Mechanisms underlying neonate-specific metabolic effects of volatile anesthetics
Figures
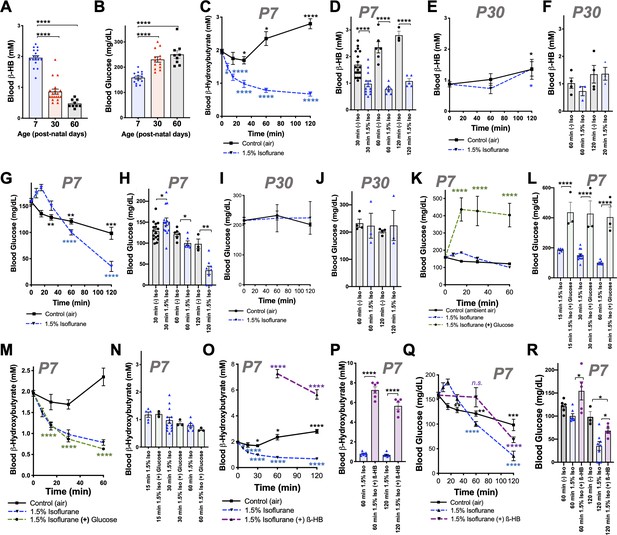
Isoflurane exposure disrupts circulating glucose and beta-hydroxybutyrate in neonatal mice.
(A) Blood β-hydroxybutyrate (β-HB) concentration in neonatal post-natal day 7 (P7), adolescent post-natal day 30 (P30), and young adult post-natal day 60 (P60) mice. n = 17, 19, and 9, respectively. ****p<0.0001 by pairwise t-test. ANOVA ****p<0.0001. (B) Blood glucose in P7, P30, and P60 mice. n = 14, 13, and 9, respectively. ****p<0.0001 by pairwise t-test. ANOVA ****p<0.0001. (C, D) Blood β-HB in P7 neonatal mice exposed to 1.5% isoflurane or control conditions for 0 to 120 min. (C) Pairwise comparisons shown between baseline (time = 0) and respective treatment timepoints. *p≤0.05, ****p<0.0001 by two-tailed pairwise t-test. (D) Bar graphs with individual datapoints for pairwise comparisons between treatments at 30, 60, and 120 min. ****p<0.0001 by two-tailed pairwise t-test. (E, F) Blood β-HB in P30 adolescent mice exposed to 1.5% isoflurane or control conditions for 0–120 min. (E) Control and isoflurane-exposed groups both show a modest but significant increase in β-HB over baseline by 2 hr of exposure, *p≤0.05. (F) Isoflurane exposure did not significantly alter β-HB levels relative to time-matched control conditions. (G–H) Blood β-HB in P7 neonatal mice exposed to 1.5% isoflurane or control conditions for 0–120 min. (G) Pairwise comparisons shown between baseline (time = 0) and respective treatment timepoints. *p≤0.05, **p<0.005, ***p<0.0005, ****p<0.0001 by two-tailed pairwise t-test. (H) Bar graphs with individual datapoints for pairwise comparisons between treatments at 30, 60, and 120 min. *p≤0.05, **p<0.005. (I, J) Blood glucose levels in P30 mice exposed to control conditions or 1.5% isoflurane anesthesia for up to 120 min. (I) Blood glucose levels did not significantly change compared to baseline. (J) Bar graphs of data in (I) with individual datapoints for pairwise comparison of blood glucose levels by treatment. No significant changes observed. (K, L) Blood glucose in P7 mice provided glucose by IP injection at the start of anesthetic exposure plotted as a function of time. (K) Control-exposed and 1.5% isoflurane- exposed data from (G) shown for reference. Pairwise comparisons shown between baseline (T = 0) values and 15, 30, and 60 min timepoints in 1.5% isoflurane (+) glucose treatment group, ****p<0.0001 by pairwise t-test. (L) Bar graphs of (K) with individual datapoints for pairwise comparisons of blood glucose in mice exposed to 1.5% isoflurane or 1.5% isoflurane (+) glucose. ****p<0.0001 by two-tailed pairwise t-test. (M, N) Blood β-HB levels in mice provided glucose by IP injection at the start of anesthetic exposure, plotted as a function of time. (M) Control-exposed and 1.5% isoflurane-exposed data from (C) shown for reference. Pairwise comparisons shown between baseline (T = 0) and 15, 30, and 60 min timepoints in 1.5% isoflurane (+) glucose treatment group, ****p<0.0001. (N) Bar graphs of (M) with individual datapoints for pairwise comparisons of blood β-HB in mice exposed to 1.5% isoflurane or 1.5% isoflurane (+) glucose. Glucose administration did not attenuate the loss of β-HB in response to isoflurane exposure. (O, P) Blood β-HB levels in mice provided β-HB by IP injection at the start of anesthetic exposure. (O) Control-exposed and 1.5% isoflurane-exposed data from (C) shown for reference. Pairwise comparisons shown between baseline (T = 0) and 60 and 120 min timepoints in 1.5% isoflurane (+) β-HB treatment group, ****p<0.0001. (P) Bar graphs of (O) with individual datapoints for pairwise comparisons of blood β-HB in mice exposed to 1.5% isoflurane or 1.5% isoflurane (+) glucose. ****p<0.0001. (Q, R) Blood glucose levels in mice provided β-HB by IP injection at the start of anesthetic exposure, plotted as a function of time. (Q) Control -exposed and 1.5% isoflurane-exposed data from (G) shown for reference. Pairwise comparisons shown between baseline (T = 0) and 60 and 120 min timepoints in 1.5% isoflurane (+) β-HB treatment group, ****p<0.0001, n.s. – not significant. (R) Bar graphs of (Q) with individual datapoints for pairwise comparisons of blood β-HB in mice exposed to 1.5% isoflurane or 1.5% isoflurane (+) glucose or baseline. *p<0.05. For all data, error bars represent standard error of the mean (SEM). ANOVA p-value for 1 hr dataset *p=0.0083; ANOVA for 2 hr dataset **p=0.0033. (A–R) For all data, n ≥ 3 per time/treatment. Each datapoint in bar graphs represents an individual animal. See Materials and methods for additional details.
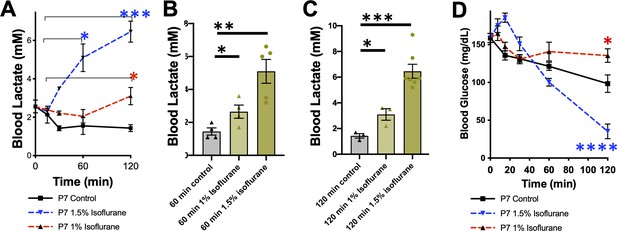
Dose-dependent effects of 1% and 1.5% isoflurane on blood lactate and glucose in P7 mice.
(A) Blood lactate as a function of time in mice exposed to control conditions in either air or 100% oxygen for up to 2 hr. (B) Bar graphs of 60 min data from (A). One-way ANOVA *p<0.05. *p<0.05 by pairwise t-test. (C) Bar graphs of 120 min data from (A). (D) Whole-blood β-HB in P7 neonatal mice exposed to 1.5%, 1% isoflurane, or control conditions for 0 to 120 min. (A, D) *p≤0.05, **p<0.005, ***p<0.0005, ****p<0.0001 by two-tailed pairwise t-test versus baseline (time = 0). (B, C) One-way ANOVA ***p=0.0001, *p<0.05 and ***p<0.0005 by pairwise t-test.
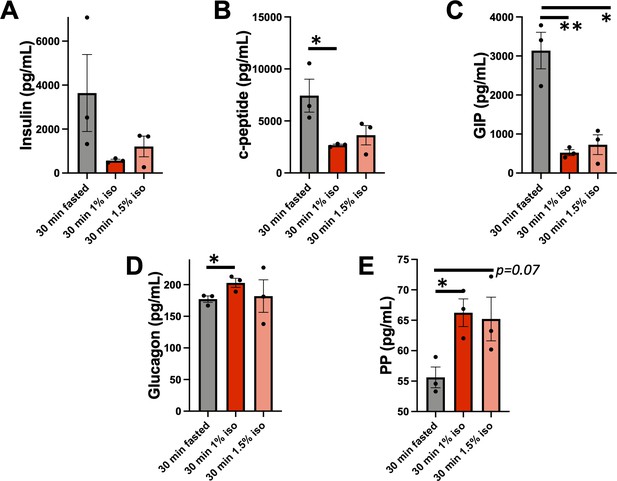
Circulating nutrient factors.
Brief exposure to isoflurane results in significant changes to glucoregulatory factors, but directionality is inconsistent with a role in driving the metabolic effects reported here. (A) Insulin, the major circulating factor responsible for glucose uptake by tissue; (B) c-peptide, a peptide released as a byproduct of insulin production; (C) gastric inhibitory polypeptide (GIP), a factor involved in stimulating insulin secretion; (D) glucagon, a key hypoglycemia response factor which drives glucose release and gluconeogenesis; (E) pancreatic polypeptide (PP), a pancreatic secretion regulator induced by acute hypoglycemia or fasting and decreased by high levels of glucose. *p≤0.05, **p≤0.005 by two-tailed pairwise t-test.
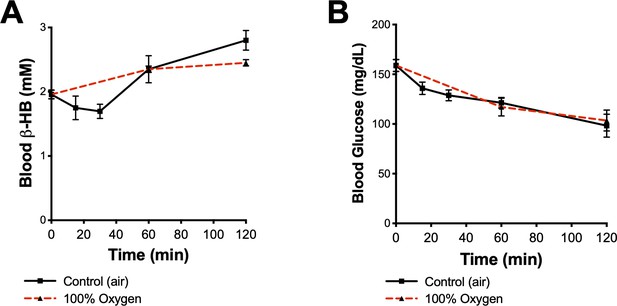
Oxygen concentration does not impact circulating glucose or β-HB.
(A) Blood β-HB as a function of time in P7 mice exposed to control conditions in either air or 100% oxygen for up to 2 hr. No significant differences were observed at any timepoint. (B) Blood glucose as a function of time in mice exposed to control conditions in either air or 100% oxygen for up to 2 hr. No significant differences were observed at any timepoint. N ≥ 3 at each timepoint.
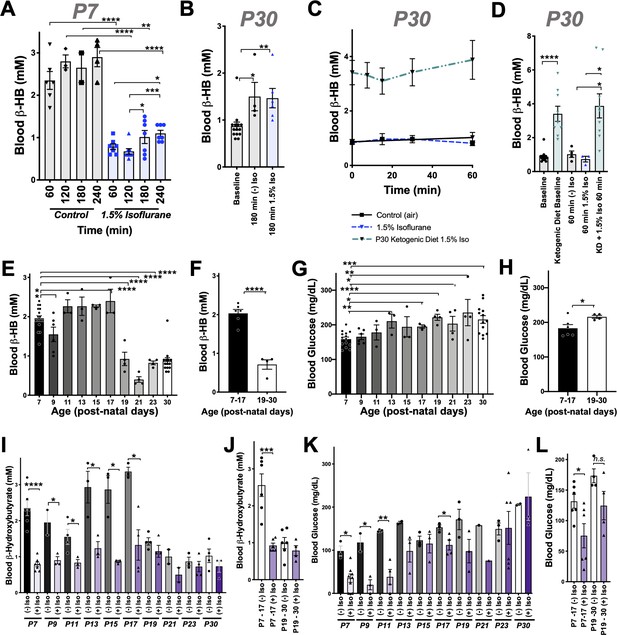
Anesthesia sensitivity of ketosis is unique to neonatal ketogenesis.
(A) Blood β-HB concentration in P7 mice exposed to 1.5% isoflurane anesthesia for 1–4 hr. One-way ANOVA *p<0.0001; *p<0.05, **p<0.005, ***p<0.005, ****p<0.0001 by pairwise t-test. (B) Blood β-HB concentration in P30 adolescent mice at baseline compared to 180 min of exposure to 1.5% isoflurane or control conditions. One-way ANOVA **p<0.005; *p<0.05, **p<0.005 by pairwise t-test. (C, D) Blood β-HB in P30 mice raised on a ketogenic diet and exposed to 1.5% isoflurane. (C) No significant differences observed at any exposure time compared to baseline within the same group. (D) Bar graphs with individual datapoints from (C), pairwise comparisons to control fed mice and control fed mice exposed to either 1.5% isoflurane or control conditions. One-way ANOVA ****p<0.0001. ****p<0.0001, *p<0.05 by pairwise t-test. Control data also appear in Figure 1E,F. (E) Baseline blood β-HB levels in mice as a function of age. One-way ANOVA ****p<0.0001. Comparisons to P7: *p<0.05, ****p<0.0001 by pairwise t-test. (F) Median blood β-HB values by age compared between post-natal periods P7–P17 and P17–P30 ages. ****p<0.0001 by pairwise t-test. (G) Baseline blood glucose levels in mice as a function of age. One-way ANOVA **p<0.005. Comparisons to P7: *p<0.05, **p<0.005, ***p<0.0005, ****p<0.0001 by pairwise t-test. (H) Median blood glucose values by age compared between post-natal periods P7–P17 and P17–30 ages. *p<0.05 by pairwise t-test. (I) Pairwise comparisons of blood β-HB in mice exposed to 1 hr of 1.5% isoflurane or control conditions at various post-natal ages. One-way ANOVA ****p<0.0001. Pairwise comparisons by treatment at each age: *p<0.05, ****p<0.0001 by pairwise t-test. Treatments non-significantly different where p-values not indicated. (J) Median β-HB values in each age and treatment group from (I) grouped by post-natal periods P7–P17 and P17–P30. One-way ANOVA ****p<0.00001, ***p<0.0005 by pairwise t-test. (K) Pairwise comparisons of blood glucose in mice exposed to 2 hr of 1.5% isoflurane or control conditions at various post-natal ages. One-way ANOVA ****p<0.0001. Pairwise comparisons by treatment at each age: *p<0.05, **p<0.005 by pairwise t-test. Treatments non-significantly different where p-values not indicated. (L) Median β-HB values in each age and treatment group in (K) grouped by post-natal periods P7–P17 and P17–P30. One-way ANOVA **p<0.005. Pairwise comparison **p<0.005 by pairwise t-test. (A–L) In all graphs, each datapoint represent values derived from an individual animal, with the exception of F, H, J, and L panels, where individual datapoints represent the mean values at different ages, as indicated. In the P30 datasets, multiple timepoints were collected per animal using the tail-prick method, whereas all neonate datapoints represent one animal with no repeat measurements (see Materials and methods).
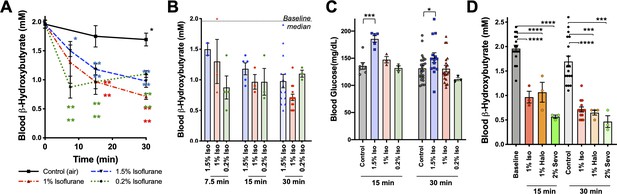
Metabolic effects of volatile anesthetics are uncoupled from sedation and properties common to multiple VA compounds.
(A) Blood β-HB levels in P7 mice exposed to varying levels of isoflurane as a function of time exposed. 1.5% and baseline as in Figure 1C shown for comparison. Pairwise comparisons versus baseline *p<0.05, ****p<0.0001 by pairwise t-test. p-values color coded to indicate exposure condition. (B) Blood β-HB levels in P7 mice exposed to varying concentrations of isoflurane for 7.5, 15, 30, or 60 min. Median values in baseline and 60 min control treated groups indicated by horizontal lines. (C) Blood glucose levels in P7 mice exposed to varying concentrations of isoflurane for 15 or 30 min. ***p<0.0005, *p<0.05 by pairwise t-test. (D) Blood β-HB levels in P7 mice exposed to sub-anesthetic concentrations of isoflurane, halothane, or sevoflurane for 15 or 30 min. ****p≤0.0001, ***p=0.0003 by pairwise t-test.
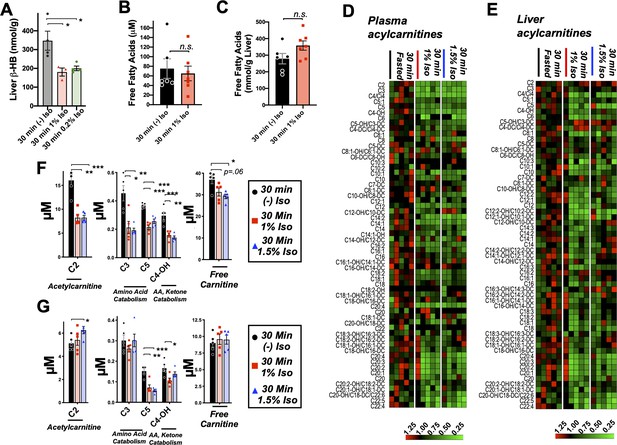
Brief exposure to isoflurane impairs fatty acid metabolism.
(A) β-HB concentration in whole liver of P7 mice exposed to 30 min fasting (n = 4), 1% isoflurane (n = 3), or 0.2% isoflurane (n = 4). ANOVA p<0.05, *p<0.05 by pairwise t-test. (B) Free fatty acid concentrations in blood of P7 neonatal mice exposed to 30 min of fasting (n = 6) or 1% isoflurane (n = 7). Not significantly different by pairwise t-test. (C) Free fatty acid concentrations in liver of P7 neonatal mice exposed to 30 min of fasting (n = 6) or 1% isoflurane (n = 7). Not significantly different by pairwise t-test. (D) Profiling of plasma acyl-carnitines in P7 neonatal mice exposed to 30 min of fasting, 1% isoflurane, or 1.5% isoflurane, n = 5 per treatment group (each column is one animal). Heat map rows (individual acyl-carnitine species) normalized to 30 min fasted control group median values, with relative levels indicated by color map, below. (E) Profiling of liver acyl-carnitines in P7 neonatal mice exposed to 30 min of fasting, 1% isoflurane, or 1.5% isoflurane, n = 5 per treatment group (each column is one animal). Heat map rows (individual acyl-carnitine species) normalized to 30 min fasted control group median values. Relative levels indicated by color map, below. (F) Plasma concentrations of major acyl-carnitine species C2, C3, C5, and C4-OH, from (D), and free carnitine. *p<0.05, **p<0.005, and ***p<0.0005 by pairwise t-test. Treatment group as indicated by color and datapoint shape indicated in legend. (G) Liver concentrations of major acyl-carnitine species C2, C3, C5, and C4-OH, from (E), and free carnitine. (A–G) *p<0.05, **p<0.005, and ***p<0.0005 by pairwise t-test. Treatment group as indicated by color and datapoint shape indicated in legend.
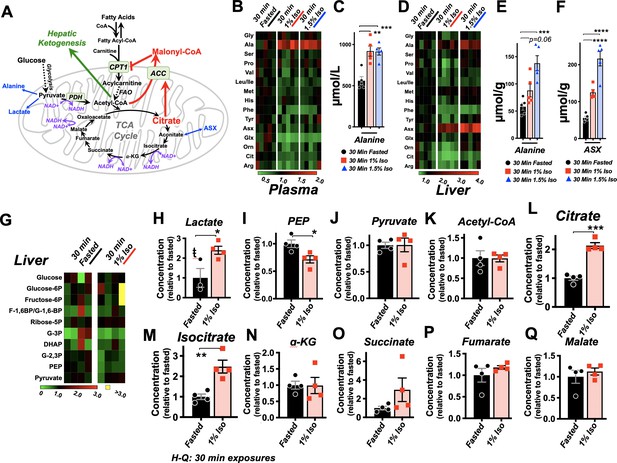
Isoflurane exposure leads to cataplerosis and citrate accumulation.
(A) Schematic of mitochondrial metabolism of glucose and fatty acids. The cataplerotic amino acids alanine and aspartate/asparagine (ASX) are generated when TCA cycle flux is impaired, and lactate is generated under conditions where glucose entry into the TCA cycle is disrupted (blue text). Citrate plays a key role in mediating feedback inhibition by activating acetyl-CoA carboxylase (ACC) to generate malonyl-CoA, which is an inhibitor of CPT1. CPT1 activity is necessary in order to enable entry of fatty acids into the mitochondria for fatty acid oxidation (FAO). Multiple steps of the TCA cycle consume NADH and are inhibited by NAD+ (purple). (B) Profiling of plasma amino acids in P7 neonatal mice exposed to 30 min of 1% isoflurane, 1.5% isoflurane, or control conditions. Columns represent individual animals, with each metabolite normalized to the 30 min fasted group. (C) Bar graphs of alanine data from (B). ANOVA ***p<0.0005. **p<0.005, ***p<0.0005 by pairwise t-test. (D) Profiling of liver amino acids in P7 neonatal mice exposed to 30 min of 1% isoflurane, 1.5% isoflurane, or control conditions. Columns within the heat map represent individual animals, with each metabolite normalized to the 30 min fasted group. (E) Bar graphs of alanine data from (D). ANOVA ***p-value<0.0005. ***p<0.0005 by pairwise t-test. (F) Bar graphs of aspartate/asparagine data from (D). ANOVA ****p-value<0.0001. ****p<0.0001 by pairwise t-test. (G) Profiling of glycolysis intermediates in P7 neonatal mice treated exposed to 30 min 1% isoflurane or control conditions. Columns within the heat map represent individual animals; each row (metabolite) is normalized to the 30 min fasted group. (H–Q) Lactate (H), PEP (I), pyruvate (J), and TCA cycle intermediates in liver of P7 neonatal mice treated exposed to 30 min 1% isoflurane or control conditions (K–Q). *p<0.05, **p<0.005, ***p<0.0005 by pairwise t-test. ŧ - one outlier in the control group (value 6.61) detected by Grubbs test and removed (a = 0.1) (see Figure 5—figure supplement 1).
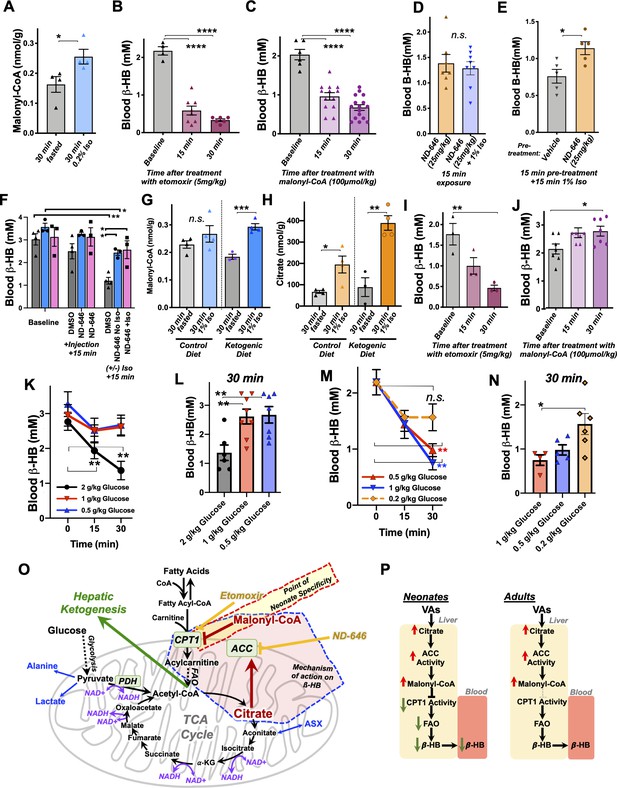
Mechanism of β-HB depletion in neonates.
(A) Hepatic malonyl-CoA concentrations in P7 neonates exposed to 30 min of 0.2% isoflurane or control conditions. *p<0.05 by pairwise t-test. (B) Blood β-HB in P7 neonatal mice treated with 5 mg/kg etomoxir by IP injection. ****One-way ANOVA p<0.0001, ****p<0.0001 by pairwise t-test. (C) Blood β-HB in P7 neonatal mice treated with 100 μmol/kg malonyl-CoA by IP injection. ****One-way ANOVA p<0.0001, ****p<0.0001 by pairwise t-test. (D) Blood β-HB in P7 neonatal mice treated with 20 mg/kg ND-646 followed by 15 min exposure to 1% isoflurane or control conditions. n.s. – not significant. (E) Blood β-HB in P7 neonatal mice treated with ND-646 or vehicle solution 15 min prior to a 15 min exposure to 1% isoflurane. *p<0.05 by pairwise t-test. (F) Blood β-HB in P16 neonatal mice at baseline, at 15 min following treatment with 10% DMSO in PBS or 2.5 mg/kg ND-646 in 10% DMSO/PBS, and then after a subsequent 15 min exposure to control conditions or 1% isoflurane. Blood β-HB was reduced by injection with ND-646 over this time period, but ND-646 significantly attenuated the impact of isoflurane. *p<0.05 by pairwise t-test. (G) Hepatic malonyl-CoA in P30 animals raised on control or ketogenic diet and exposed to 30 min of 1% isoflurane or control conditions. ***p<0.0005 by pairwise t-test. (H) Hepatic citrate in P30 animals raised on control or ketogenic diet and exposed to 30 min of 1% isoflurane or control conditions. *p<0.05, **p<0.005 by pairwise t-test. (I) Blood β-HB in P30 mice raised on a ketogenic diet treated with 5 mg/kg etomoxir by IP injection. *One-way ANOVA p<0.05; **p<0.01 by pairwise t-test. (J) Blood β-HB in P30 mice raised on a ketogenic diet treated with 100 μmol/kg malonyl-CoA by IP injection. *One-way ANOVA p<0.05; *p<0.05 by pairwise t-test. (K) Blood β-HB in P30 mice raised on a ketogenic diet and treated with 2, 1, or 0.5 g/kg glucose by IP injection. **p<0.05 by pairwise t-test, comparison to baseline (t = 0). (L) Bar graph of 30 min data in (J) to show individual datapoints. O*one-way ANOVA p-value<0.005; **p<0.005 by pairwise t-test. (M) Blood β-HB in P7 mice treated with 1, 0.5, or 0.2 g/kg glucose by IP injection. **p<0.05 by pairwise t-test, comparison to baseline (t = 0). (N) Bar graph of 30 min data in (L) to show individual datapoints. *One-way ANOVA p-value<0.05; *p<0.05 by pairwise t-test. (O) Schematic of the metabolic processes underlying the effects of VAs with targets of relevant pharmacologic agents indicated. (P) Model of VA action on circulating β-HB in neonates and the differential effects in P30 mice.
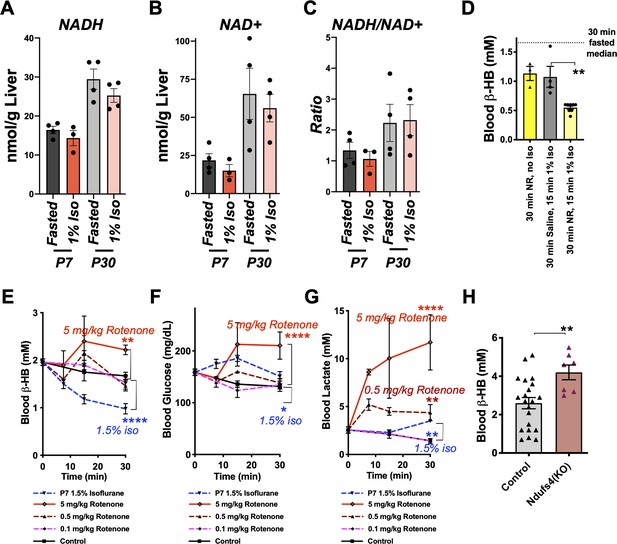
ETC CI and the metabolic response to VAs.
(A) Whole-liver NADH concentrations in P7 and P30 mice exposed to 30 min of 1% isoflurane or control conditions. Isoflurane exposure did not significantly alter NADH at either age. (B) Whole-liver NAD+ concentrations in P7 and P30 mice exposed to 30 min of 1% isoflurane or control conditions. Isoflurane exposure did not significantly alter NAD+ at either age. (C) Whole-liver NADH/NAD+ ratio (from data in A and B) in P7 and P30 mice exposed to 30 min of 1% isoflurane or control conditions. Isoflurane exposure did not significantly alter the NADH/NAD+ ratio at either age. (D) Blood β-HB concentration in mice treated with 500 mg/kg nicotinamide riboside (NR) or saline and collected immediately or exposed to 15 min of 1% isoflurane. 30 min fasted median (see Figure 1) shown for reference. NR treatment led to an exacerbation of β-HB loss in the isoflurane-exposed mice. Data represent animals from two litters split among treatments. **p<0.01 by pairwise t-test. (E) Blood glucose in P7 mice treated with 5, 0.5, or 0.1 mg/kg rotenone. Control treated and 1.5% isoflurane-exposed data from Figure 1 shown for reference. **p<0.005, ****p<0.0001 by pairwise t-test vs control treated. Only 30 min timepoint comparison shown. (F) Blood β-HB from P7 mice treated with 5, 0.5, or 0.1 mg/kg rotenone. Control treated and 1.5% isoflurane-exposed data from Figure 1 shown for reference. *p<0.05, ****p<0.0001 by pairwise t-test vs control treated. Only 30 min timepoint comparison shown. (G) Blood lactate from P7 mice treated with 5, 0.5, or 0.1 mg/kg rotenone. Control treated and isoflurane-exposed data from Figure 1—figure supplement 1 shown for reference. **p<0.005, ****p<0.0001 by pairwise t-test vs control treated. Only 30 min timepoint comparison shown. (H) Blood β-HB from ad-libitum fed P17 neonatal Ndufs4 (KO) and control (heterozygous or wild-type) mice. **p<0.005 by pairwise t-test. (A–H) n ≥ 3 animals for every timepoint, biological replicates indicated in bar graphs.
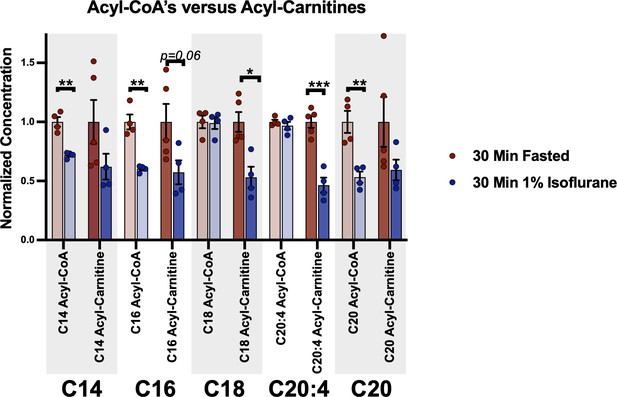
Acyl-CoA versus acyl-carnitine changes.
Quantification of select long-chain fatty acid acyl-CoA’s and acyl-carnitine levels. While exposure to isoflurane results in a broad depletion of all liver acyl-carnitines (Figure 3), without impacting overall levels of free fatty acids in liver, the precise impact of anesthesia on individual fatty acids appears somewhat heterogeneous. C18 and C20:4 show depletion of acyl-carnitines without changes to acyl-CoA levels, while both acyl-carnitine and acyl-CoA levels are reduced for C14, C16, and C20. **p<0.005, *p<0.05 by pairwise t-test.
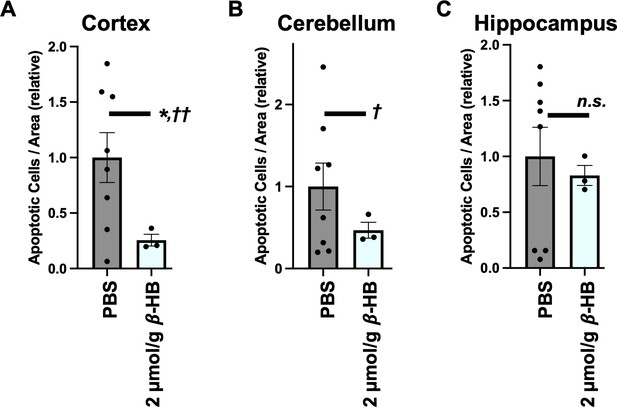
Impact of exogenous beta-hydroxybutyrate on anesthesia-induced neurotoxicity.
In a pilot cohort, we treated animals with saline (PBS) or 2 µmol/g β-HB by IP injection then immediately exposed to 1.5% isoflurane for 4 hr and allowed to recover for 2 hr, as we have previously done (Johnson et al., 2019a). β-HB administration prior anesthesia led to a significant reduction in cleaved caspase-3-positive nuclei in the cortex (A) and a trend toward reduced cleaved caspase-3-positive cells in the cerebellum (B), but no significant differences detected in the hippocampus (C). *p<0.05 by pairwise two-tailed unequal variances t-test, ††p<0.005 by pairwise one-tailed unequal variances t-test, †p=0.06 by pairwise one-tailed unequal variances t-test. n.s. – not significant. n = 8 PBS treated, 3 β-HB treated. Samples were blinded; the presence of apoptotic cells was determined by counting the number of cleaved caspase-3-positive nuclei.
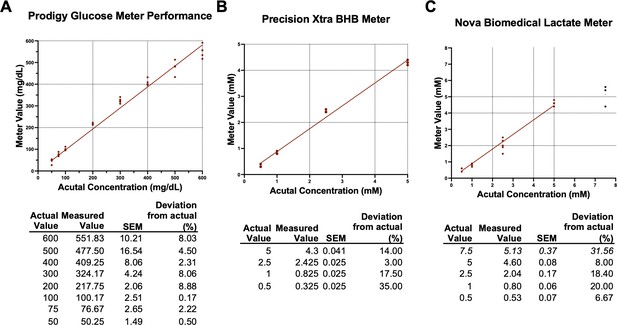
Point-of-care meter performance.
Assessment of performance of point-of-care meters: (A) Prodigy Autocode glucometer, (B) Precision Xtra glucose and BHB meter (used for BHB only), and (C) Nova Biomedical lactate meter. All measures were performed using the relevant molecule dissolved into phosphate buffered saline at pH 7.4 and kept warm at 37° C for sampling to match physiologic conditions. Each concentration was read 3–5 times, all datapoints shown on graphs. Red lines are best fit linear regression curves, included to show linear range of measurements.
Additional files
-
Source data 1
Source data for all figures.
- https://cdn.elifesciences.org/articles/65400/elife-65400-data1-v2.xlsx
-
Transparent reporting form
- https://cdn.elifesciences.org/articles/65400/elife-65400-transrepform-v2.pdf