Unveiling the sensory and interneuronal pathways of the neuroendocrine connectome in Drosophila
Figures
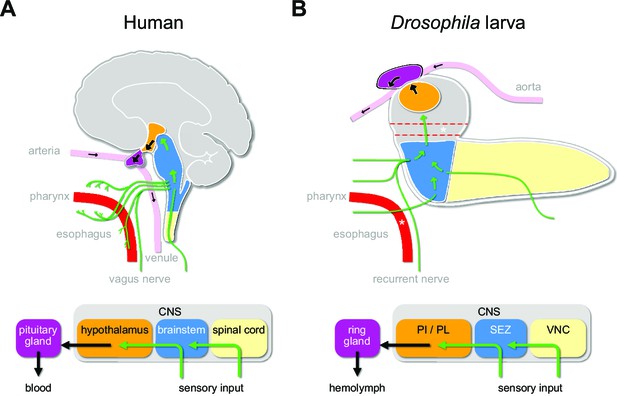
Sensory to endocrine pathways.
(A) Schematic showing information flow from sensory input (green) to the endocrine system in the human brain compared to the Drosophila larval brain. (B) Asterisks denote the foramen (dotted red tube), where the esophagus (solid red tube) would pass through the CNS. Green arrows denote flow of sensory information; black arrows denote release of hormones into the circulatory system. CNS: central nervous system; PI: pars intercerebralis; PL: pars lateralis; SEZ: subesophageal zone; VNC: ventral nerve cord.
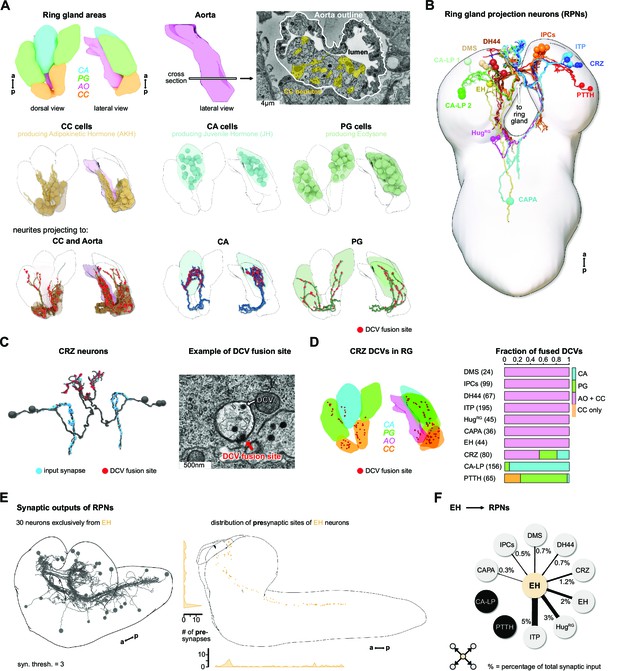
Reconstruction of the Drosophila larval ring gland and RPNs.
(A) Upper panel: 3D reconstructed RG areas in dorsal and lateral view (CC = orange, PG = green, CA = blue, AO = pink). Cross section of the AO: colored areas represent single neurites of different CC cells. Middle panel: dorsal and lateral view of the RG showing the different cells in the distinct RG areas (CC, CA, and PG). Lower panel: neurites innervating the RG areas were separated based on innervation of the CC and aorta, only CA or only PG. Fused DCVs are marked as red dots. (B) Schematic of all 56 neurons innervating the RG named by the main neuropeptide produced. Total number of neurons per RPN cluster: DMS = 4, IPCs = 14, DH44 = 6, CRZ = 6, ITP = 8, CA-LP = 6, PTTH = 4, HugRG = 4, CAPA = 2, EH = 2. For clarity, only one side is shown for each neuronal cluster. (C) Left: reconstructed CRZ. Fused DCVs were marked as non-polar output synapses at distal neurites in RG tissues (red dots). Blue dots represent chemical synaptic input sites. Right: example picture of a DCV fusion site in the EM volume (DCV has to be fused to the membrane). (D) Left: magnification of the reconstructed RG with spatial distribution of CRZ DCV fusion sites (red dots).Right: quantification of all DCV fusion sites found in the RG areas for each RPN group. Numbers in brackets are total numbers of marked DCVs. The X-axis represents a fraction of fused DCVs. (E) Left: synaptic outputs of all RPNs (threshold = 3 synapses) constitute in total 30 neurons, which are exclusively downstream of EH RPNs. Right: spatial distribution of presynaptic sites of EH. EH neurons are the only RPNs having presynaptic sites located along abdominal, thoracic segments, and SEZ and protocerebrum. (F) EH neurons synaptically target other RPNs. Percentage represents the fraction of input of distinct RPNs from EH neurons, for example, ITP neurons receive 5% of its inputs from EH. a: anterior; AO: aorta; CA: corpus allatum; CA-LP: corpus allatum innervating neurosecretory neurons of the lateral protocerebrum; CAPA: capability neurons; CC: corpora cardiaca; CRZ: corazonin neurons; DCVs: dense core vesicles; DH44: diuretic hormone 44 neurons; DMS: Drosophila myosuppressin neurons; EH: eclosion hormone neurons; HugRG: Hugin neurons innervating ring gland; IPCs: insulin-producing cells; ITP: ion transport peptide neurons; p: posterior; PG: prothoracic gland; PTTH: prothoracicotropic hormone neurons; RG: ring gland; RPN: ring gland projection neurons; SEZ: subesophageal zone; ssTEM: serial section transmission electron microscope.
-
Figure 2—source data 1
Comprehensive overview of all Drosophila RPN clusters.
- https://cdn.elifesciences.org/articles/65745/elife-65745-fig2-data1-v1.docx
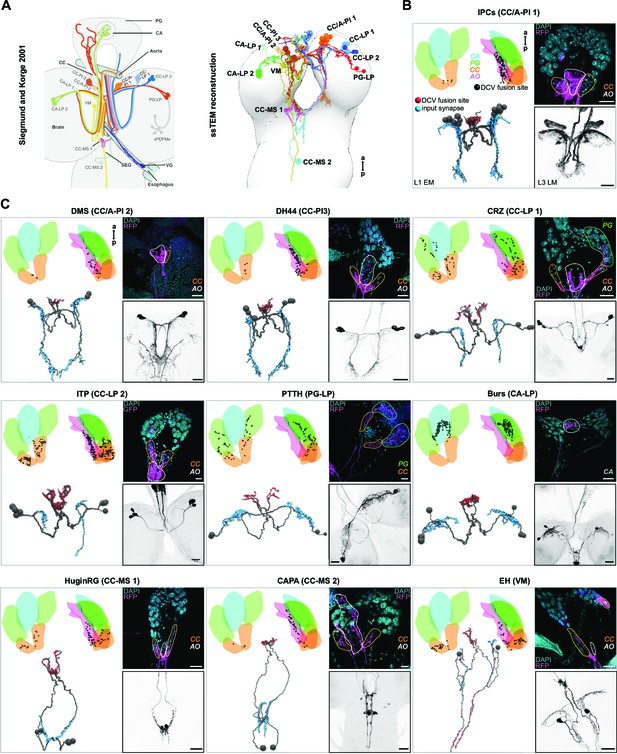
Neurons projecting to the ring gland in Drosophila larvae.
(A) All RPNs could be re-identified by comparing the neurons innervating the ring gland previously published by Siegmund and Korge, 2001 to all RPNs found in the ssTEM volume (right panel). (B, C) DCV fusion sites were analyzed (black dots in dorsal and lateral view of the ring gland) in the ssTEM dataset (upper left part of each panel). Lower left part of each panel: blue dots represent chemical postsynapses in the brain and red dots represent DCV fusion sites in the RG. Note that presynapses for EH neurons are also found in the CNS (red dots). RPNs identified in the ssTEM volume were compared to respective expression patterns of Gal4/LexA lines in fluorescence stainings (RFP/GFP, right side of each panel). Upper right of each panel displays Gal4/LexA-driven expression patterns of RFP or GFP in the ring gland co-stained with DAPI to visualize RG areas. CA-LP neurons were visualized using a Bursicon-Gal4 line. Expression in the CA has not been reported yet for Bursicon. Note that ssTEM volume was generated from a first instar larva (L1 EM), whereas fluorescence staining/light microscopy was carried out in third instar larvae (L3 LM). For all genotypes, see Material and methods section. All scale bars represent 20 µm, except scale bar in CAPA analysis (10 µm). a: anterior; AO: aorta; Burs: bursicon neurons; CA: corpus allatum; CA-LP: corpus allatum innervating neurosecretory neurons of the lateral protocerebrum; CAPA: capability neurons; CC: corpora cardiaca; CC‐LP: corpora cardiaca innervating neurosecretory neurons of the lateral protocerebrum; CC‐MS: corpora cardiaca innervating neurosecretory neurons of the medial subesophageal ganglion; CC‐PI: corpora cardiaca innervating neurosecretory neurons of the pars intercerebralis; CC/A‐PI: corpora cardiaca and aorta innervating neurosecretory neurons of the pars intercerebralis; CRZ: corazonin neurons; DAPI: 4′,6-diamidino-2-phenylindole; DCVs: dense core vesicles; DH44: diuretic hormone 44 neurons; DMS: Drosophila myosuppressin neurons; EH: eclosion hormone neurons; GFP: green fluorescent protein; HuginRG: hugin neurons innervating ring gland; IPCs: insulin-producing cells; ITP: ion transport peptide neurons; p: posterior; PG: prothoracic gland; PG‐LP: prothoracic gland innervating neurosecretory neurons of the lateral protocerebrum; PTTH: prothoracicotropic hormone neurons; RFP: red fluorescent protein; RG: ring gland; RPNs: ring gland projection neurons; SEG: subesophageal ganglion; sPDFMe: small pigment dispersing factor neurons in the medulla; ssTEM: serial section transmission electron microscope; VG: ventricular ganglion; VM: ventromedial cells.
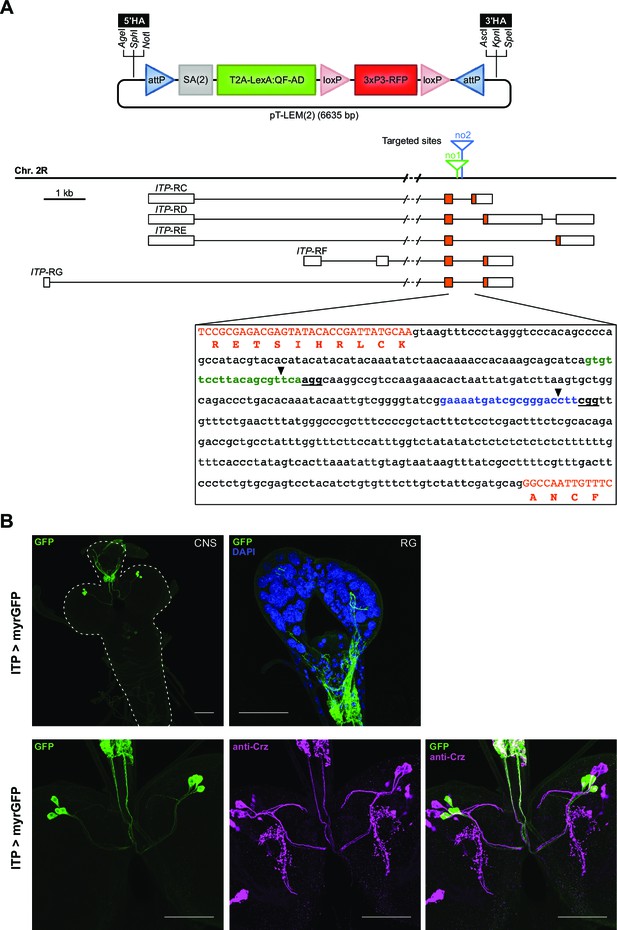
CRISPR/Cas-dependent integration of T-LEM cassettes into ITP intron.
(A) Schematic of the T2A-LexA expression module (T-LEM) vector pT-LEM(2), which contains RFP under the control of 3xP3. Multiple cloning sites flanking T-LEM permit insertion of appropriate homology arms to target ITP intron. SA(2) in pT-LEM(2) allows integration of T-LEM as a new exon into phase two introns. Genomic locus on second chromosome with all five predicted splice variant transcripts of ITP gene depicting two intronic sites (no1, no2) targeted for T-LEM insertion. Boxes represent exons; orange areas show protein-coding regions. Sequence of ITP intron displaying protospacers no1 (green) and no2 (blue). PAMs are bold and underlined. Coding exons are capitalized and orange. One-letter code amino acid sequence is shown in orange and positioned under the first nucleotide of the encoding triplet. Black arrowheads mark target sites for T-LEM insertion. (B) Fluorescence staining of ITP-T2A-LexA line driving LexAop-myrGFP. Upper panel: expression in whole CNS and RG. Lower panel: colocalization analysis with CRZ reveals no overlap of both RPN groups. For all genotypes, see Material and methods. All scale bars represent 20 µm. 3'HA: upstream homology arm; 3xP3: synthetic eye promoter; 5'HA: downstream homology arm; AD: activating domain; attP: attachment site on the phage part; bp: base pairs; Chr. 2 R: right arm of second chromosome; CNS: central nervous system; Crz: corazonin; DAPI: 4′,6-diamidino-2-phenylindole; GFP: green fluorescent protein; ITP: ion transport peptide; ITP-RC/-RD/-RE/-RF/-RG: ITP transcript C/D/E/F/G; kb: kilo base pairs; loxP: locus of crossover in phage P1 sequence; myrGFP: myristoylated green fluorescent protein; PAM: protospacer adjacent motif; RFP: red fluorescent protein; RG: ring gland; RPNs: ring gland projection neurons; SA(2): splice acceptor sequence and intron phase two linker; T2A: 2A self-cleaving peptide from Thosea asigna virus.
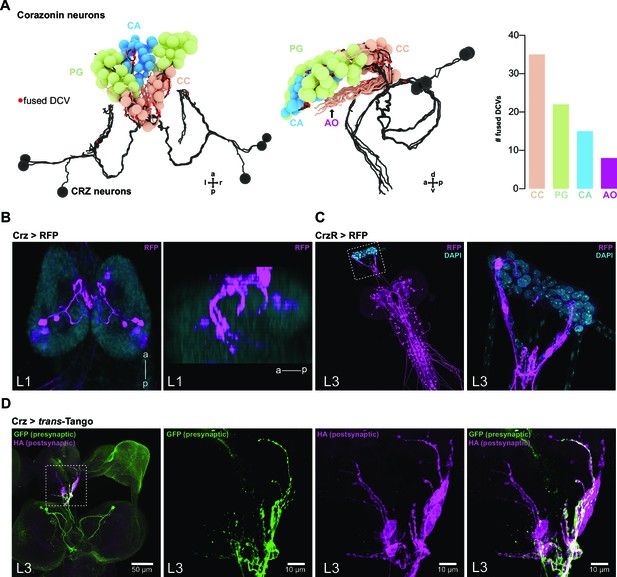
Fused DCV sites as proxy for real release sites in the ring gland: example CRZ.
(A) Reconstructed CRZ in dorsal and lateral view with projections to RG cells (red dots are DCV fusion sites). Right bar plot shows quantification of the number of fused DCVs in CC, PG, CA, and AO. Most output release sites were found in the CC. (B) Analysis of the Crz-Gal4 line in L1 CNS shows only activity in CRZ projecting to RG. View angles are the same as in (A). (C) Analysis of CrzR in L3 CNS (left) and in RG (right) shows CrzR activity in CC cells. (D) Staining of post-'peptidergic' cells of CRZ using the trans-Tango system (note that no output synapses for CRZ were identified in ssTEM volume; whether synapses are present in L3 stage is not known). Only CC cells were marked as being postsynaptic to CRZ. For all genotypes, see Materials and methods section. a: anterior; AO: aorta; CA: corpus allatum; CC: corpora cardiaca; CRZ: corazonin neurons; Crz: corazonin (gene/peptide related); CrzR: corazonin receptor; d: dorsal; DAPI: 4′,6-diamidino-2-phenylindole; DCV: dense core vesicle; GFP: green fluorescent protein; HA: hemagglutinin tag; l: left; L1: first instar larval stage; L3: third instar larval stage; p: posterior; PG: prothoracic gland; r: right; RFP: red fluorescent protein; RG: ring gland; v: ventral.
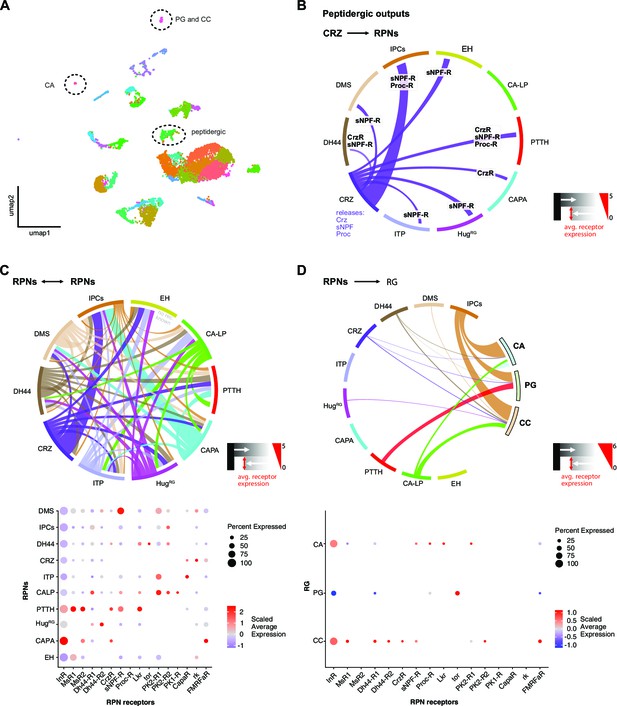
Analysis of larval brain transcriptome data.
(A) Cluster plot (umap) of all cell clusters derived from the larval brain transcriptome (Brunet Avalos et al., 2019). Highlighted are clusters containing peptidergic and RG-specific cells (CC, CA, and PG). (B) Graphical representation of peptide receptor interactions of CRZ other RPNs. Since CRZ are known to release additionally sNPF and proctolin, they potentially interact with RPNs having the respective receptors for these peptides. (C) Peptide receptor connectivity of all RPNs using the known expressed peptide and respective receptors. This illustrates dense lateral connections of RPNs. Average receptor expression is visualized by thickness of connections (0–5). Dot plot showing the scaled average peptide receptor expression (color code) and certain percentage of the RPN cell clusters (size of dots). (D) Peptide receptor connectivity of the RPNs to the RG areas (CC, PG, and CA). InR expression is strongest in all three tissues. Dot plot showing the scaled average peptide receptor expression (color code) and certain percentage of the distinct RG areas. Analysis was performed using a modified version of the Seurat workflow published by Satija lab (Stuart et al., 2019). For details on separation parameters of RPNs, please see Materials and methods section. avg.: average; CA: corpus allatum; CA-LP: corpus allatum innervating neurosecretory neurons of the lateral protocerebrum; CAPA: capability neurons; CapaR: capability receptor; CC: corpora cardiaca; CRZ: corazonin neurons; Crz: corazonin (gene/peptide related); CrzR: corazonin receptor; DH44: diuretic hormone 44 neurons; Dh44-R1/-R2: diuretic hormone 44 receptor 1/2; DMS: Drosophila myosuppressin neurons; EH: eclosion hormone neurons; FMRFaR: FMRFamide receptor; HugRG: hugin neurons innervating ring gland; InR: insulin-like receptor; IPCs: insulin-producing cells; ITP: ion transport peptide neurons; Lkr: leucokinin receptor; MsR1/MsR2: myosuppressin receptor 1/2; PG: prothoracic gland; PK1-R: pyrokinin one receptor; PK2-R1/-R2: pyrokinin 2 receptor 1/2; Proc: proctolin; Proc-R: proctolin receptor; PTTH: prothoracicotropic hormone neurons; RG: ring gland; rk: rickets; RPNs: ring gland projection neurons; sNPF: short neuropeptide F precursor; sNPF-R: short neuropeptide F receptor; tor: torso.
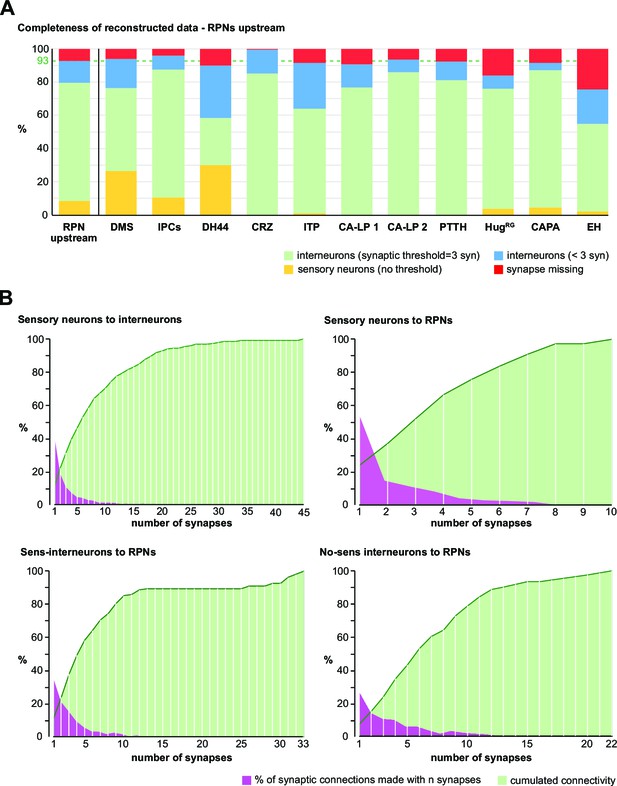
Thresholds for reconstruction and analysis of the RPN connectome.
(A) Sensory neurons with every presynaptic site have been completely reconstructed by Miroschnikow et al., 2018. RPNs have been reconstructed completely with every postsynaptic site. Interneurons located upstream of RPNs were completely reconstructed using a synaptic threshold of 3 (to RPNs). This made it possible to reach in total 93% of all incoming synaptic connections to the RPNs. Yellow = synapses from sensory neurons; green = annotated synapses from interneurons; blue = synapse bycatch from interneurons with 1–2 synapses to respective RPN cluster (these interneurons, however, have minimum three synapses to other RPN clusters); red = synapses from unidentified upstream partners. Thus even more synapses were reconstructed in total than interneurons having three or more synapses to RPNs. (B) Upper left: shown is the percentage of synaptic connections of sensory neurons to interneurons made with a certain number of synapses. Sensory neurons make most of their connections (nearly 40% of all connections) with one synapse to an interneuron, reaching about 20% of their connectivity by 1- and 2-synapse connections. Upper right: over 50% of connections from sensory neurons to RPNs are made by 1-synapse connections. Nearly 40% of connectivity from sensory neurons to RPNs are made by 1- and 2-synapse connections. Lower left: sensory-receiving interneurons made more than 30% of their synaptic connections with 1-synapse and reached 20% of connectivity to RPNs with only 1- and 2-synapse connections. Lower right: non-sensory-receiving interneurons made less than 30% of their synaptic connections with 1-synapse and about 15% of their total connectivity to RPNs with 1- or 2-synapses. In general, sensory neurons made more ‘weak’ (one or two synapses) connections than interneurons to the RPNs. Thus, using a reconstruction threshold for interneurons of three synapses per connection to a RPN and using the minimal possible threshold of 1 for the sensory system connected to RPNs (having been reconstructed by Miroschnikow et al., 2018), it was possible to fully describe the RPN connectome with a completeness of 93%. For abbreviations, see Figure 2—figure supplement 4. In subsequent analysis, synaptic thresholds were chosen based on the scientific message we want to convey in the clearest possible manner and may differ depending on the type of graphs, schemes, or tables used. These can be presented in terms of absolute number of synapses or relative to a total number (e.g., percentage of total incoming synapses for a given neuron). One cannot say a priori which is more useful for a given figure as the number of synapses and synaptic partners can vary widely depending on the neuron.
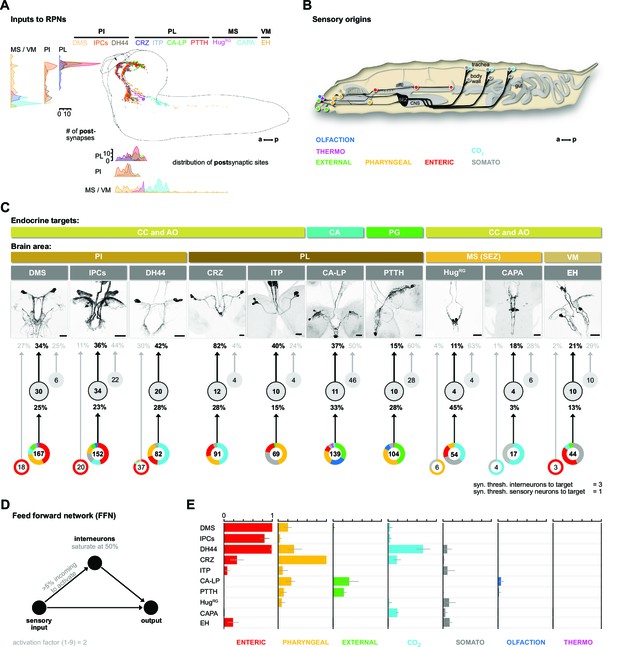
Inputs of RPNs and sensory origins.
(A) Spatial distribution of postsynaptic sites of all RPNs (color coded). RPN postsynaptic sites are located along upper SEZ and in the protocerebrum in a sprinkler-like fashion. (B) Schematic side view of a Drosophila larva. Colored dots represent the location of sensory organs, based on their sensory origin. (C) Synaptic connections to RPNs (grouped) from top to bottom: RPNs are grouped by their endocrine targets or their location of somata within the CNS (brain area, colored bars). RPNs (displayed by expression pattern of the respective Gal4 or LexA lines) receive synaptic inputs (fraction of total synaptic inputs as percentage) from distinct sets of interneurons (numbers in circles represent the number of interneurons connected to RPNs), which in turn receive information from sensory neurons (fraction of total synaptic input as percentage). Colored pie charts represent the sensory profile through which interneurons (grouped) of each RPN group integrate sensory inputs (numbers in white circles). Colors of pie charts correspond to the respective sensory origins shown in (B). Note that the monosynaptic sensory neurons are also involved in polysynaptic pathways to the RPNs. (D) Scheme of the FFN. Sensory neurons are activated with an activation factor of 2 in the FFN. When more than 5% of presynaptic neurons are active, interneurons become activated up to an activity of 50%. (E) Summary of sensory-driven modulation of RPN output groups by FFN. The X-axis for each panel shows the mean activity of RPNs listed on the Y-axis. Colors represent the different sensory origins used to activate the network through 1- and 2-hop synaptic connections. a: anterior; AO: aorta; CA: corpus allatum; CA-LP: corpus allatum innervating neurosecretory neurons of the lateral protocerebrum; CAPA: capability neurons; CC: corpora cardiaca; CNS: central nervous system; CO2: carbon dioxide; CRZ: corazonin neurons; DCVs: dense core vesicles; DH44: diuretic hormone 44 neurons; DMS: Drosophila myosuppressin neurons; EH: eclosion hormone neurons; FFN: feed forward network; HugRG: hugin neurons innervating ring gland; IPCs: insulin-producing cells; ITP: ion transport peptide neurons; MS (SEZ): medial subesophageal ganglion; p: posterior; PG: prothoracic gland; PI: pars intercerebralis; PL: pars lateralis; PTTH: prothoracicotropic hormone neurons; RPNs: ring gland projection neurons; syn. thresh.: synaptic threshold; VM: ventromedial cells.
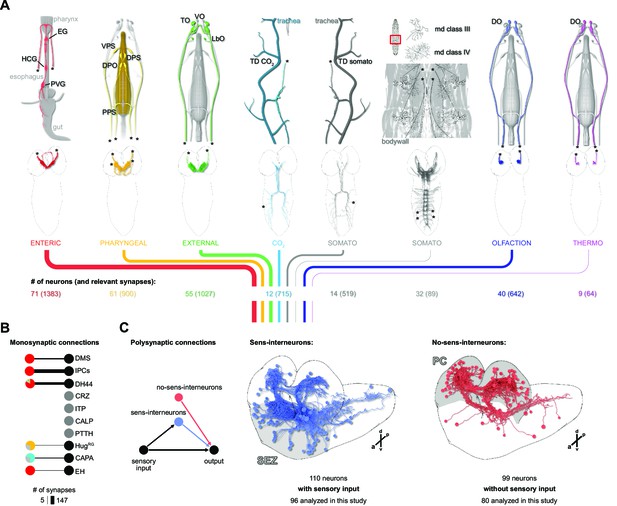
Connectivity of the distinct sensory origins to RPN upstream neurons.
(A) Top: schematic drawings of the dorsal view of individual sensory origins and their projections into the CNS. Strength of synaptic connections from different sensory origins is displayed as total synapse number from a given sensory origin by color-coded lines (thickness represents number of synapses). Main connected sensory origins (to RPN upstream neurons) derive from enteric, pharyngeal, external, TD (CO2), TD somato, and olfaction (B) All monosynaptic connections to RPNs. Note that neurons of the pars lateralis (CRZ, ITP, CA-LP, and PTTH) have no monosynaptic connections to the sensory system. (C) Presynaptic neurons of all RPNs (threshold = 3 synapses) were divided based on connectivity to sensory neurons (minimum two synapses, blue) or having no or minor connectivity to sensory neurons (<2 synapses) (red). Interneurons having three synapses to RPNs and their hemilateral partners connected to each RPN with at least one synapse were analyzed. If a hemilateral partner of an interneuron had no synaptic connection to a given RPN group, the interneuron was excluded from analysis. a: anterior; CALP: corpus allatum innervating neurosecretory neurons of the lateral protocerebrum; CAPA: capability neurons; CO2: carbon dioxide; CRZ: corazonin neurons; d: dorsal; DH44: diuretic hormone 44 neurons; DMS: Drosophila myosuppressin neurons; DO: dorsal organ; DPO: dorsal pharyngeal organ; DPS: dorsal pharyngeal sense organ; EG: esophageal ganglion; EH: eclosion hormone neurons; HCG: hypocerebral ganglion; HugRG: hugin neurons innervating ring gland; IPCs: insulin-producing cells; ITP: ion transport peptide neurons; LbO: labial organ; p: posterior; md: multidendritic neurons; PC: protocerebrum; PPS: posterior pharyngeal sense organ; PTTH: prothoracicotropic hormone neurons; PVG: proventricular ganglion; RPNs: ring gland projection neurons; SEZ: subesophageal zone; TD: tracheal dendritic neurons; TO: terminal organ; v: ventral; VO: ventral organ; VPS: ventral pharyngeal sense organ.
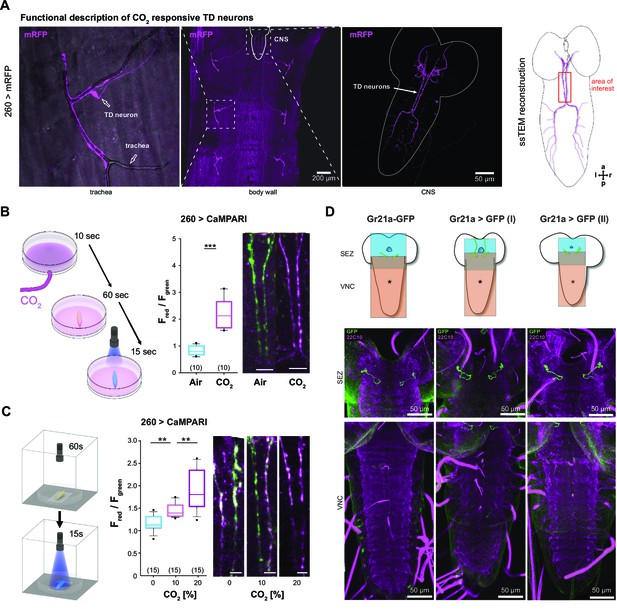
A subset of TD sensory neurons responds to CO2.
(A) TDs visualized in 260-Gal4 line crossed with UAS-mRFP. TD wrap around peripheral trachea and project to the VNC and SEZ (Qian et al., 2018). Right: dorsal view of TD projecting to the SEZ in the sTEM reconstruction. Area of interest was used for CaMPARI experiments. (B) Schematic of experimental setup. CO2 was blown for 10 s into Petri dish, larva was placed in Petri dish for 1 min, and subsequently illuminated with 405 nm light for 15 s. Red to green fluorescence ratios shown for TD dendrites projecting to the SEZ in 260-Gal4 line crossed with UAS-CaMPARI-1 line. Strong photoconversion was observed in larvae within CO2-enriched Petri dishes. Scale bars represent 20 µm. (C) Schematic setup for dose-dependent CO2 stimulation. Similar to (B), but CO2 concentration was 0, 10, or 20% CO2. Activation of TDs could be observed in a dose-dependent manner with rising concentrations of CO2. Scale bars represent 10 µm. (D) The known CO2 receptor Gr21a is not expressed in the TDs. Three different Gr21a promoter lines all drive target gene expression in the SEZ, but not in the TDs of VNC as seen in (A). Note that Gal4 driver line in Gr21a>GFP (I) (middle panel) was Bloomington line #23,890 and in Gr21a>GFP (II) (right panel) Bloomington line #24,147. For all genotypes, see Materials and methods section. a: anterior; CaMPARI: calcium-modulated photoactivatable ratiometric integrator; CNS: central nervous system; CO2: carbon dioxide; d: dorsal; GFP: green fluorescent protein; Gr21a: gustatory receptor 21a; l: left; mRFP: monomeric red fluorescent protein; p: posterior; r: right; SEZ: subesophageal zone; ssTEM: serial section transmission electron microscope; TD: tracheal dendritic neurons; VNC; ventral nerve cord.
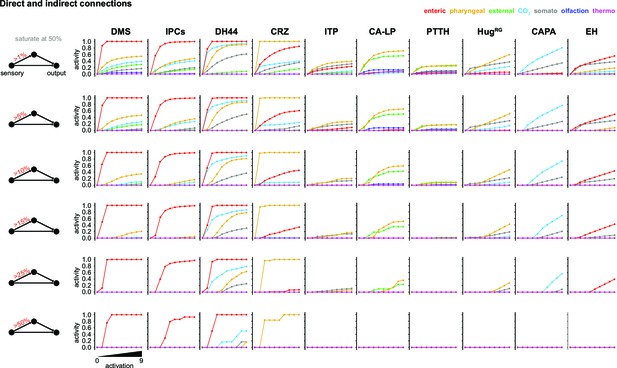
Parameter changes in the FFN.
Activity modulation of RPNs using direct and indirect connectivity to the sensory system (monosynaptic = direct, 2-hop polysynaptic = indirect). Only those interneurons were used that have at least three synapses to each of the RPNs. FFN: feed forward network; for other abbreviations, see Figure 3—figure supplement 1.
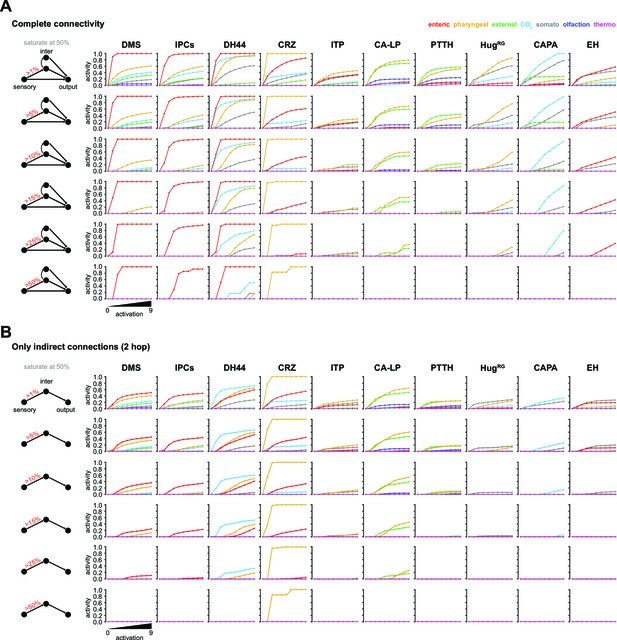
Parameter changes in the FFN.
(A) Activity modulation of RPNs using the complete connectivity to the sensory system monosynaptic = direct, 2-hop polysynaptic = indirect and 3-hop polysynaptic = indirect. Only those interneurons were used that have at least three synapses to each of the RPNs. (B) Activity modulation of RPNs using only 2-hop indirect connections. FFN: feed forward network; for other abbreviations, see Figure 3—figure supplement 1.
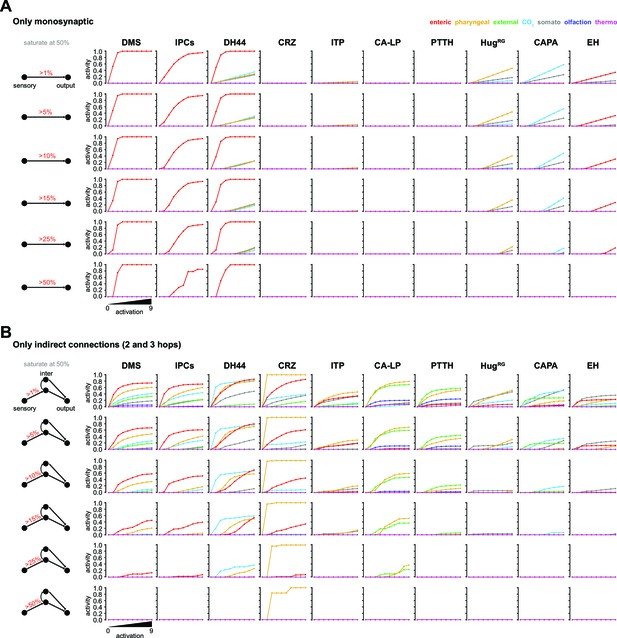
Parameter changes in the FFN.
(A) Activity modulation of RPN using only monosynaptic connections to the sensory origins. (B) Activity modulation of RPNs using 2- and 3-hop polysynaptic connections. FFN: feed forward network; for other abbreviations, see Figure 3—figure supplement 1.
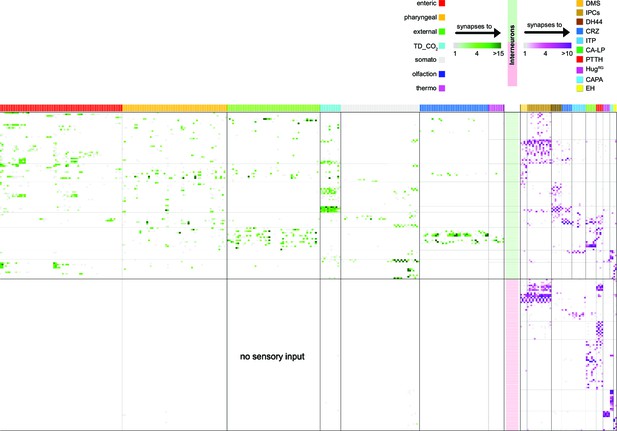
Adjacency matrix of all neurons used in this study.
Adjacency matrix showing sensory to interneuron (no synaptic threshold) and interneuron (selected with a synaptic threshold of at least three synapses to any of the RPNs) to RPNs. All synapses between shown neuron types were included. Top: color-coded sensory origins synapse onto upstream neurons (interneurons) of the RPNs (green heatmap). Upstream neurons synapse onto RPNs (magenta heatmap), clustered by peptide identity. Matrix: sensory origins are displayed by single cells color coded as above scheme. Interneurons were divided into those receiving and not receiving synaptic input from sensory neurons. Matrix was formatted using the connectivity of interneurons to specific peptidergic RPN clusters. For abbreviations, see Figure 3—figure supplement 1.
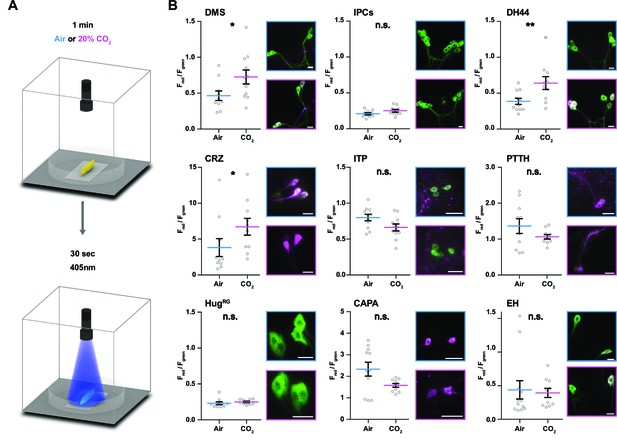
Impact of CO2 stimulation on RPNs.
(A) Setup for CO2 stimulation of intact larvae. After 1 min of CO2 exposure (0 or 20%), light of 405 nm wavelength was activated for 30 s. (B) Different peptide Gal4-lines driving expression of CaMPARI-2 in RPN clusters. Note that certain peptidergic clusters show baseline activity (CRZ, PTTH, CAPA) and therefore different scaling for the Y-axis was used, which represents the red to green fluorescence ratio. Significant activity changes could be observed for DMS, DH44, and CRZ upon CO2 stimulation (magenta bars) compared to air (blue bars). Images next to the graphs show representative maximum projections of imaged cells (blue border = air, magenta border = 20% CO2). All scale bars represent 20 µm. CaMPARI-2: calcium-modulated photoactivatable ratiometric integrator 2; CAPA: capability neurons; CO2: carbon dioxide; CRZ: corazonin neurons; DH44: diuretic hormone 44 neurons; DMS: Drosophila myosuppressin neurons; EH: eclosion hormone neurons; HugRG: hugin neurons innervating ring gland; IPCs: insulin-producing cells; ITP: ion transport peptide neurons; n.s.: not significant; PTTH: prothoracicotropic hormone neurons; RPN: ring gland projection neuron.
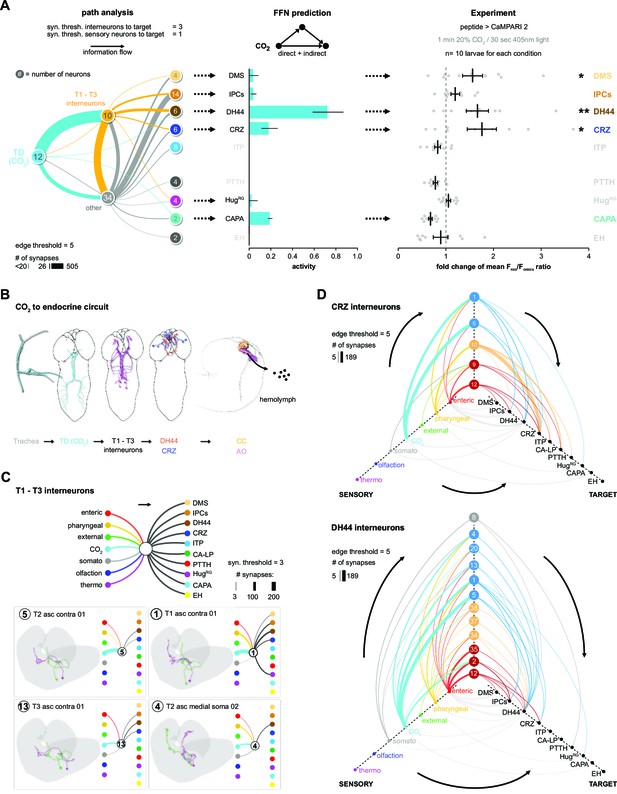
CO2-dependent pathway from TD neurons to RPNs.
(A) Comparison of underlying connectivity of TD (CO2) neurons via interneurons to the RPNs, with the predicted outcome of mean activity (with an activation factor of 2; when more than 5% of presynaptic neurons are active, interneurons become activated up to an activity of 50%) of RPNs, and the outcome of CaMPARI-2 CO2 experiments. FFN diffusion model reliably shows modulation of the RPNs. Please note that the circled numbers in the path analysis refer to the total number of neurons, not neuron identification number. (B) Using the combination of connectivity, prediction, and functional imaging experiments, a new sensory to endocrine neural circuit can be derived. TD (CO2) neurons at the trachea respond to CO2 levels and communicate predominantly via a core set of thoracic interneurons to DH44 and CRZ, which show release sites in the CC and AO. (C) Connectivity of the single thoracic interneurons (hemilateral pairs) to presynaptic sensory origins and to the distinct postsynaptic RPN groups. Thoracic interneurons receive additionally other sensory modalities apart from TD (CO2) neurons and target different combinations of RPNs. (D) CRZ interneurons: hive plot showing the polysynaptic pathways from all sensory origins to all RPN target groups using the interneurons (synaptic threshold = 3) that target CRZ. Main sensory origins are enteric, pharyngeal, and CO2. DH44 interneurons: TD (CO2) represent the most dominant polysynaptic path from sensory origins to DH44. Note that monosynaptic connections from sensory neurons to RPNs are shown in gray. AO: aorta; CA-LP: corpus allatum innervating neurosecretory neurons of the lateral protocerebrum; CaMPARI-2: calcium-modulated photoactivatable ratiometric integrator 2; CAPA: capability neurons; CC: corpora cardiaca; CO2: carbon dioxide; CRZ: corazonin neurons; DH44: diuretic hormone 44 neurons; DMS: Drosophila myosuppressin neurons; EH: eclosion hormone neurons; FFN: feed forward network; HugRG: hugin neurons innervating ring gland; IPCs: insulin-producing cells; ITP: ion transport peptide neurons; PTTH: prothoracicotropic hormone neurons; RPNs: ring gland projection neurons; ssTEM: serial section transmission electron microscope; TD: tracheal dendritic neurons.
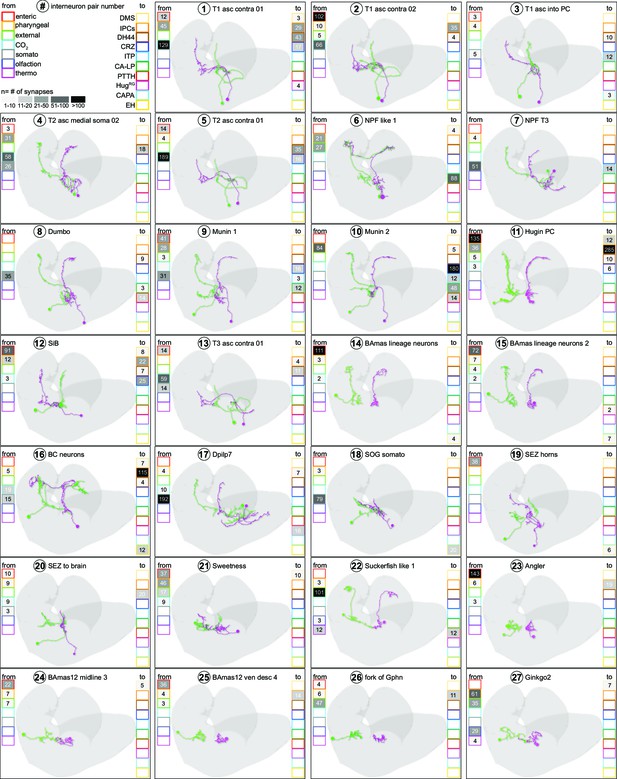
Neuron catalogue of interneurons receiving sensory input.
Absolute synapse numbers from sensory neurons to interneurons, and from interneurons to RPN target groups, are displayed in color-coded boxes. Strength of synaptic contacts is heatmap coded in grayscale. For abbreviations, see Figure 3—figure supplement 1.
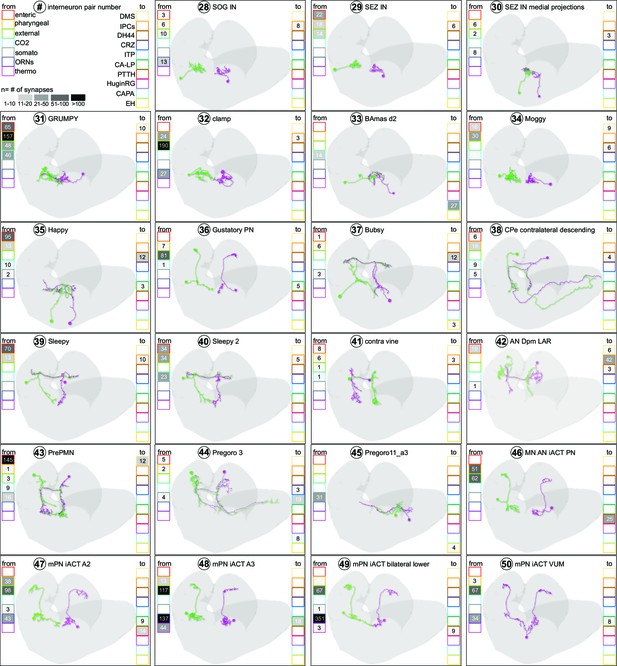
Neuron catalogue of interneurons receiving sensory input.
Absolute synapse numbers from sensory neurons to interneurons, and from interneurons to RPN target groups, are displayed in color-coded boxes. Strength of synaptic contacts is heatmap coded in grayscale. For abbreviations, see Figure 3—figure supplement 1.
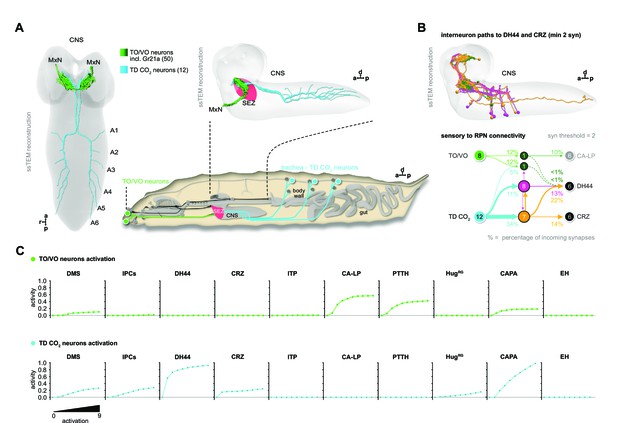
Impact of Gr21a candidate neurons on modulation of RPN activity.
(A) Ventral and lateral 3D representations of reconstructed chemosensory neurons of the TO, VO (Miroschnikow et al., 2018), and TD (CO2) neurons in the CNS (top left). Note that Gr21a is expressed in TO ganglion cell 6 and projects via the MxN to the SEZ in the brain (Kwon et al., 2011). Schematic side view of a Drosophila larva. Colored dots and lines represent the location and projection of TOG and TDs (CO2). (B) Shortest path (2-hops) analysis reveals 17 interneurons relaying TO, VO, and TD (CO2) sensory information to DH44 and CRZ neuroendocrine RPNs. Path threshold = minimum two synapses. Neuron colors correspond to respective nodes in connectivity graph. Note that only 8 of the 50 TO and VO neurons are weakly connected to DH44 and CRZ via two interneurons. Percentage represents the fraction of synapses from upstream neurons (arrows). Numbers in circles represent number of cells. (C) Activation of all neurons of the TOG and VO in the FFN, using all reconstructed interneurons to the RPNs, did not alter modulation of the strong CO2-responsive RPNs (e.g., DH44 and CRZ). Instead, TOG/VO neurons are capable of modulating the activity of a different set of RPNs, namely PTTH and CA-LP. Slight activity modulation was observed for DMS and CAPA. Thus it seems unlikely, given the connectome-based modulation of RPNs via the FFN, that Gr21a neurons have an effect on RPN activity based on the CO2 pathway originating from TDs (CO2). A potential implication in terms of valence has to be determined in future experiments. a: anterior; CA-LP: corpus allatum innervating neurosecretory neurons of the lateral protocerebrum; CAPA: capability neurons; CO2: carbon dioxide; CRZ: corazonin neurons; d: dorsal; DH44: diuretic hormone 44 neurons; DMS: Drosophila myosuppressin neurons; EH: eclosion hormone neurons; FFN: feed forward network; HugRG: hugin neurons innervating ring gland; IPCs: insulin-producing cells; ITP: ion transport peptide neurons; MxN: maxillary nerve; p: posterior; PTTH: prothoracicotropic hormone neurons; r: right; RPNs: ring gland projection neurons; SEZ: subesophageal zone; ssTEM: serial section transmission electron microscope; TD: tracheal dendritic neurons; TO: terminal organ; TOG: terminal organ ganglion; VO: ventral organ.
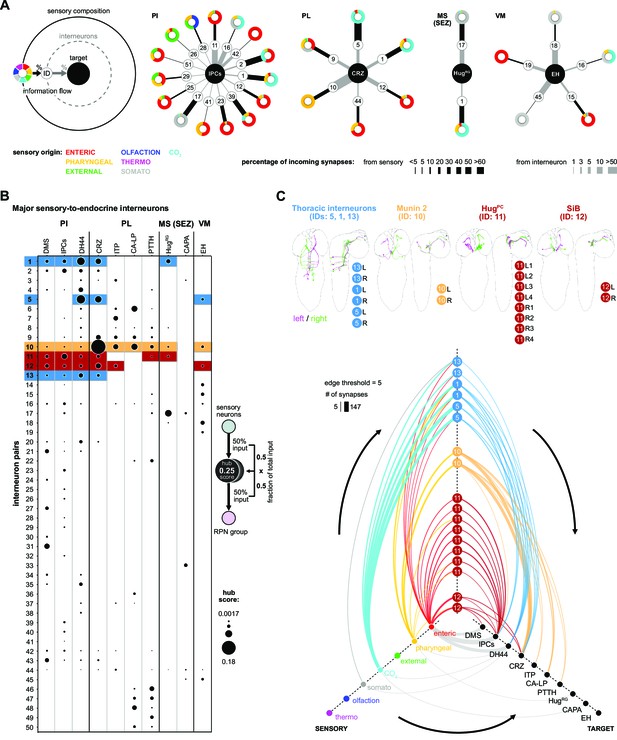
Interneurons and hub analysis of sensory to endocrine pathways.
(A) Schematic of graph representation. Outer ring represents the sensory composition of neurons targeting upstream neurons of RPNs. Synaptic threshold for upstream neurons of RPNs = 3. Line thickness to interneurons and targets represents the percentage of synaptic input. Striped ring represents the interneuron layer (black lined white circle). Inner ring represents target neurons (RPN peptide clusters). Sensory-interneuron circuits of neurons within the PI region. IPCs integrate mainly information from enteric sensory areas. Sensory-interneuron circuits within the PL region. CRZ integrates mainly CO2 and pharyngeal sensory information. Peptides of the MS (SEZ) or VM cluster show least number of sensory-interneuron input. HugRG neurons receive sensory information from two interneuron pairs integrating mainly somatosensory and CO2 sensory information. Please note different scaling for strength of connections between sensory origin to interneurons (black lines) and interneurons to target peptide groups (gray lines). (B) Dot plot showing the importance of interneurons acting as sensory to endocrine hub. Dot size was calculated using the fraction of total input an interneuron receives from sensory neurons multiplied by the fraction of total input this interneuron gives to an RPN output group. Colored backgrounds of dots are highlighted for (C). (C) Selected interneurons (highlighted in B) connecting the sensory system with RPNs. Thoracic interneurons receive sensory information from TD (CO2) neurons and target IPCs, DH44 and CRZ (hive plot, strongest connection = 147 synapses). Munin 2 interneurons connect CRZ, ITP, PTTH, and CA-LP RPNs with pharyngeal sensory neurons. HugPC connect the IPCs with enteric sensory neurons. SiB neurons also receive information from enteric origins but target DMS, IPCs, and CRZ. Edge threshold for hive plot = 5 synapses. CA-LP: corpus allatum innervating neurosecretory neurons of the lateral protocerebrum; CAPA: capability neurons; CO2: carbon dioxide; CRZ: corazonin neurons; DH44: diuretic hormone 44 neurons; DMS: Drosophila myosuppressin neurons; EH: eclosion hormone neurons; HugPC: hugin neurons innervating protocerebrum; HugRG: hugin neurons innervating ring gland; IPCs: insulin-producing cells; ITP: ion transport peptide neurons; MS (SEZ): medial subesophageal ganglion; PI: pars intercerebralis; PL: pars lateralis; PTTH: prothoracicotropic hormone neurons; RPNs: ring gland projection neurons; SiB: subesophageal zone into brain neurons; VM: ventromedial.
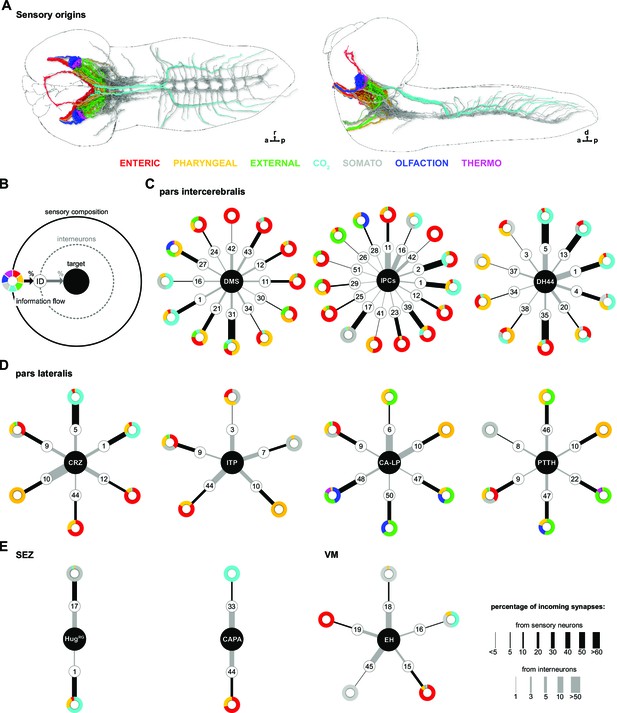
Sensory-interneuron paths to specific RPNs.
(A) Dorsal (left) and lateral (right) view of the larval CNS and all reconstructed sensory neurons used for analysis in this study (sensory neurons were pooled from published data of earlier studies; see main text). (B) Schematic of graph representation. Outer ‘ring’ represents the sensory composition of neurons targeting upstream neurons of RPNs. Line thickness to interneurons and targets represents the percentage of synaptic input. Striped ring represents the interneuron layer (black lined white circle). Inner ring = target neurons (RPN peptide clusters). (C) Sensory-interneuron paths of neurons within the PI region. IPCs and DMS integrate mainly information from enteric sensory areas. DH44 show most connections from TDs (CO2) via interneurons. (D) Sensory-interneuron paths within the PL region. Peptidergic groups show connectivity to less sensory-receiving interneurons. CA-LP and PTTH are the only RPNs from PL receiving sensory information from external sensory neurons and ORNs. CRZ integrate mainly CO2 and pharyngeal sensory information, while ITP integrate somatosensory and pharyngeal sensory information. (E) Peptides of the SEZ or VM cluster show least number of sensory-interneuron input. HugRG receive sensory information from two interneuron pairs integrating mainly somatosensory and CO2 sensory information; CAPA integrate CO2 and enteric information; EH integrate enteric and somatosensory neurons. Please note different scaling for strength of connections between sensory origin to interneurons (black lines) and interneurons to target peptide groups (gray lines). a: anterior; CA-LP: corpus allatum innervating neurosecretory neurons of the lateral protocerebrum; CAPA: capability neurons; CRZ: corazonin neurons; d: dorsal; DH44: diuretic hormone 44 neurons; DMS: Drosophila myosuppressin neurons; EH: eclosion hormone neurons; HugRG: hugin neurons innervating ring gland; IPCs: insulin-producing cells; ITP: ion transport peptide neurons; ORNs: olfactory receptor neurons; p: posterior; PI: pars intercerebralis; PL: pars lateralis; PTTH: prothoracicotropic hormone neurons; r: right; RPNs: ring gland projection neurons; SEZ: subesophageal zone; TD: tracheal dendritic neurons; VM: ventromedial cells.
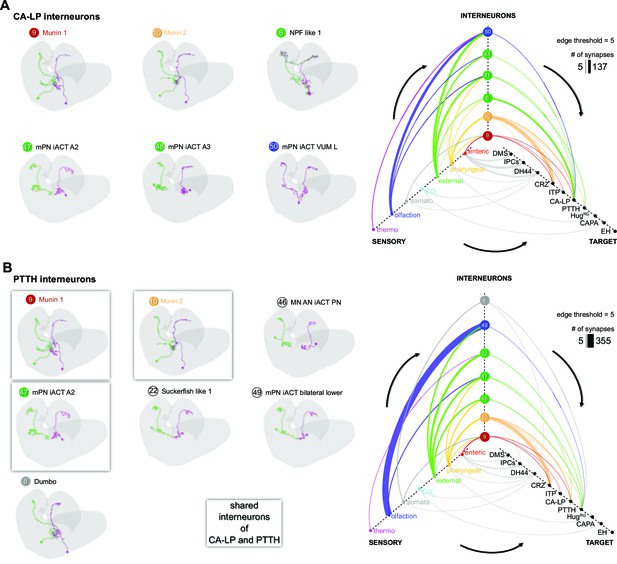
Sensory integration by CA-LP and PTTH RPNs.
(A) Upstream neurons of CA-LP (synaptic threshold = 3). CA-LP integrate mainly pharyngeal, external and olfactory sensory signals via their upstream neurons (edge threshold = 5, strongest synaptic connection has 137 synapses). (B) Upstream neurons of PTTH. PTTH RPNs share three interneurons with CA-LP RPNs (neuron IDs: 9, 10, and 47). PTTH integrates similar sensory inputs via interneurons as CA-LP (pharyngeal, external, and olfactory origins; edge threshold = 5, strongest synaptic connection has 355 synapses). CA-LP: corpus allatum innervating neurosecretory neurons of the lateral protocerebrum; CAPA: capability neurons; CRZ: corazonin neurons; DH44: diuretic hormone 44 neurons; DMS: Drosophila myosuppressin neurons; EH: eclosion hormone neurons; HugRG: hugin neurons innervating ring gland; IPCs: insulin-producing cells; ITP: ion transport peptide neurons; PTTH: prothoracicotropic hormone neurons; RPNs: ring gland projection neurons.
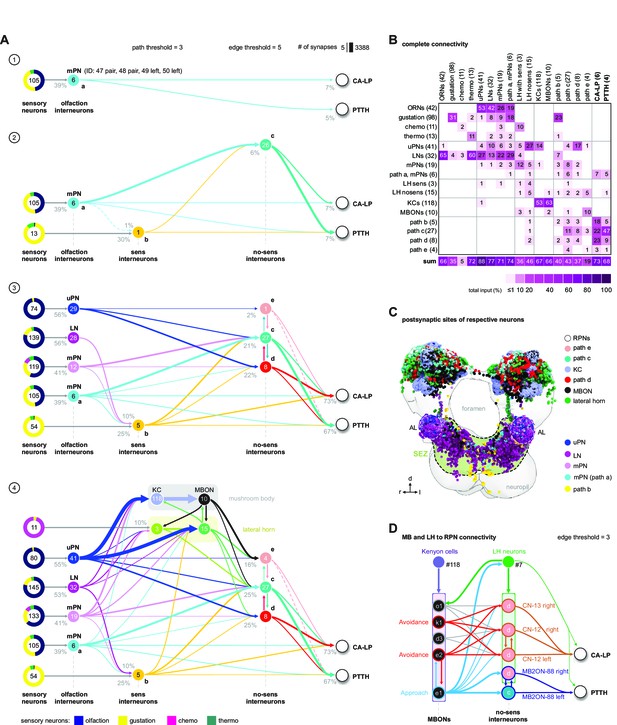
Olfactory pathways to neuroendocrine cells.
(A) Panel 1. Shortest-path (2-hops) analysis reveals six mPNs (Berck et al., 2016) relaying olfactory sensory information (ORNs) to CA-LP and PTTH neuroendocrine RPNs (path a). Synaptic threshold is 1 from ORNs to interneurons and minimum three synapses from interneurons to targets. Percentage represents the fraction of synapses from upstream neurons (arrows). Colored pie charts represent the percentage composition of total synaptic input from sensory neurons onto interneurons. Colors of pie charts correspond to the respective sensory compartments as in Miroschnikow et al., 2018. Panel 2. Alternative paths (paths b and c) from mPNs converging onto the same RPNs (CA-LP and PTTH) with a synaptic threshold of minimum 3. One interneuron (path b, neuron ID: 22) receives information from non-olfactory sensory neurons (sens interneurons). All 26 interneurons of path c have no connectivity to sensory neurons (no-sens interneurons). Note that path b in turn uses four neurons of path c as alternative routes to PRNs (syn.thresh. = 2). Panel 3. Adding more neurons that integrate olfactory information (olfaction interneurons): uPN, mPN, and LN (Berck et al., 2016) increases the number of possible and combinatorial paths (3-hops, syn. threshold = 3) to RPNs. Note that olfaction interneurons integrate information from both olfactory sensory neurons as well as from non-olfactory sensory neurons. Edges between olfaction interneurons are not shown. Panel 4: Superimposition of lateral horn, mushroom body KC, and MBON onto the ORN-to-RPN circuit. Note that all sensory information-integrating neurons, mushroom body, and lateral horn converge onto no-sens interneurons. (B) Adjacency matrix showing neurons of panel 4 in (A), color-coded by percentage of inputs on targets (columns). (C) Frontal view of the neuropil showing the distribution of presynaptic sites of all neurons (panel 4 in A) that act as paths for olfactory information to RPNs (CA-LP and PTTH). Each circle represents a synaptic site. Synapses are colored based on neuron class. The SEZ and AL are marked with dotted lines. (D) Representation and connectivity of KCs, MBONs, lateral horn neurons, convergence neurons, and MB2ONs (Eichler et al., 2017; Eschbach et al., 2020) that act as paths to CA-LP and PTTH neuroendocrine RPNs (synaptic threshold = 3). Convergence neurons and MB2ON correspond to path c and e neurons (no-sens interneurons) in (A). Edges of MBONs are colored based on their behavioral effect (avoidance, approach) (Eschbach et al., 2020). AL: antennal lobe; CA-LP: corpus allatum innervating neurosecretory neurons of the lateral protocerebrum; KC: Kenyon cells; LH: lateral horn; LN: local neurons; MBON: mushroom body output neurons; mPNs: multiglomerular projection neurons; ORNs: olfactory receptor neurons; PTTH: prothoracicotropic hormone neurons; RPNs: ring gland projection neurons; SEZ: subesophageal zone; uPN: uniglomerual projection neurons.
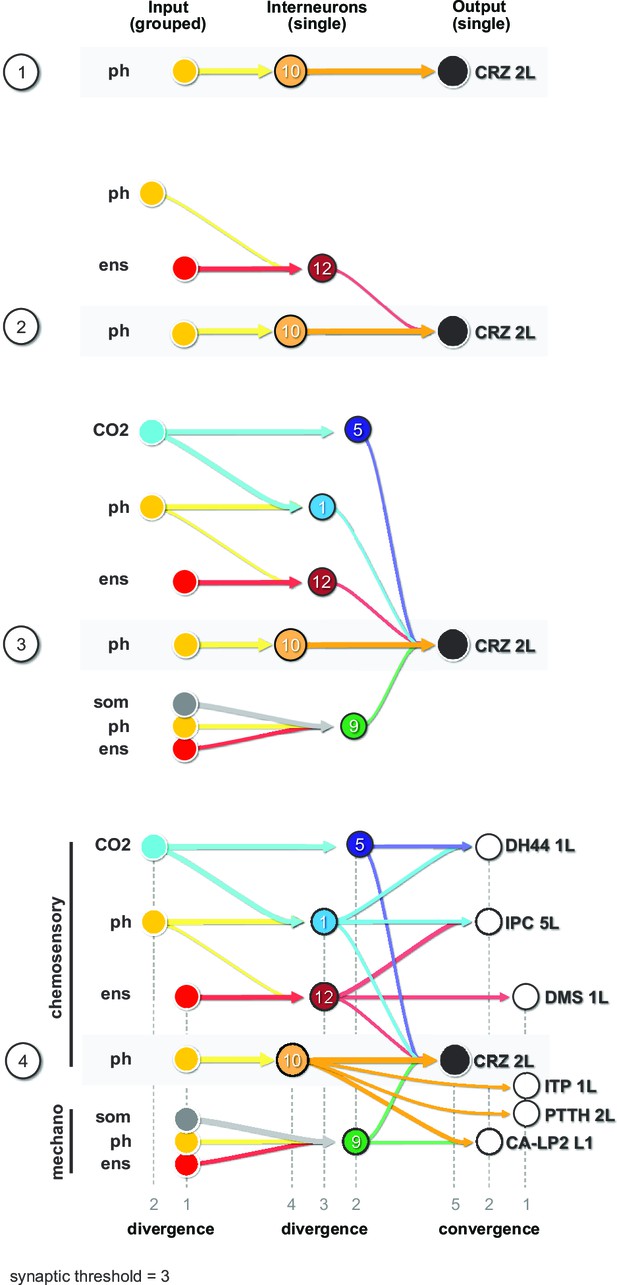
Diverging sensory and interneuronal paths converge onto a linked set of output neurons.
Circuit architecture common for all RPNs (CRZ single-cell example). 1: the strongest polysynaptic path based on hub analysis from pharyngeal sensory origin to CRZ output neuron via interneuron 10. 2: second interneuron (12) integrating enteric information and different pharyngeal information, converging onto CRZ output neuron. 3: all interneurons of one CRZ output neuron integrating multiple sensory origins and converging onto one single output. 4: concept of divergence and convergence in the neuroendocrine connectome. Sensory neurons diverge/converge onto distinct sets of interneurons. Interneurons diverge in varying synaptic strength onto distinct sets of linked RPN output neurons. Numbers at bottom show degree of convergence and divergence (e.g., interneuron 10 diverges to CRZ, ITP, PTTH, and CA-LP; all interneurons converge to CRZ; synaptic threshold = 3 for all connections). CA-LP: corpus allatum innervating neurosecretory neurons of the lateral protocerebrum; CO2: carbon dioxide; CRZ: corazonin neurons; DH44: diuretic hormone 44 neurons; DMS: Drosophila myosuppressin neurons; ens: enteric nervous system; IPCs: insulin-producing cells; ITP: ion transport peptide neurons; ph: pharynx; PTTH: prothoracicotropic hormone neurons; som: somatic; RPNs: ring gland projection neurons.
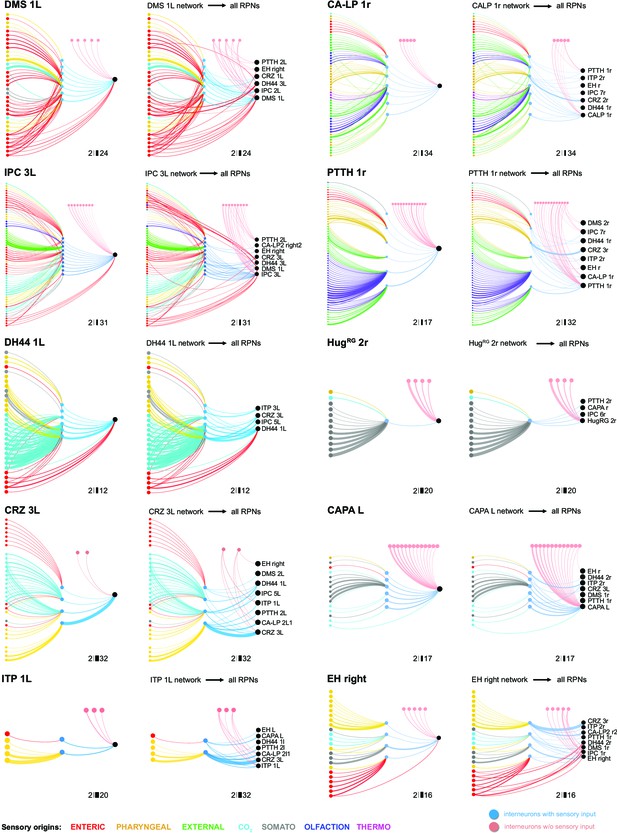
Diverging single-cell RPN circuits.
Shown are, based on single-output RPN cell, circuits from sensory origins (single cells) via interneurons (single cells) to output (single cell). Included are also interneurons (upstream of one particular RPN), which do not receive input from sensory neurons (pink interneurons). These are shown on the left panel for each RPN set. Converging paths onto a specific output RPN automatically result in connections to different sets of RPNs (‘linked RPNs’). These linked RPN paths are shown on the right panel for each RPN set. Using DMS 1L as an illustration, a series of parallel paths from different sensory neurons converge, either directly or via a layer of interneurons (presented vertically), onto a single-output cell. In addition, there is another layer of interneurons, but that have no direct contact to sensory neurons (presented horizontally), that also converge onto the same output cell. These pathways are then found to converge, in varying strengths, onto additional RPNs (in this case, a total of six cells), thus forming a linked set of outputs. The same principle applies to RPNs with no direct sensory connections, such as CRZ. For abbreviations, see Figure 6—figure supplement 2.
Tables
Genotypes of experimental flies.
Figure | Genotypes | Chr. |
---|---|---|
Figure 3C (antibody staining, from left to right) | w; P{UAS-mCD8.mRFP.LG}18a; P{Ms-GAL4.P} | X; 2; 3 |
w; P{Ilp2-GAL4.R}2/ P{UAS-mCD8.mRFP.LG}18a | X; 2 | |
w; P{UAS-mCD8.mRFP.LG}18a; P{Dh44-GAL4.TH}2 M | X; 2; 3 | |
w; P{UAS-mCD8.mRFP.LG}18a; P{Crz-GAL4.391}4 M | X; 2; 3 | |
w; TI{2A-lexA::QF}ITP2A-lexA.no1; P{13XLexAop2-IVS-myr::GFP}attP2 | X; 2; 3 | |
w; P{Burs-GAL4.TH}4 M/ P{UAS-mCD8.mRFP.LG}18a | X; 2 | |
w; P{UAS-mCD8.mRFP.LG}18a; P{Ptth-GAL4.M}45, P{Ptth-GAL4.M}117b | X; 2; 3 | |
w; P{UAS-mCD8.mRFP.LG}18a; P{GMR17B03-GAL4}attP2 | X; 2; 3 | |
w; PBac{IT.GAL4}CG79970714-G4/ P{UAS-mCD8.mRFP.LG}18a | X; 2 | |
w; P{GAL4-Eh.2.4}C21P/ {UAS-mCD8.mRFP.LG}18a | X; 2 | |
Figure 4B (CaMPARI analysis, from top left to bottom right) | w; P{Ms-GAL4.P}/ PBac{UAS-CaMPARI2}VK00005 | X; 3 |
w; P{Ilp2-GAL4.R}2; PBac{UAS-CaMPARI2}VK00005 | X; 2; 3 | |
w; P{Dh44-GAL4.TH}2 M/ PBac{UAS-CaMPARI2}VK00005 | X; 3 | |
w; P{Crz-GAL4.391}4 M/ PBac{UAS-CaMPARI2}VK00005 | X; 3 | |
w; TI{2A-GAL4}ITP2A-D.GAL4; PBac{UAS-CaMPARI2}VK00005 | X; 2; 3 | |
w; P{Ptth-GAL4.M}45, P{Ptth-GAL4.M}117b/ PBac{UAS-CaMPARI2}VK00005 | X; 3 | |
w; P{GMR17B03-GAL4}attP2/ PBac{UAS-CaMPARI2}VK00005 | X; 3 | |
w; PBac{IT.GAL4}CG79970714-G4; PBac{UAS-CaMPARI2}VK00005 | X; 2; 3 | |
w; P{GAL4-Eh.2.4}C21P; PBac{UAS-CaMPARI2}VK00005 | X; 2; 3 | |
Figure 2—figure supplement 1B,C | Same genotypes as in Figure 3C | |
Figure 2—figure supplement 2B | w; TI{2A-lexA::QF}ITP2A-lexA.no1; P{13XLexAop2-IVS-myr::GFP}attP2 | X; 2; 3 |
Figure 2—figure supplement 3B | w; P{UAS-mCD8.mRFP.LG}18a; P{Crz-GAL4.391}4 M | X; 2; 3 |
Figure 2—figure supplement 3C | w; P{CrzR-GAL4.3.5.S}T11A/ P{UAS-mCD8.mRFP.LG}18a | X; 2 |
Figure 2—figure supplement 3D | y, w, P{UAS-myrGFP.QUAS-mtdTomato-3xHA}su(Hw)attP8; P{trans-Tango}attP40; P{Crz-GAL4.391}4 M | X; 2; 3 |
Figure 3—figure supplement 2A | w; P{UAS-mCD8.mRFP.LG}18a; PBac{IT.GAL4}lqfR0260-G4 | X; 2; 3 |
Figure 3—figure supplement 2B,C | w; P{UAS-CaMPARI}attP40; PBac{IT.GAL4}lqfR0260-G4 | X; 2; 3 |
Figure 3—figure supplement 2D (antibody staining, from left to right) | w; P{Gr21a-Mmus\Cd8a.GFP}2 | X; 2 |
w; P{Gr21a-GAL4.C}133t52.1/ P{10XUAS-mCD8::GFP}attP2 | X; 3 | |
w; P{Gr21a-GAL4.C}133t1.2/ P{10XUAS-mCD8::GFP}attP2 | X; 3 |
Primer sequences to generate homology arms.
Forward primer sequence | Revers primer sequence | |
---|---|---|
5´HA no1 | gcatgcACGCGCTGTTAATCAAAT | gcggccgcACGCTGTAAGGAACACTGATG |
5´HA no2 | gcatgcCGCTGTCATCGCTGTAATTC | gcggccgcGTCCCGCGATCATTTTCC |
3´HA no1 | ggcgcgccTCAAGGCAAGGCCGTCC | ggtaccCGAATTAAATTTGGGCGTTT |
3´HA no2 | ggtaccCTTCGGTTGTTTCTGAACTTTATG | actagtTCTCCCACTCCCCAATTATG |
Additional files
-
Supplementary file 1
Statistics table.
Green to red ratios in CaMPARI measurements. For experiments and use of statistical tests, see Materials and methods.
- https://cdn.elifesciences.org/articles/65745/elife-65745-supp1-v1.docx
-
Transparent reporting form
- https://cdn.elifesciences.org/articles/65745/elife-65745-transrepform1-v1.docx