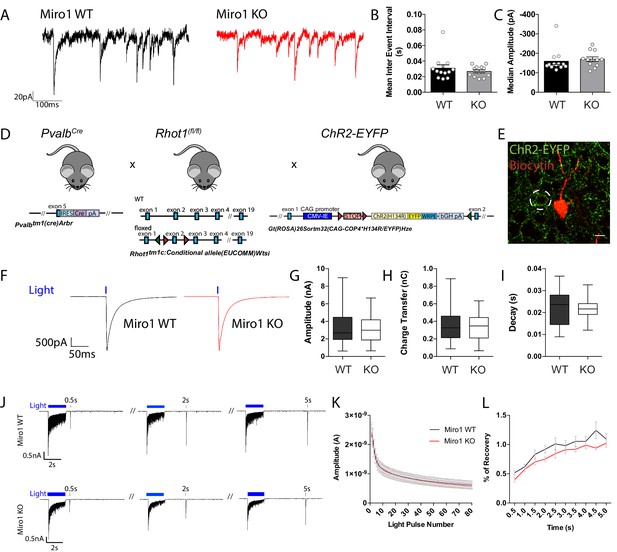
Miro1 knock-out does not alter spontaneous and evoked inhibitory synaptic transmission in the hippocampus.
(A) Representative electrophysiological traces of spontaneous inhibitory post-synaptic currents from WT (black) and Miro1 KO (red) cells in the hippocampus. (B) Quantification for the mean inter event interval (IEI). (C) Quantification for the median sIPSC amplitude. (nWT = 13 recordings, two animals and nKO = 12 recordings, two animals). (D) Generation of the PvalbCre Rhot1 ChR2-EYFP transgenic mouse line. Schematic diagram of the expression of ChR2-EYFP and simultaneous conditional removal of Rhot1. When the Cre recombinase is expressed, under the PV+ promoter, the stop-floxed codon is excised from the Rosa26 locus allowing the downstream expression of ChR2-EYFP. Additionally, the second exon of the Rhot1 gene is found between two loxP sites and also removed selectively in PV+ interneurons. (E) Example of confocal image from a biocytin-filled recorded pyramidal cell in the hippocampus (red) in close proximity to an EYFP+ PV+ interneuron (green). Scale bar = 10 μm. (F) Representative traces from light evoked inhibitory postsynaptic current (eIPSC) in WT (black) and Miro1 KO (red) cells in acute brain slices (nWT = 23 recordings, four animals and nKO = 23 recordings, four animals). (G) Boxplot for the quantification of peak amplitude. (H) Boxplot for the quantification of charge transfer. (I) Boxplot for the quantification of decay. (J) Control and conditional knock-out cells can sustain inhibition and recover with similar rates after long-lasting photostimulation. Example traces from the inhibitory responses pyramidal cells received in WT and Miro1 KO slices during light train stimulation (40 Hz for 2 s; 1 ms pulse width). (K) Mean amplitude of each peak during the light train stimulation (nWT = 21 recordings, four animals and nKO = 24 recordings, four animals). (L) Quantification of the percentage recovery after light stimulation of all cells at increasing time intervals from the end of the light train (nWT = 21 recordings, four animals and nKO = 18 recordings, three animals).
-
Figure 4—source data 1
Source data for PV+ interneuron function.
- https://cdn.elifesciences.org/articles/65215/elife-65215-fig4-data1-v2.xlsx
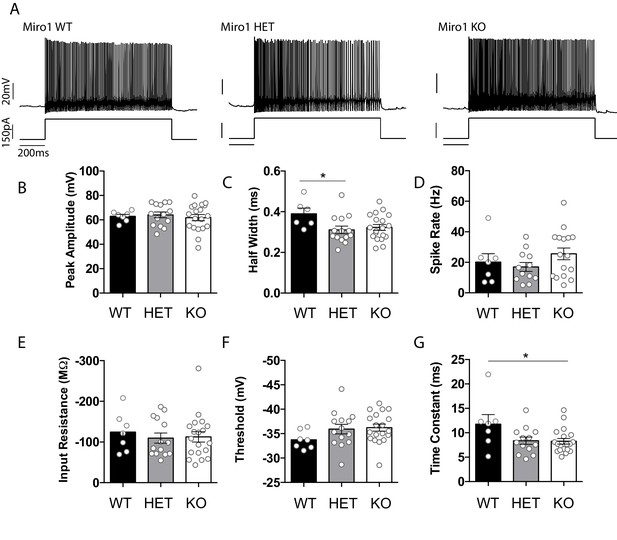
Intrinsic properties of PV+ interneurons in the absence of Miro1.
(A) Representative traces of the firing patterns of WT, ΗΕT, and Miro1 KO cells in response to 200 pA current injection (nWT = two animals, nΗΕT = four animals, nKO = five animals). (B) Quantification of the action potential peak amplitude. (C) Quantification of the action potential half-width. (D) Quantification of Spike Rate at RheoBase +40 pA. Cells that fire less than five spikes were excluded from the quantification. (E) Quantification of input resistance. (F) Quantification of threshold to fire. (G) Quantification of time constant. White circles in bar graphs represent recordings from individual cells.
-
Figure 4—figure supplement 1—source data 1
Source data for PV+ interneuron intrinsic properties.
- https://cdn.elifesciences.org/articles/65215/elife-65215-fig4-figsupp1-data1-v2.xlsx
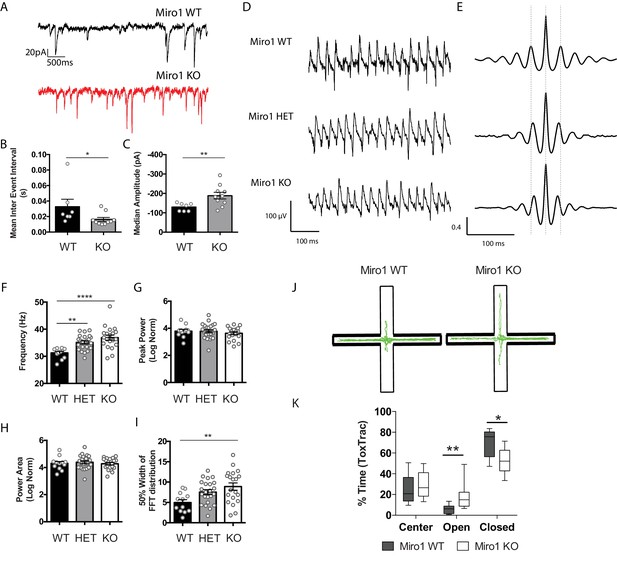
Miro1 knock-out results in altered hippocampal network activity and anxiety-related behavior.
(A) Miro1 KO PV+ interneurons received increased glutamateric input. Representative electrophysiological traces from WT (black) and Miro1 KO (red) cells. (B) Quantification for the mean inter event interval (IEI). (C) Quantification for the median amplitude (nWT = seven recordings, two animals and nKO = 10 recordings, two animals). (D) Loss of Miro1 increases the frequency of γ-oscillations. Representative local field potential recordings from the stratum pyramidale of the CA3 hippocampal area in acute brain slices from WT, ΗΕT, and Miro1 KO mice. (E) Representative auto-correlogram of γ-oscillations from WT, ΗΕT, and Miro1 KO animals. (F) Quantification of the peak frequency. (G) Quantification of the normalized peak power. (H) Quantification of the normalized power area. (I) Quantification of the 50% width dispersion of the FFT distribution (nWT = 12 slices, two animals, nΗΕT = 23 slices, six animals, and nKO = 20 slices, six animals). (J) Assessment of anxiety-related behavior using the elevated plus maze (EPM). Schematic diagram of the EPM and representative ToxTrac trajectories (green) from WT and Miro1 KO animals. (K) Boxplot for the quantification of the percentage of time that WT and Miro1 KO animals spent in the closed, open arms and center of the EPM (nWT = nine animals, nKO = eight animals).
-
Figure 5—source data 1
Source data for excitatory drive, network activity and anxiety-related behavior.
- https://cdn.elifesciences.org/articles/65215/elife-65215-fig5-data1-v2.xlsx
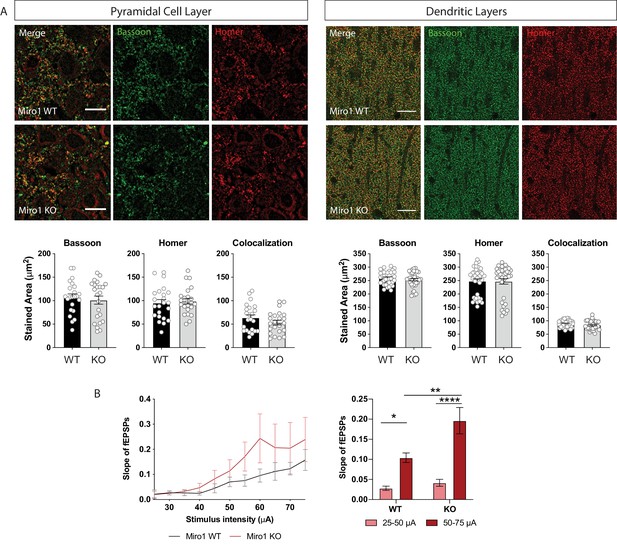
Loss of Miro1 is accompanied by a general increase in excitatory drive in the hippocampus without altering the total levels of excitatory pre- and post- synaptic markers in the hippocampus.
(A) Representative confocal images of the hippocampal pyramidal and dendritic cell layers that were immuno-stained for the presynaptic marker Bassoon, and the postsynaptic marker Homer. Scale bar = 10 μm. The quantification shows the total immunostained area occupied by the markers and their extent of overlap (colocalization). (Pyramidal Cell Layer: nWT = 23 hippocampal strata, 10 slices, three animals, nKO = 23 hippocampal strata, 10 slices, three animals, Dendritic (oriens/radiatum) Cell Layers: nWT = 37 hippocampal strata, 10 slices, three animals, nKO = 36 hippocampal strata, 11 slices, three animals). (B) Input–output relationship for WT and Miro1 KO slope of fEPSP against increasing stimulus intensity. Bar chart shows the slope of fEPSPs at low (25–50 μA) and high (50–75 μA) stimulations (nWT = 7 slices, three animals, nKO = 7 slices, three animals).
-
Figure 5—figure supplement 1—source data 1
Source data for excitation in hippocampus.
- https://cdn.elifesciences.org/articles/65215/elife-65215-fig5-figsupp1-data1-v2.xlsx

Loss of Miro1 does not affect husbandry behavior, motor coordination, short-term memory, and spatial exploration.
(A) Assessment of husbandry behavior based on the amount of shredded and unshredded Nestlet between WT and Miro1 KO animals. Bar chart shows the quantification of the percentage of unshredded Nestlet. Blue points represent values from male mice and pink points represent values from female mice (nWT = nine animals, nKO = eight animals). (B) Assessment of motor coordination using the rotarod. The animal is placed on the revolving rot until it falls and the speed at which it falls is registered to calculate the revolutions per minute (RPM) indicated by the box plot (nWT = five animals, nKO = five animals). (C) Assessment of short-term memory using the spontaneous alternation T-maze behavioral paradigm. The animal starts every trial at position marked as start, the animal is allowed to make a decision at the end of the maze and is kept in that arm for 30 s. A correct entry is considered when the animal makes the opposite decision from the previous trial. A wrong entry is when the animals makes the same decision as in the previous trial. Box plot shows the quantification of the percentage of correct entries. (nWT = eight animals, nKO = seven animals). (D) Assessment of general exploration in an open field. Example trajectories (green) of WT and Miro1 KO animals. (E) Box plot for the quantification of the average velocity. (F) Box plot for the quantification of the distance traveled. (G) Quantification of the percentage time spent at distances away from the centre of the box (nWT = nine animals, nKO = nine animals).
-
Figure 5—figure supplement 2—source data 1
Source data for animal behavior experiments.
- https://cdn.elifesciences.org/articles/65215/elife-65215-fig5-figsupp2-data1-v2.xlsx
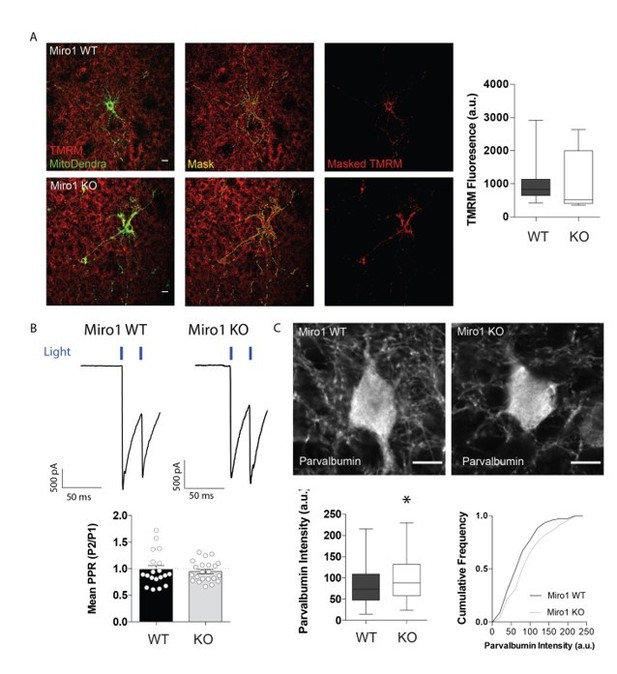
Parvalbumin levels seem to be increased in the Miro1 KO while mitochondrial TMRM fluorescence and synaptic facilitation are unaffected by the absence of Miro1.
(A) Loss of Miro1 does not affect mitochondrial TMRM uptake in parvalbumin interneurons. Representative confocal images from control and cKO organotypic brain slices that were bulk loaded with TMRM. The MitoDendra signal was used as a mask to isolate the TMRM signal emerging from mitochondria in parvalbumin interneurons. Scale Bar = 10 μm. Boxplot shows the quantification for the mean TMRM fluorescence in the cell (nWT = 28 neurons from 6 slices from 3 animals, nKO = 21 neurons from 4 slices from 2 animals). (B) Loss of Miro1 does not alter short-term facilitation. Example responses from control and Miro1 KO cells in the hippocampus. The bar chart shows the quantification for the mean paired-pulse ratio (PPR) of the second response divided by the first response to light and the error bars represent the standard error of the mean (nWT = 19, nKO = 22 recordings from 4 animals). (C) Loss of Miro1 increases parvalbumin levels in the hippocampus. Boxplot and cumulative frequency distribution of the parvalbumin fluorescent intensity signal (nWT = 79 neurons from 10 slices from 3 animals, nKO = 73 neurons from 8 slices from 3 animals).
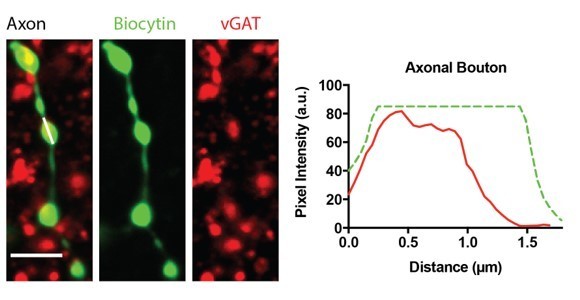
Biocytin-filled boutons on the PV+ interneuron axon represent inhibitory pre-synaptic terminals.
Example max-projected confocal images of biocytin-filled axon. Scale Bar = 3 μm. The graphs represent the fluorescence signal of vGAT (red) from a line-scan (white line) through a biocytin-filled bouton (green).

MitoDendra fluorescence within the biocytin-filled puncta is reduced when Miro1 is knocked-out from PV+ interneurons.
Boxplot and cumulative distribution for the quantification of the mean MitoDendra fluorescent intensity within biocytin-filled puncta (nMiro(+/+) = 246, nMiro(Δ/Δ) = 173 boutons).
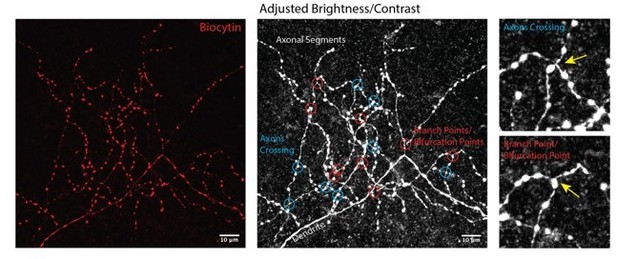
Identification of axonal branch points in biocytin-filled PV+ interneurons.
Adjustment of brightness and contrast allows to distinguish between bifurcation points and points where the axons cross over each other..
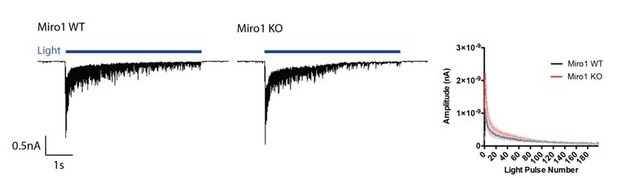
Cells in WT and Miro1 KO mice can sustain inhibition after long-lasting photostimulation.
Example traces from the inhibitory responses pyramidal cells received in WT and Miro1 KO slices during light train stimulation (40 Hz for 5 s; 1 ms pulse width). The quantification shows the mean amplitude of each peak during the light train stimulation (nWT = 4 recordings and nKO = 7 recordings).
Tables
Reagent type (species) or resource | Designation | Source or reference | Identifiers | Additional information |
---|---|---|---|---|
Gene (mouse) | Rhot1 | GenBank | Gene ID: 59040 | |
Strain, strain background (include species and sex here) | Mouse B6;129S−Gt(ROSA)26Sortm1(CAG−COX8A/Dendra2)Dcc/J | The Jackson Laboratory | Stock number 018385 | |
Strain, strain background (include species and sex here) | Mouse: Rhot1tm1a (EUCOMM)Wtsi | Wellcome Trust Sanger Institute | MBTN EPD0066 2 F01 | |
Strain, strain background (include species and sex here) | Mouse: B6;129S−Gt(ROSA)26Sortm32(CAG−COP4*H134R/EYFP)Hze/J | The Jackson Laboratory | Stock number 012569 | |
Biological sample (include species here) | Mouse Organotypic Brain Slices | This Paper | ||
Biological sample (include species here) | Mouse Acute Brain Slices | This Paper | ||
Antibody | Anti-Parvalbumin (mouse monoclonal) | Millipore | Cat# MAB1572 RRID:AB_2174013 | 1:500 |
Antibody | Anti-Rhot1 (Miro1) (rabbit polyclonal) | Atlas | Cat# HPA010687 RRID:AB_1079813 | 1:100 |
Antibody | Anti-COX-IV (rabbit polyclonal) | Abcam | Cat# Ab16056 RRID:AB_443304 | 1:500 |
Antibody | Anti-Bassoon (mouse monoclonal) | Abcam | Cat# ab82958 RRID:AB_1860018 | 1:500 |
Antibody | Homer (rabbit polyclonal) | Synaptic Systems | Cat# 160 002 RRID:AB_2120990 | 1:500 |
Antibody | Donkey Anti-Mouse Alexa Fluor 488 | Jackson ImmunoResearch | Cat# 715-545-151 RRID:AB_2341099 | 1:500-1:1000 |
Antibody | Goat Anti-Rabbit Alexa Fluor 555 | Thermo Fisher Scientific | Cat# A-21430 RRID:AB_2535851 | 1:500-1:1000 |
Antibody | Donkey Anti-Rabbit Alexa Fluor 568 | Thermo Fisher Scientific | Cat# A-10042 RRID:AB_2534017 | 1:500-1:1000 |
Antibody | AffiniPure Fab Fragment Goat Anti-Mouse IgG (H+L) | Jackson ImmunoResearch | Cat# 115-007-003 RRID:AB_2338476 | 50 μg/ml |
Sequence-based reagent | Rhot1 Forward: Rhot1_16_F | This paper, López-Doménech et al., 2016, Sigma-Aldrich | PCR Primers | TTAGGATTTGTACTTTGCCCCTG |
Sequence-based reagent | Rhot1 Reverse: Rhot1_16_R | This paper, López-Doménech et al., 2016, Sigma-Aldrich | PCR Primers | AAAACCCTTCCTGCATCACC |
Sequence-based reagent | Cas | This paper, López-Doménech et al., 2016, Sigma-Aldrich | PCR Primers | TCGTGGTATCGTTATGCGCC |
Sequence-based reagent | MitDend WT Forward | This paper, Sigma-Aldrich | PCR Primers | CCAAAGTCGCTCTGAGTTGTTATC |
Sequence-based reagent | MitDend WT Reverse | This paper, Sigma-Aldrich | PCR Primers | GAGCGGGAGAAATGGATATG |
Sequence-based reagent | MitDend Mut Reverse | This paper, Sigma-Aldrich | PCR Primers | TCAATGGGCGGGGGTCGTT |
Sequence-based reagent | Cre Forward | This paper, Sigma-Aldrich | PCR Primers | CACCCTGTTACGTATAGCCG |
Sequence-based reagent | Cre Reverse | This paper, Sigma-Aldrich | PCR Primers | GAGTCATCCTTAGCGCCGTA |
Sequence-based reagent | LacZ_2_small_F | This paper, López-Doménech et al., 2016, Sigma-Aldrich | PCR Primers | ATCACGACGCGCTGTATC |
Sequence-based reagent | LacZ_2_small_R | This paper, López-Doménech et al., 2016, Sigma-Aldrich | PCR Primers | ACATCGGGCAAATAATATCG |
Sequence-based reagent | ChR Forward | This paper, Sigma-Aldrich | PCR Primers | AAGGGAGCTGCAGTGGAGTA |
Sequence-based reagent | ChR Reverse | This paper, Sigma-Aldrich | PCR Primers | CCGAAAATCTGTGGGAAGTC |
Sequence-based reagent | ChR mut Forward | This paper, Sigma-Aldrich | PCR Primers | ACATGGTCCTGCTGGAGTTC |
Sequence-based reagent | ChR mut Reverse | This paper, Sigma-Aldrich | PCR Primers | GGCATTAAAGCAGCGTATCC |
Peptide, recombinant protein | Streptavidin conjugated to Alexa Fluor 555 | Invitrogen | S32355 | 1:500 |
Chemical compound, drug | Carbachol | Sigma Aldrich | 51.83.2 | [5 μM] |
Chemical compound, drug | Biocytin | Sigma Aldrich | B4261 | [3–4 mg/ml] |
Software, algorithm | Fiji | Schindelin et al., 2012 | https://www.fiji.sc/ | |
Software, algorithm | Simple Neurite Tracer | Longair et al., 2011 | https://imagej.net/SNT | |
Software, algorithm | Neuromantic | Myatt et al., 2012 | https://www.reading.ac.uk/neuromantic/body_index.php | |
Software, algorithm | ToxTrac | Rodriguez et al., 2018 | https://sourceforge.net/projects/toxtrac/ | |
Software, algorithm | Matlab_R2015a | Mathworks | https://www.mathworks.com/products/matlab.html | |
Software, algorithm | Prism 6 | Graphpad | https://www.graphpad.com/scientific-software/prism/ | |
Software, algorithm | IgorPro 6.3 | Wavemetrics | https://www.wavemetrics.com/ | |
Other | DAKO mounting media | Agilent Technologies | S3023 | |
Other | Omnipore membrane inserts | Millipore | Cat no. FHLC01300 |
Sequencing primers.
Genotyping primer | Sequence (5’ to 3’) |
---|---|
Rhot1 forward: Rhot1_16_F | TTAGGATTTGTACTTTGCCCCTG |
Rhot1 reverse: Rhot1_16_R | AAAACCCTTCCTGCATCACC |
Cas | TCGTGGTATCGTTATGCGCC |
MitDend WT forward | CCAAAGTCGCTCTGAGTTGTTATC |
MitDend WT reverse | GAGCGGGAGAAATGGATATG |
MitDend Mut reverse | TCAATGGGCGGGGGTCGTT |
Cre forward | CACCCTGTTACGTATAGCCG |
Cre reverse | GAGTCATCCTTAGCGCCGTA |
LacZ_2_small_F | ATCACGACGCGCTGTATC |
LacZ_2_small_R | ACATCGGGCAAATAATATCG |
ChR forward | AAGGGAGCTGCAGTGGAGTA |
ChR reverse | CCGAAAATCTGTGGGAAGTC |
ChR mut forward | ACATGGTCCTGCTGGAGTTC |
ChR mut reverse | GGCATTAAAGCAGCGTATCC |
Additional files
-
Source code 1
MitoSholl Matlab Script.
- https://cdn.elifesciences.org/articles/65215/elife-65215-code1-v2.zip
-
Transparent reporting form
- https://cdn.elifesciences.org/articles/65215/elife-65215-transrepform-v2.docx