Structural basis of Stu2 recruitment to yeast kinetochores
Figures
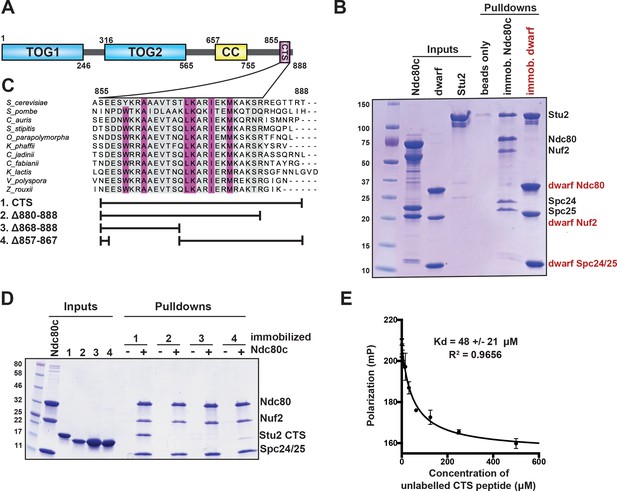
Binding of Stu2 C-terminal segment (CTS) with Ndc80c.
(A) Schematic representation of the domain structure of Stu2, showing the tubulin-binding TOG domains, the dimeric coiled-coil, and the CTS. Molecular mass markers shown indicate molecular weight in kDa. (B) Association of full-length Ndc80c and Stu2. Dwarf and full-length Ndc80c were immobilized to saturation on Ni-NTA agarose and incubated with Stu2. After extensive washing, bound proteins were eluted with buffer containing 400 mM imidazole. (C) Multiple sequence alignment showing conservation of the CTS among budding and fission yeasts. Conservation calculated using T-Coffee Server (gray boxes) and percent identity calculated using Clustal Omega (purple boxes). The bars below the alignment correspond to the Saccharomyces cerevisiae sequence in the alignment and show the constructs used in the pulldown experiments in (D). The blank parts of each line represent deletions. (D) Binding of Ndc80cdwarf and Stu2 CTS. The Stu2 constructs used in this experiment consist of the Stu2 coiled-coil domain, followed by a glycine-serine linker, followed by the regions of the CTS indicated by the bars in (C). Ndc80cdwarf was immobilized on Co-NTA agarose and incubated with Stu2. After extensive washing, bound proteins were eluted with buffer containing 400 mM imidazole. (E) Affinity of Ndc80cdwarf and Stu2 CTS. Competition fluorescence polarization, showing displacement of Oregon-green labeled CTS peptide (50 nM) from Ndc80cdwarf (10 µM) with increasing concentrations of an unlabeled CTS peptide. Polarization (in milliP) is plotted against concentration of unlabeled peptide. Data fitted with a single-site saturation binding model implemented in GraphPad Prism 9.
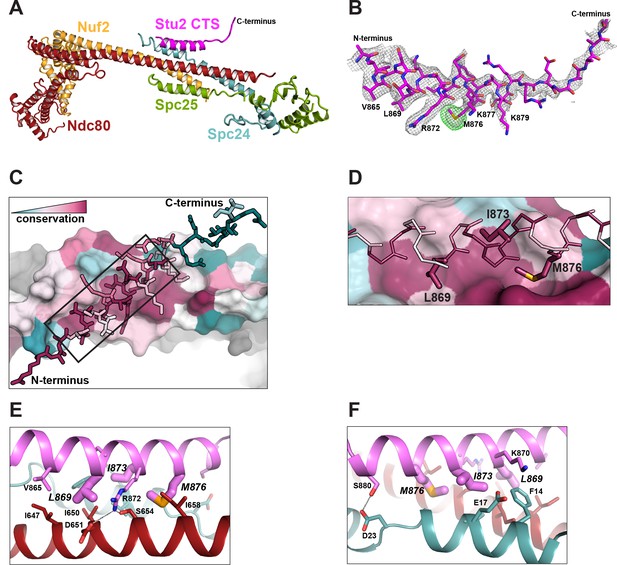
Structure of the Stu2 C-terminal segment (CTS) bound to the Ndc80cdwarf.
(A) Stu2 CTS (residues 855–888) bound to the Ndc80cdwarf. The peptide binds at the four-way junction of Ndc80, Nuf2, Spc24, and Spc25 in a groove between Ndc80 and Spc24. (B) Model of the Stu2 peptide built into the 2Fo-Fc map (gray mesh); anomalous difference map (green mesh), contoured at 8σ, showing the position of SeMet in the peptide. (C) Conservation of residues at the contacts of Stu2 CTS, Ncd80, and Spc24, shaded from red (conserved) to blue (variable). Nuf2 and Spc25 are in gray. Ndc80c components in surface representation; Su2 in stick representation. The N- and C-termini of the Stu2 peptide are indicated. The expanded region depicted in (D) is shown as a black box. (D) Expanded view of the Ndc80-Spc24 surface corresponding to the boxed region in panel C, showing conserved pockets for the three hydrophobic residues of Stu2 discussed in the text. Coloring as in (C). (E and F) Detailed views of the contacts between the Stu2 peptide and Ndc80 and Spc24.
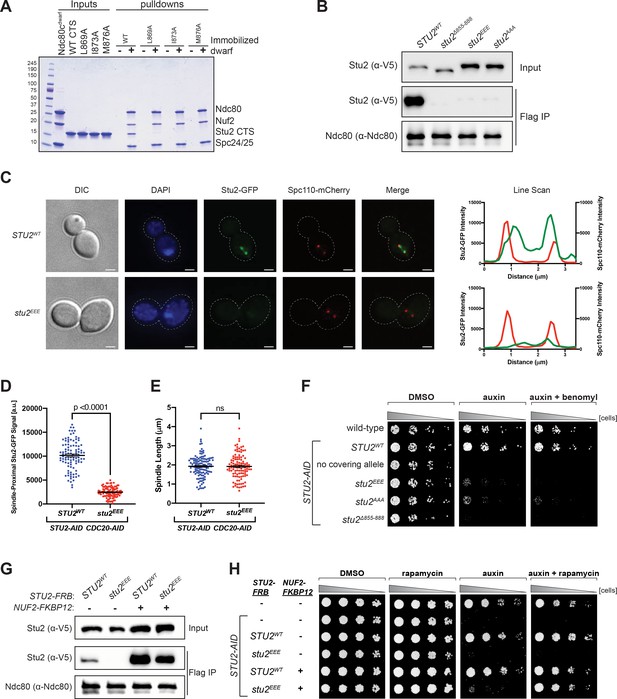
Effects of Stu2 mutations at the interface with Ndc80c on kinetochore association and cell viability and their rescue by re-tethering.
(A) Effect of mutations at the binding interface. The Stu2 constructs contain the Stu2 coiled-coil domain, a glycine-serine linker, and either native or mutated C-terminal segment (CTS). Ndc80cdwarf was immobilized on Co-NTA agarose, incubated with Stu2, washed, and eluted with 400 mM imidazole. (B) Exponentially growing STU2-AID cultures expressing an ectopic copy of STU2 (STU2WT, M622; stu2Δ855-888, M653; stu2L869E,I873E,M876E or stu2EEE, M1444; stu2L869A,I873A,M876A or stu2AAA, M1576) and also expressing from the genomic locus DSN1-6His-3Flag were treated with auxin 30 min prior to harvesting. Kinetochore particles were purified from lysates by anti-Flag immunoprecipitation (IP) and analyzed by immunoblotting. (C) Exponentially growing STU2-AID CDC20-AID cultures with an ectopically expressed STU2-GFP allele (STU2WT-GFP, M1757; stu2EEE-GFP, M1985) that also contained SPC110-mCherry (spindle pole) were treated with auxin for 2.5 hr to arrest cells in metaphase. Left: Representative micrographs for each are shown. White bars represent 2 μm. Right: Line scan plots through spindle axis (encompassing maximum Stu2-GFP signal) show Stu2-GFP and Spc110-mCherry intensity from example cells shown on left. (D) Spindle-Proximal Stu2-GFP signal from cells (n = 100) and line scan plots described in (C). Area under the curve for each line scan was measured using Fiji (p-value from a two-tailed unpaired t-test). (E) Spindle length (distance between Spc110-mCherry foci) was measured for cells (n = 100) described in (C) (p-value from a two-tailed unpaired t-test; n.s. = not significant). (F) Wild-type (M3), STU2-AID (no covering allele, M619), and STU2-AID cells expressing various STU2-3V5 alleles from an ectopic locus (STU2WT, M622; stu2EEE, M1444; stu2AAA, M1576; stu2Δ855-888, M653) were serially diluted (fivefold) and spotted on plates containing DMSO, 500 μM auxin, or 500 μM auxin + 5 μg/mL benomyl. (G) Exponentially growing STU2-AID fpr1Δ TOR1-1 cultures expressing an ectopic copy of STU2-FRB with wild-type NUF2 (STU2WT, M1513; stu2EEE, M1515) or with NUF2-FKBP12 (STU2WT, M1505; stu2EEE, M1507) were treated with rapamycin and auxin 30 min prior to harvesting. Protein lysates prepared, subjected to α-Flag IP, and analyzed as in (B). (H) fpr1Δ TOR1-1 cells (M1375), STU2-AID fpr1Δ TOR1-1 cells (no covering allele, M1476), and STU2-AID fpr1Δ TOR1-1 cells expressing STU2-FRB alleles at an ectopic locus with and without NUF2-FKBP12 (M1513; M1515; M1505; M1507 – same genotypes as in D) were serially diluted (fivefold) and spotted on benomyl plates containing DMSO, 50 ng/mL rapamycin, 500 μM auxin, or 500 μM auxin + 50 ng/mL rapamycin.
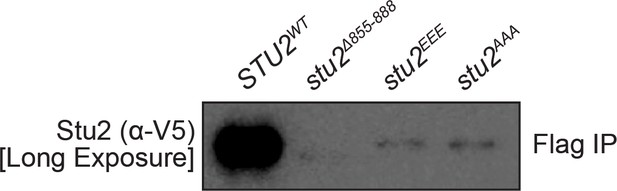
Reproduction of western blot from Figure 3B also showing immunoblot signal for Stu2-3V5 with longer exposure included.
Note: small residual binding of Stu2EEE protein to purified kinetochore particles may explain observed difference between stu2EEE and no covering allele in percent biorientation shown in Figure 4B.
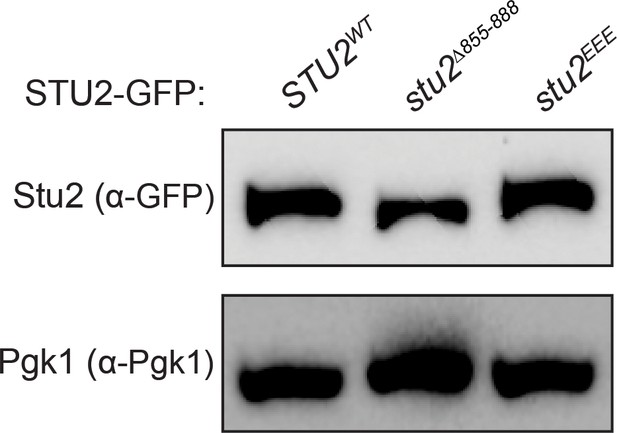
Stu2-GFP expression levels examined by immunoblotting.
Exponentially growing cultures used in Figure 3C,D that contained an ectopically expressed STU2-GFP allele (STU2WT-GFP, M1757; stu2EEE-GFP, M1985; stu2∆855-888-GFP, M1761) were harvested to examine Stu2-GFP expression levels and examined by immunoblotting. Pgk1 is shown as a loading control.
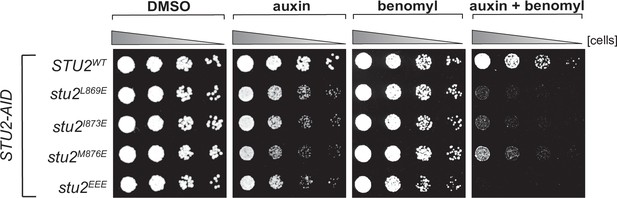
Cell viability assays for single residue hydrophobic-to-charged mutations.
STU2-AID cells expressing various STU2-3V5 alleles from an ectopic locus (STU2WT, M622; stu2L869E, M1443; stu2I873E, M1442; stu2M876E, M1441; stu2EEE, M1444) were serially diluted (fivefold) and spotted on plates containing DMSO, 500 μM auxin, 5 μg/mL benomyl or 500 μM auxin + 5 μg/mL benomyl.
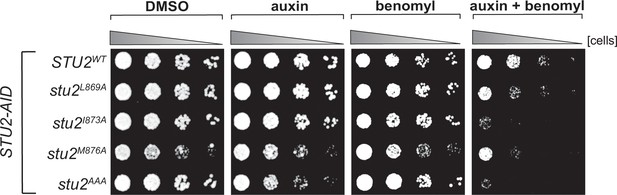
Cell viability assays for single residue hydrophobic-to-alanine mutations.
STU2-AID cells expressing various STU2-3V5 alleles from an ectopic locus (STU2WT, M622; stu2L869A, M1576; stu2I873A, M1575; stu2M876A, M1574; stu2AAA, M1525) were serially diluted (fivefold) and spotted on plates containing DMSO, 500 μM auxin, 5 μg/mL benomyl, or 500 μM auxin + 5 μg/mL benomyl.
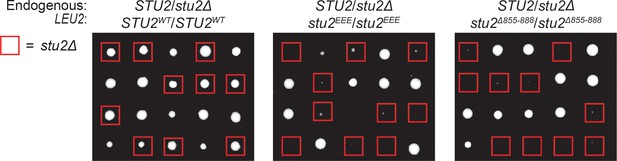
Heterozygous diploid dissection of different stu2 mutant alleles.
Diploid cells heterozygous for STU2/stu2∆::HIS3 at the endogenous STU2 locus and homozygous for different STU2 alleles integrated at the LEU2 locus (STU2WT/STU2WT, M1718; stu2EEE/stu2EEE, M1714; stu2∆855-888/ stu2∆855-888, M1716) were sporulated and dissected on YPD plates. Spores scored positively for HIS3 are boxed in red. Spores harboring both deletion of endogenous STU2 and either stu2EEE or stu2∆855-888 have a viability defect. The stu2EEE strain shows a dominant meiotic phenotype in this assay as well, possibly resulting from aberrant meiotic chromosome segregation in STU2/stu2∆:HIS3 stu2EEE/stu2EEE diploid cells.
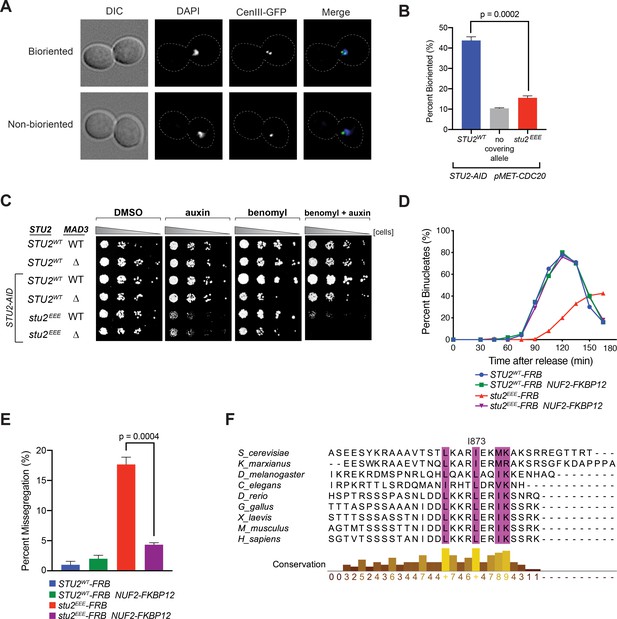
Cellular phenotypes of stu2EEE and conservation of key residues in multicellular eukaryotes.
(A) Exponentially growing STU2-AID pMET-CDC20 cultures with an ectopically expressed STU2 allele (STU2WT, M1154; stu2EEE, M1610) or no ectopic allele (no covering allele, M1153) and also containing CEN3 marked with GFP (CEN3::lacO LacI-GFP) were arrested in methionine + auxin containing media for 2.5 hr. Representative micrographs for bioriented and non-bioriented cells shown. (B) Percent bioriented cells were measured for cultures described in (A). Three replicates of n = 200 cells shown; p-value determined with unpaired t-test. (C) Wild-type cells (M3), cells with spindle checkpoint mutation (mad3Δ, M36), and STU2-AID cells expressing an ectopic copy of STU2 without and with a spindle checkpoint mutation (STU2WT, M622; STU2WT mad3Δ, M1622; stu2EEE, M1444; stu2EEE mad3Δ, M1541) were serially diluted (fivefold) and spotted on plates containing DMSO, 500 μM auxin, 5 μg/mL benomyl, or 500 μM auxin + 5 μg/mL benomyl. (D) STU2-AID cells expressing STU2-FRB alleles at an ectopic locus with NUF2 or NUF2-FKBP12 (STU2WT-FRB, M1513; stu2EEE-FRB, M1515; STU2WT-FRB NUF2-FKBP12, M1505; stu2EEE-FRB NUF2-FKBP12, M1507) were released from a G1 arrest into auxin and rapamycin containing media. Cell cycle progression determined by the accumulation of binucleate cells. (E) STU2-AID mad3∆ cells expressing STU2-FRB alleles at an ectopic locus with NUF2 or NUF2-FKBP12 (STU2WT-FRB, M2025; stu2EEE-FRB, M2024; STU2WT-FRB NUF2-FKBP12, M2027; stu2EEE-FRB NUF2-FKBP12, M2026) that also contained a fluorescently labeled centromere of chromosome III were released from G1 arrest into auxin- and rapamycin-containing medium. Quantification of chromosome mis-segregation in anaphase (percent of binucleate cells with a fluorescently labeled chromosome III signal in only one of the two nuclei). Shown is an average of three biological replicates, n = 100 cells each. (F) Multiple sequence alignment of the Stu2 C-terminus and C-termini from Stu2 eukaryotic homologs. Histogram shows conservation score generated with Clustal Omega. Highly conserved residues, including hydrophobic amino acids important for Ndc80c binding, are boxed in purple.

Schematic illustrating re-tethering of Stu2EEE to Ndc80c.
In the presence of rapamycin, Stu2EEE-FRB binds to Nuf2-FKBP12, restoring Stu2EEE-Ndc80c interaction.
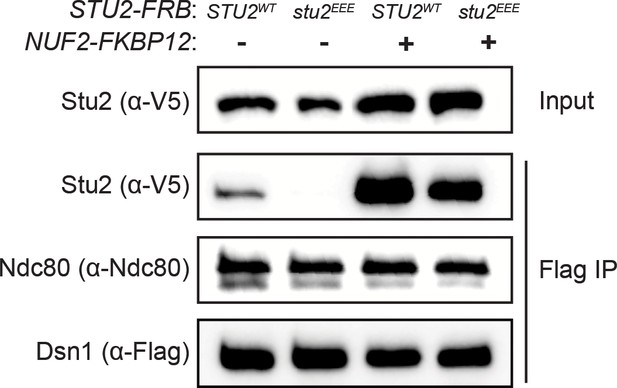
Reproduction of western blot from Figure 3D also showing immunoblot signal for Dsn1-6His-3Flag.
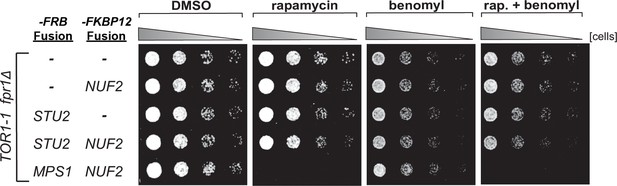
Characterization of STU2-FRB and NUF2-FKBP12 fusion alleles.
fpr1Δ TOR1-1 cells (M1375), and fpr1Δ TOR1-1 cells expressing -FRB and/or -FKBP12 fusion alleles at the endogenous fusion-target locus (NUF2-FKBP12, M1422; STU2-FRB, M1387; STU2-FRB NUF2-FKBP12, M1428; MPS1-FRB NUF2-FKBP12, M1461) were serially diluted (fivefold) and spotted on plates containing DMSO, 50 ng/mL rapamycin, 3.5 µg/mL benomyl, or 3.5 µg/mL benomyl + 50 ng/mL rapamycin. Note: tethering Stu2-FRB to Nuf2-FKBP12 does not affect cell viability, whereas tethering Mps1-FRB results in lethality, as previously reported (Aravamudhan et al., 2015).
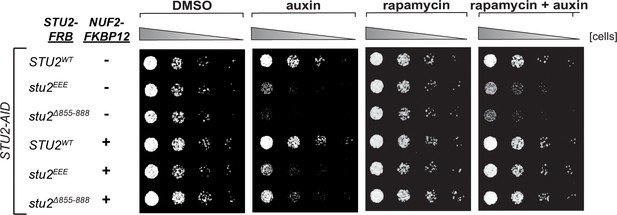
Tethering Stu2∆855-888-FRB to Ndc80c restores viability to the same degree as does tethering Stu2EEE-FRB.
STU2-AID fpr1Δ TOR1-1 cells expressing STU2-FRB alleles at an ectopic locus with and without NUF2-FKBP12 (STU2WT-FRB, M1513; stu2EEE-FRB, M1515; stu2∆855-888-FRB, M1587; STU2WT-FRB NUF2-FKBP12, M1505; stu2EEE-FRB NUF2-FKBP12, M1507; stu2∆855-888-FRB NUF2-FKBP12, M1554) were serially diluted (fivefold) and spotted on YPD plates containing DMSO, 50 ng/mL rapamycin, 500 μM auxin, or 500 μM auxin + 50 ng/mL rapamycin. Tethering Stu2∆855-888-FRB to Ndc80c restores viability to the same degree as does tethering Stu2EEE-FRB. Thus the essential function of the Stu2 C-terminal segment (CTS) is kinetochore binding. These data also imply that the kinetochore function of Stu2 does not require its association with other factors that bind the CTS.
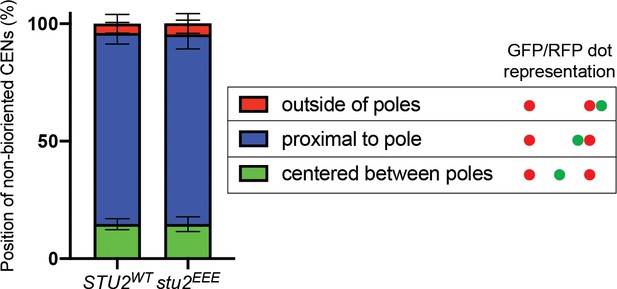
Examining the axial position of unseparated sister centromeres along the length of the mitotic spindle.
Exponentially growing STU2-AID pMET-CDC20 cultures with an ectopically expressed STU2 allele (STU2WT, M2029; stu2EEE, M2030) and also containing SPC110-mCherry and CEN3 marked with GFP (CEN3::lacO LacI-GFP) were arrested in methionine- and auxin-containing medium for 2.5 hr and the axial position of unseparated sister centromeres along the length of the mitotic spindle (near spindle equator, proximal to one Spc110-mCherry foci, or outside of Spc110-mCherry foci) was determined. Schematic shows representative sister centromere positions illustrated as GFP/RFP dots. Graphs represent an average of three biological replicates, n = 50 cells each.
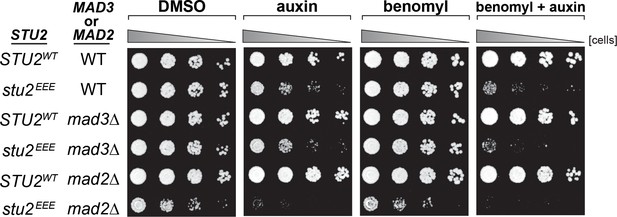
Synthetic growth defects of stu2EEE with mad2∆ and mad3∆.
Cells with spindle checkpoint mutation (mad3Δ, M36; mad2Δ, M35) and STU2-AID cells expressing an ectopic copy of STU2 without and with a spindle checkpoint mutation (STU2WT, M622; stu2EEE, M1444; stu2EEE mad3Δ, M1541; stu2EEE mad2Δ, M1933) were serially diluted (fivefold) and spotted on plates containing DMSO, 500 μM auxin, 5 μg/mL benomyl, or 500 μM auxin + 5 μg/mL benomyl.
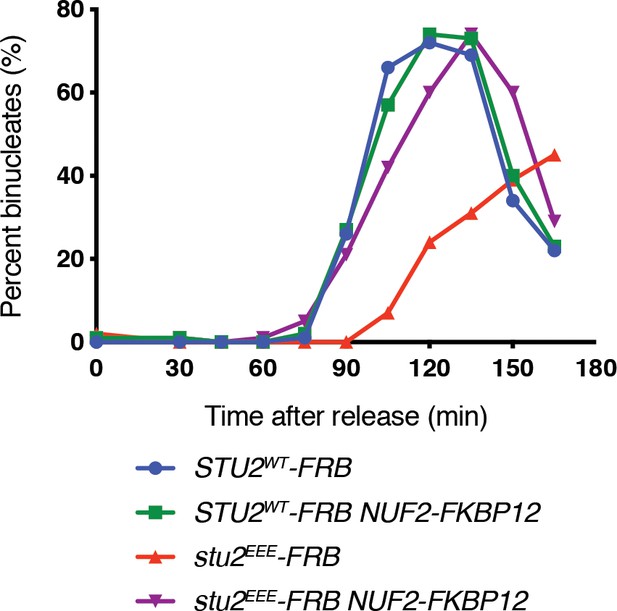
Replicate cell cycle delay experiment as described in Figure 4D.
Additional files
-
Supplementary file 1
Strains used in this study.
All strains are derivatives of M3 (W303).
- https://cdn.elifesciences.org/articles/65389/elife-65389-supp1-v2.docx
-
Supplementary file 2
Plasmids and primers used in this study.
All pM plasmids (except pM646) are derivatives of pM225.
- https://cdn.elifesciences.org/articles/65389/elife-65389-supp2-v2.xlsx
-
Supplementary file 3
Data collection and refinement statistics.
- https://cdn.elifesciences.org/articles/65389/elife-65389-supp3-v2.docx
-
Transparent reporting form
- https://cdn.elifesciences.org/articles/65389/elife-65389-transrepform-v2.pdf