Doublecortin engages the microtubule lattice through a cooperative binding mode involving its C-terminal domain
Figures
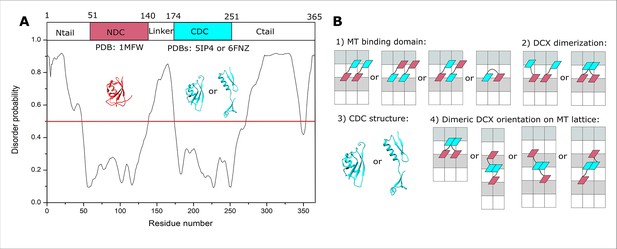
Doublecortin (DCX) structure and lattice interaction options.
(A) The disordered regions of DCX sequence predicted using PrDOS (Ishida and Kinoshita, 2007). (B) Schematic representations of the four orientational challenges to the elucidation of the DCX–microtubule (MT)-binding mode. In each case, a minimal DCX construct is shown, comprising NDC (red), linker (black), and CDC cyan. α- and β-tubulin are shown with white and light gray rectangles, respectively.
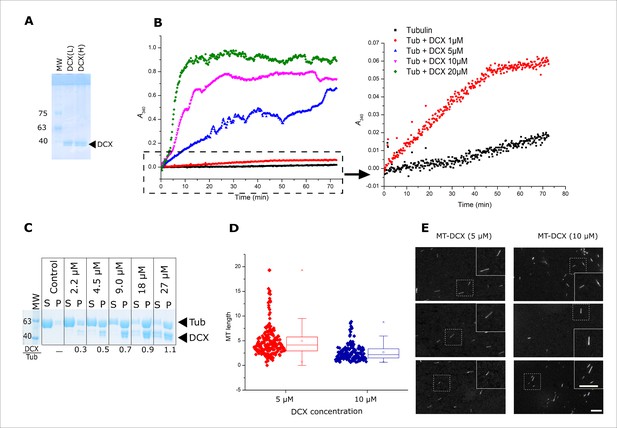
The evaluation of purity and activity of in house purified Doublecortin.
(A) The purity of DCX-Light and DCX-Heavy isotopically labeled sample was evaluated on 8% SDS-PAGE gel. (B) Turbidity measurement at 340 nm versus time for Tubulin (10 μM) with different concentrations of DCX (1, 5, 10 and 20 μM) in the presence of 1mM GTP. Samples containing Tubulin with even low DCX concentrations produced a significant augmentation in scattering, confirming the formation of microtubules (MTs). The right graph is the zoomed-in view of the negative control containing Tubulin (10 μM) + GTP (1 mM ) in black and Tubulin + DCX (1 μM) + GTP (1 mM ) in red. (C) MT polymerization in the presence of different concentrations of DCX (2.5-30 μM). The tested Tubulin concentration was 10 μM and GTP concentration was 1 mM. The control lane is devoid of DCX. (S) denotes supernatant and (P) denotes pellet. (D) The length distribution of MTs polymerized in the presence of 5 and 10 μM DCX concentrations (the average MT length was 5.0±3.3 and 2.6±1.7 µm, respectively). There is a significant difference between the two populations at p = 0.001. The horizontal line indicates the median value, the box indicates the 25th and 75th percentiles (N~120). (E) Fluorescence microscopy evaluation of MTs polymerized in the presence of DCX at 5 and 10 μM concentrations. No bundling was observed. Scale bar = 10 µm.
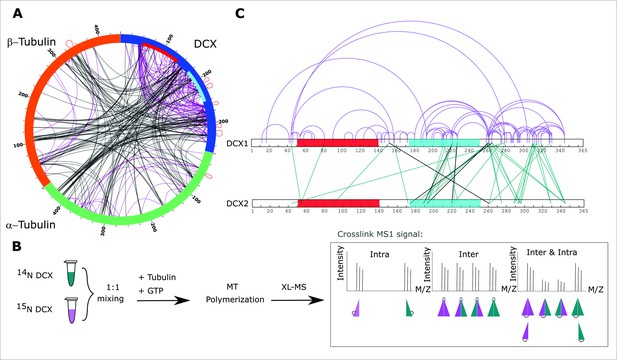
Crosslinking mass spectrometry analysis of Doublecortin (DCX).
(A) Two-dimensional crosslinking map linking α−β-tubulin and DCX at specific residues. NDC and C-terminal DC (CDC) domains of DCX sequence are shown with red and cyan, respectively. Intraprotein crosslinking sites are shown in black and interprotein crosslinks in purple. A subset of interprotein crosslinking sites observed between peptides with a shared sequence are shown in red loops. The crosslinking map is produced using xVis (Grimm et al., 2015). (B) Inter- and intra-DCX crosslinking sites differentiated using a protein isotopic labeling technique. Three categories are defined, where the ratios of intensities for the inner and outer doublet in the mixed state are variable. The crosslinking map is produced using xiNET online tool (Combe et al., 2015). (C) Two-dimensional crosslinking map linking DCXs at specific residues. NDC and CDC domains of DCX are shown in red and cyan, respectively. Shared inter and intra XLs are shown as inter (green) and intra (purple) DCX crosslinks. Unique inter-DCX crosslinking sites are shown in black. In all cases, when there is ambiguity in the crosslinking site, a single crosslinking site pair was used for visualization.
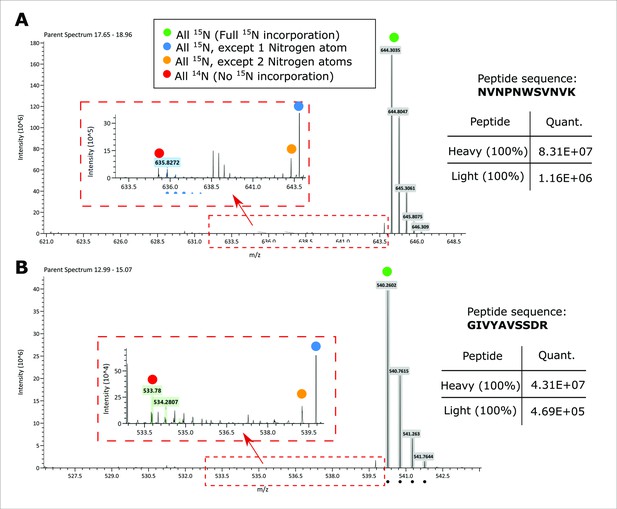
15N incorporation in purified heavy labeled DCX was assessed by LC-MS analysis.
The two MS1 scans for two candidate DCX peptides are shown as the examples. The highlighted mass to charge ratios (M/Zs) are corresponding to a range of species from fully 14N to fully 15N species. The corresponding sample area for light and heavy species is shown in the inserted tables.
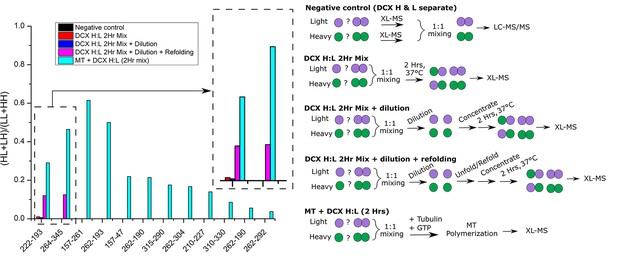
Monitoring the ratio of heterogenous species (Heavy-Light:HL and Light-Heavy:LH) to the homogenous species (Light-Light:LL and Heavy-Heavy:HH), for different crosslinked peptides.
The X-axis IDs are crosslinked residues. The experimental procedure for each sample is schematically represented in right. Only two peptides were shared among different samples (221-193 an 264-345). The two peptides were also observed in the H/L sample (negative control) but no HL or LH species were observed.
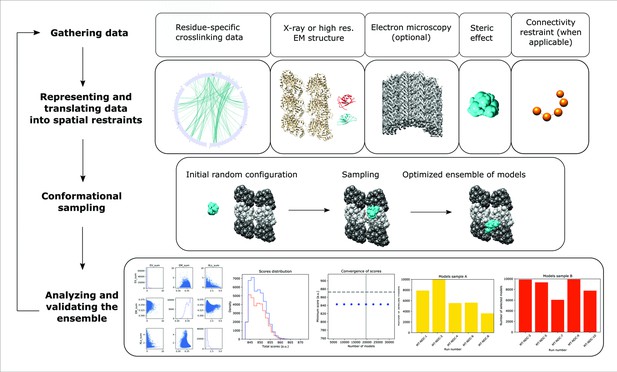
Integrative structure determination of microtubule (MT)–Doublecortin (DCX), using four stage workflow.
(1) Data gathering, including chemical crosslinking, atomic structures (MT structure, PDB 6EVZ; NDC structure, DCX component of PDB 4ATU; CDC structure, PDB 5IP4), and cryo-EM map EMD 2095. (2) Representation of subunits and translation of the data into spatial restraints, including crosslinking distance restraints, atomic structures, cryo-EM map, and physical restraints (steric effect or excluded volume restraint and connectivy restraint). (3) Monte-Carlo conformational sampling to obtain an ensemble of structures needed to satisfy the input data. (4) Statistical analysis of the computed models and clustering the sample models into distinct groups of structures, followed by the analysis in terms of accuracy and precision.
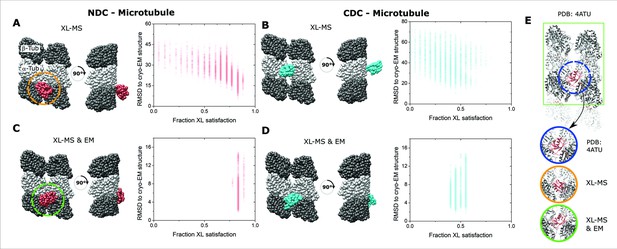
Integrative structural modeling for NDC/CDC–microtubule (MT).
(A) The centroid structure for the main cluster of models produced by IMP for NDC–MT guided exclusively by crosslinking mass spectrometry (XL-MS) restraints. (B) The centroid structure for the main cluster of models produced by IMP for CDC–MT guided exclusively by XL-MS restraints. (C) The centroid structure for the main cluster of models produced by IMP NDC–MT for XL-MS restraints combined with the EM density map. (D) The centroid structure for the main cluster of models produced by IMP CDC–MT guided by XL-MS restraints and combined with the EM density map. The fractional crosslink satisfaction (defined as <35 Å) versus RMSD to the canonical binding site (PDB 4ATU) for all models in the main structural cluster is presented for each modeling scenario. α- and β-tubulin are shown as light and dark gray, respectively. NDC is shown in red, and CDC in cyan. (E) Expansions of the NDC orientations from modeling, compared to cryo-EM based NDC–MT structure (PDB 4ATU).
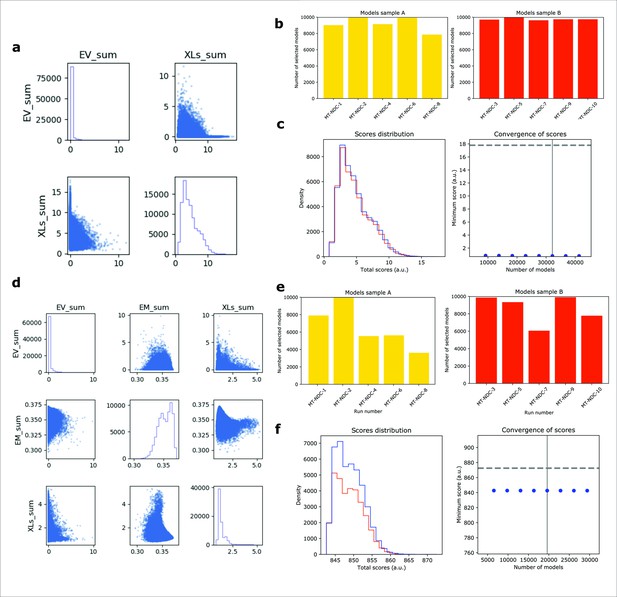
IMP analysis output for MT-NDC.
(a) The distribution of scores for the models computed for MT-NDC, using only crosslinking data (500,000 models). (b) The number of models randomly selected for model samples A (yellow) and sample B (red), from 10 different modeling runs. (c) The score convergence for models in samples A and B. The nonparametric Kolmogorov–Smirnov two-sample test (two-sided) indicates that the difference between the two score distributions is insignificant, the magnitude of the difference is small, as demonstrated by the Kolmogorov–Smirnov two-sample test statistic, D, of 0.006. Thus, the two score distributions are effectively equal. (d) The distribution of scores for the models computed for MT-NDC, using both crosslinking data and EM map (500,000 models). (e) The number of models randomly selected for model samples A (yellow) and B (red), obtained from 10 different modeling runs. (f) The score convergence for the models in samples A and B. The nonparametric Kolmogorov–Smirnov two-sample test (two-sided) indicates that the difference between the two score distributions is insignificant, the magnitude of the difference is small, as demonstrated by the Kolmogorov–Smirnov two-sample test statistic, D, of 0.031. Thus, the two score distributions are effectively equal.
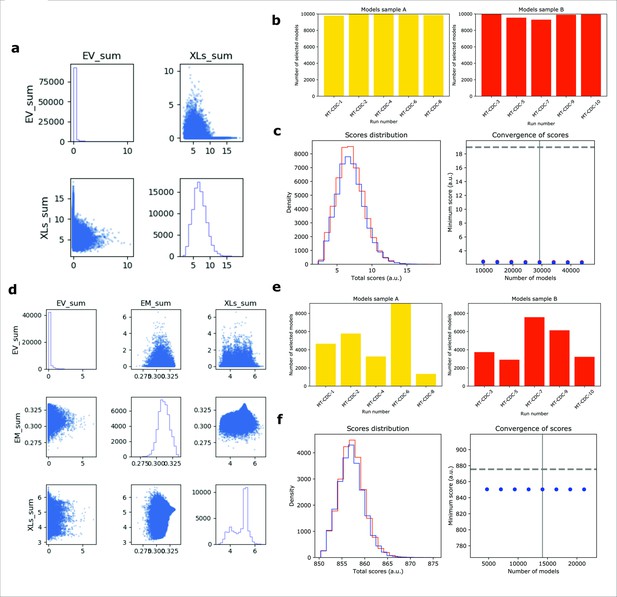
IMP analysis output for MT-CDC.
(a) The distribution of scores for the models computed for MT-CDC, using only crosslinking data (500,000 models). (b) The number of models randomly selected for model samples A (yellow) and B (red), from 10 different modeling runs. (c) The score convergence for models in samples A and B. The nonparametric Kolmogorov–Smirnov two-sample test (two-sided) indicates that the difference between the two score distributions is insignificant, the magnitude of the difference is small, as demonstrated by the Kolmogorov–Smirnov two-sample test statistic, D, of 0.010. Thus, the two score distributions are effectively equal. (d) The distribution of scores for the models computed for MT-CDC, using both crosslinking data and EM map (500,000 models). (e) The number of models randomly selected for model samples A (yellow) and B (red), from 10 different modeling runs. (f) The score convergence for models in samples A and B. The nonparametric Kolmogorov–Smirnov two-sample test (two-sided) indicates that the difference between the two score distributions is insignificant, the magnitude of the difference is small, as demonstrated by the Kolmogorov–Smirnov two-sample test statistic, D, of 0.034. Thus, the two score distributions are effectively equal.
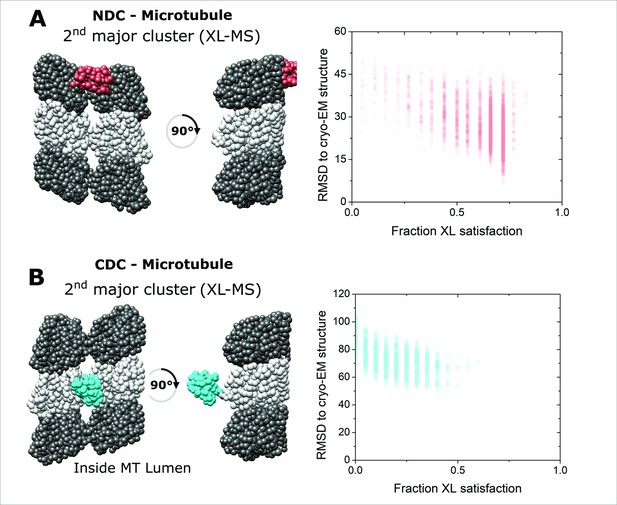
Integrative structural modeling for NDC-MT and CDC-MT (2nd major clusters).
The centroid structure for the 2nd major cluster of models produced by IMP for NDC-MT (A) and CDC-MT (B) guided exclusively by XL-MS restrains; alpha-Tubulin and beta-Tubulin are shown as light and dark grey, respectively. NDC is shown in red, and CDC is shown in cyan. The fraction crosslink satisfaction (defined as <35 Å) versus RMSD to canonical binding site (PDB 4ATU) for all models present in the main structural cluster is presented for each modeling scenarios.
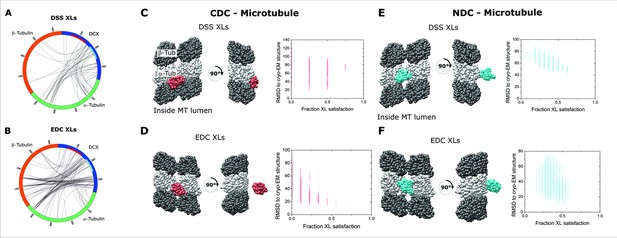
Crosslinking-mass spectrometry analysis of DCX-MT using conventional crosslinkers.
Two-dimensional crosslinking map linking alpha/beta-Tubulin and DCX at specific residues using (A) DSS and (B) EDC. NDC and CDC domains of DCX sequence are shown with red and cyan, respectively. A subset of inter-DCX crosslinking sites observed among peptides with a shared sequence are shown in red loops. The crosslinking map is produced using xVis online tool [10]. The centroid structure for the major cluster of models produced by IMP for NDC-MT guided exclusively by XL-MS restrains produced by DSS crosslinker (C) and EDC (D). The centroid structure for the major cluster of models produced by IMP for CDC-MT guided exclusively by XL-MS restrains produced by DSS crosslinker (E) and EDC (F); alpha-Tubulin and beta-Tubulin are shown as light and dark grey, respectively. NDC is shown in red, and CDC is shown in cyan. The fraction crosslink satisfaction (defined as <35 Å) versus RMSD to canonical binding site (PDB 4ATU) for all models present in the main structural cluster is presented for each modeling scenarios.
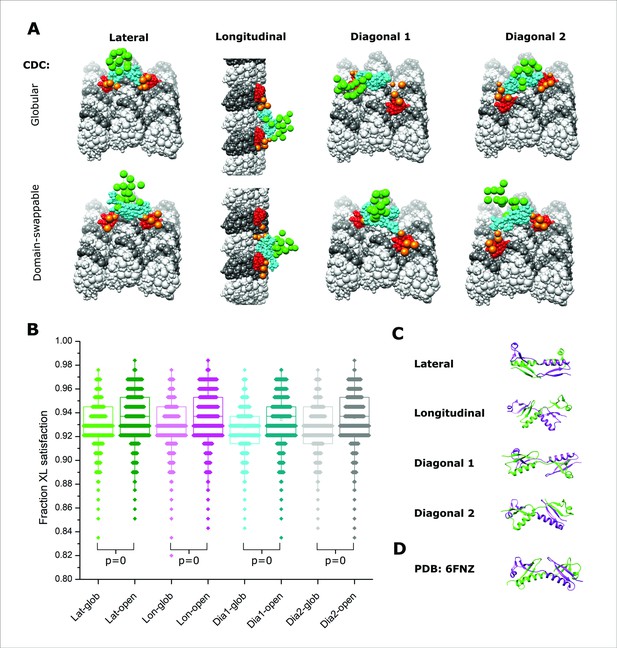
Integrative modeling of Doublecortin (DCX) self-assembly on microtubule (MT) lattice.
(A) The dimeric DCX–MT centroid model of the main clusters of models generated using only DCX–DCX crosslinking restraints. Four different relative positions of fixed NDC on the MT lattice were assessed (lateral, longitudinal, diagonal 1, and diagonal 2); α- and β-tubulin are shown as light and dark gray, respectively. NDC, linker, CDC, and C-tail regions are shown as red, orange, cyan, and green, respectively. CDC structure is represented as either globular or open (domain-swappable) conformations. (B) The fractional XL satisfaction (defined as <35 Å) for the main cluster of models generated for each modeling scenario. (C) The relative orientation of the dimeric CDC structures in the centroid model of the main cluster using the domain swappable CDC conformation; green is CDC (monomer 1) and purple is CDC (monomer 2). (D) The crystal structure of domain-swapped CDC dimer (PDB 6FNZ).
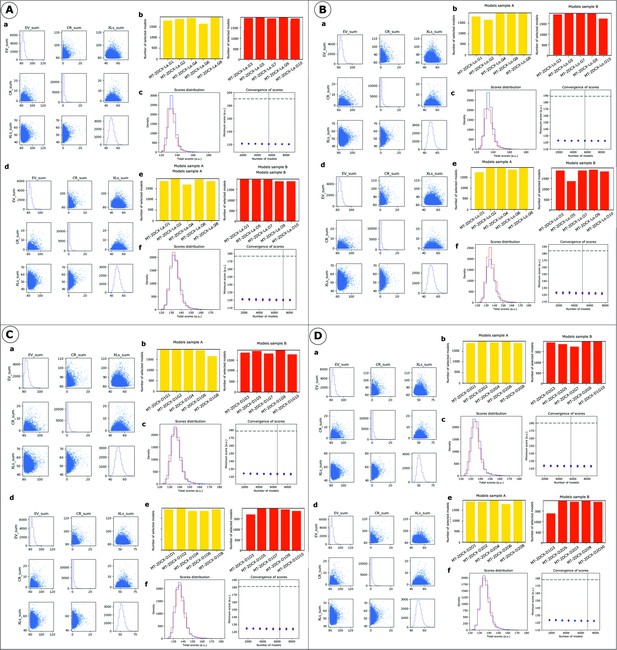
IMP analysis output for MT-dimeric DCX using DCX-DCX crosslinking data, where the NDCs were fixed at lateral (A), longitudinal (B), diagonal 1 (C) and diagonal 2 (D) relative positions on the MT lattice.
(a) The distribution of scores for the models computed for MT-dimeric DCX using the globular CDC structure (100,000 models). (b) The number of models randomly selected for model samples A (yellow) and B (red), from 10 different modeling runs. (c) The score convergence for the models in sample A and B. (d) The distribution of scores for the models computed for MT-dimeric DCX using the open CDC structure (100,000 models). (e) The number of models randomly selected for model samples A (yellow) and sample B (red), from 10 different modeling runs. (f) The score convergence for models in sample A and B. The nonparametric Kolmogorov–Smirnov two-sample test (two-sided) indicates that the difference between the two score distributions is insignificant for each modeling run, as the magnitude of the difference is small, demonstrated by the Kolmogorov–Smirnov two-sample test statistic for each modeling run (lateral-globular: 0.010, lateral-open: 0.013, longitudinal-globular:0.014, longitudinal-open: 0.011, diagonal1-globular:0.023, diagonal1-open:0.015, diagonal 2-globular:0.019, and diagonal 2-open:0.014.) Thus, the two score distributions are effectively equal for each modeling run.
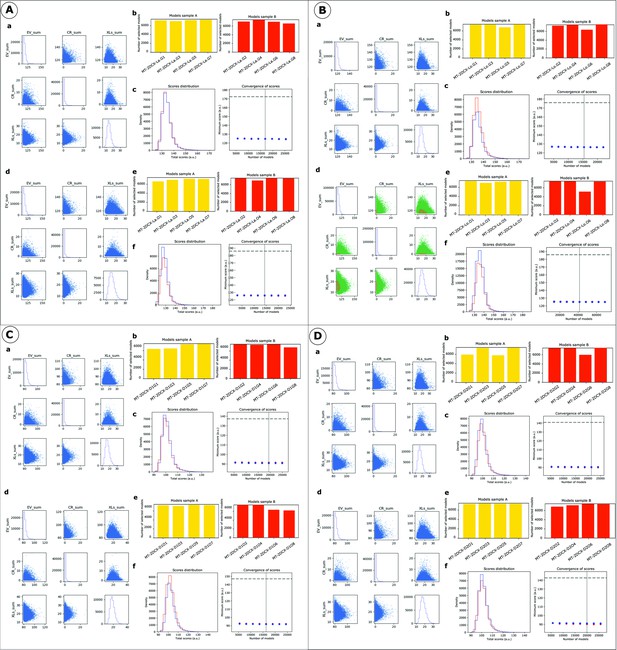
IMP analysis output for MT-dimeric DCX using MT-DCX and DCX-DCX crosslinking data, where the NDCs were fixed at lateral (A), longitudinal (B), diagonal 1 (C) and diagonal 2 (D) relative positions on MT lattice.
(a) The distribution of scores for the models computed for the MT-dimeric DCX using globular CDC structure (320,000 models). (b) The number of models randomly selected for model samples A (yellow) and B (red), from 10 different modeling runs. (c) The score convergence for models in sample A and B. (d) The distribution of scores for all the models computed for MT-dimeric DCX using the open CDC structure (320,000 models). (e) The number of models randomly selected for model samples A (yellow) and B (red), from 10 different modeling runs. (f) The score convergence for models in samples A and B. The nonparametric Kolmogorov–Smirnov two-sample test (two-sided) indicates that the difference between the two score distributions is insignificant for each modeling run, as the magnitude of the difference is small, demonstrated by the Kolmogorov–Smirnov two-sample test statistic for each modeling run (lateral-globular: 0.010, lateral-open: 0.010, longitudinal-globular:0.009, longitudinal-open:0.006, diagonal1-globular: 0.007, diagonal1-open:0.010, diagonal 2-globular:0.016, and diagonal 2-open: 0.010). Thus, the two score distributions are effectively equal for each modeling run.
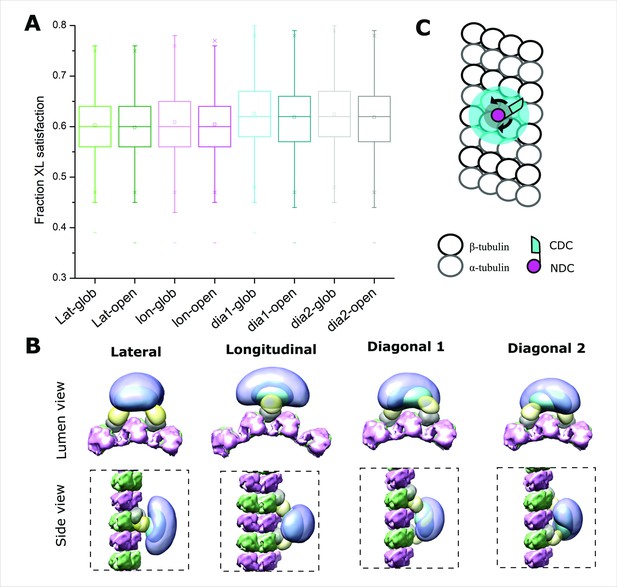
Positional evaluation of dimeric Doublecortin (DCX) on microtubule (MT) lattice.
(A) The fractional XL satisfaction for the main cluster of models generated for dimeric DCX–MT, employing all crosslinking restraints. The four different relative positions of fixed NDC on the MT lattice and the two CDC conformations were assessed. (B) The density maps corresponding to the main cluster of the dimeric DCX–MT model, using all crosslinking restraints. The four different relative positions of fixed NDC on the MT lattice were assessed; α- and β-tubulin are shown in pink and green, respectively. NDC, linker, CDC, and C-tail are shown as gray, lemon, cyan, and light purple, respectively. (C) The DCX–MT interaction model showing the flexibility of the DCX structure on the MT lattice.
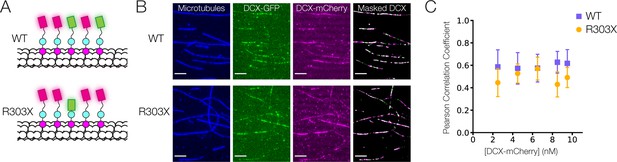
Cooperative binding of C-terminal tail truncated Doublecortin (DCX) on microtubule (MT).
(A) Schematic of DCX-mCherry engaging in cooperative binding with DCX-GFP or DCX-R303X-GFP on the MT lattice. (B) Images of taxol MTs, 0.5 nM DCX-GFP, 2.5 nM DCX-mCherry, and the color-combined image of DCX-GFP and DCX-mCherry restricted to a mask generated from the taxol MT image. (C) Mean Pearson correlation coefficient for the mCherry and GFP intensities of the masked images. At least three fields of view were collected for each condition.
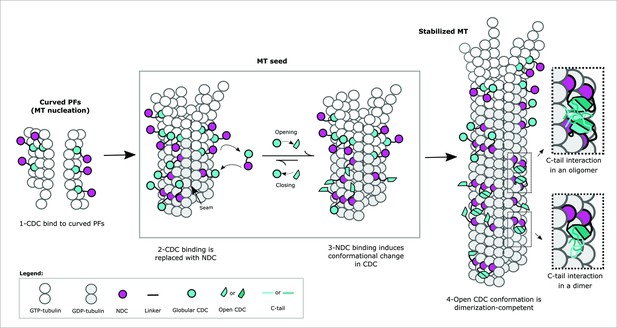
Mechanism of Doublecortin (DCX)-mediated microtubule (MT) nucleation and stabilization.
DCX stabilizes early GTP-tubulin oligomers through the CDC domain, which is then replaced with the NDC domain at the canonical binding site as during full MT assembly. NDC binding triggers a conformational change in CDC domain, which facilitates DCX self-association and prevents CDC from rebinding. The inserts illustrate the role of C-tail domain in the formation of either intermonomer interactions, interdimer interactions, or both.
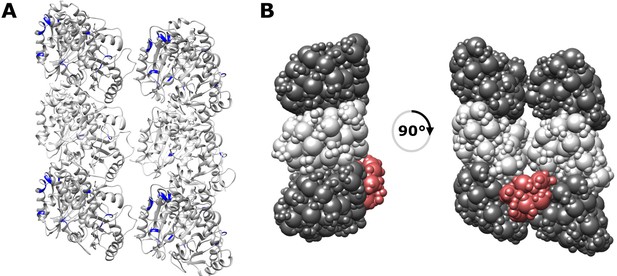
(A) Crosslink sites on the MT lattice repeat unit highlighted in blue, showing that some are indeed buried within the interprotofilament groove.
(B) Alternative representation showing the buried nature of NDC on the lattice.
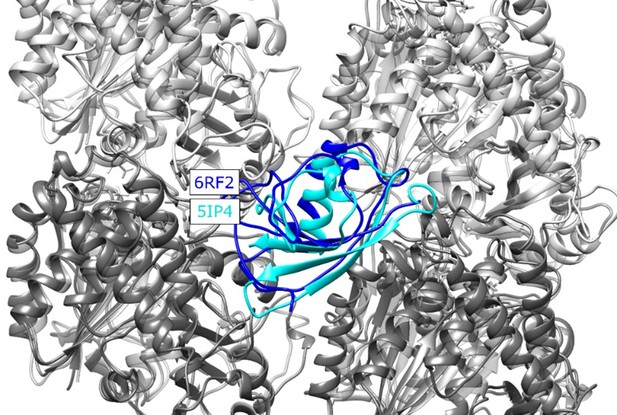
Structural alignment of the new MT-CDC structure (6RF2) to the one used in our study (5IP4), placed at the NDC binding site for illustration.
CDC structures corresponding to 6RF2 and 5IP4 are shown with blue and cyan, respectively, α tubulins are shown in light grey and β tubulins are shown in dark grey, The RMSD calculated for residues 178-251 of the 5IP4 and 6RF2 is 5.1 Å.
Tables
Reagent type (species) or resource | Designation | Source or reference | Identifiers | Additional information |
---|---|---|---|---|
Gene (Homo sapiens) | DCX | UniProtKB | O43602 | |
Gene (Sus scrofa) | α-Tubulin | UniProtKB | P02550 | |
Gene (Sus scrofa) | β-Tubulin | UniProtKB | P02554 | |
Strain, strain background (Escherichia coli) | Arctic Express (DE3) | Agilent | 230,192 | Electrocompetent cells |
Strain, strain background (Escherichia coli) | BL21(DE3) | New England BioLabs Inc. | C2527 | Mix and Go competent cells |
Antibody | Anti-His (Mouse monoclonal) | Applied Biological Materials | G020 | WB (1:1000) |
Antibody | Anti-β-tubulin (Mouse monoclonal) | Sigma-Aldrich | T4026 | (1:20 dilution in BRB80) |
Recombinant DNA reagent | DCX-WT-pHAT-HUS | Gift of Dr. Susanne Bechstedt | Human doublecortin (1–365) plasmid | |
Recombinant DNA reagent | DCX-WT-pHAT-HUGS | Gift of Dr. Susanne Bechstedt | GFP version of Human doublecortin (1–365) plasmid | |
Recombinant DNA reagent | DCX-R303X-pHAT-HUGS | Gift of Dr. Susanne Bechstedt | GFP version of Human doublecortin (1–302) plasmid | |
Recombinant DNA reagent | DCX-WT-pHAT-HUCS | Gift of Dr. Susanne Bechstedt | mCherry version of Human doublecortin (1–365) plasmid | |
Peptide, recombinant protein | Human doublecortin (1–365) | This paper | Purified from E. coli Arctic Express cells | |
Peptide, recombinant protein | GFP-doublecortin (1–365) | This paper | Purified from E. coli BL21 cells | |
Peptide, recombinant protein | GFP-doublecortin (1-302) | This paper | Purified from E. coli BL21 cells | |
Peptide, recombinant protein | mCherry-doublecortin (1-365) | This paper | Purified from E. coli BL21 cells | |
Peptide, recombinant protein | α/β-Tubulin | Cytoskeleton | TL590M-A | |
Peptide, recombinant protein | Rhodamine-labeled α/β-tubulin | Cytoskeleton | T240 | |
Peptide, recombinant protein | Streptavidin–HRP | Thermo Fisher Scientific | N100 | |
Peptide, recombinant protein | Glucose oxidase | Sigma-Aldrich | G2133-10KU | |
Peptide, recombinant protein | Catalase | Sigma-Aldrich | E3289 | |
Chemical compound, drug | Paclitaxel | European Pharmacopoeia Reference Standard | Y0000698 | |
Chemical compound, drug | Docetaxel | Sigma-Aldrich | 01885 | |
Chemical compound, drug | Atto 633 NHS-ester | ATTO-TEC GmbH | AD 633-35 | |
Chemical compound, drug | LC-SDA | Thermo Fisher Scientific | 26,168 | |
Chemical compound, drug | DSS | Thermo Fisher Scientific | 21,655 | |
Chemical compound, drug | EDC | Thermo Fisher Scientific | 22,980 | |
Software, algorithm | xVis | https://xvis.genzentrum.lmu.de/login.php | PMID:25956653 | |
Software, algorithm | xiNET | http://crosslinkviewer.org/ | PMID:25648531 | |
Software, algorithm | IMP | https://integrativemodeling.org/ | v.2.12 | PMID:22272186 |
Software, algorithm | Mass Spec Studio | https://www.msstudio.ca | V2.0 | PMID:25242457 |
Software, algorithm | TrackMate | https://imagej.net/plugins/trackmate/ | PMID:27713081 |
Additional files
-
Supplementary file 1
Complete list of crosslinking sites (and their associated crosslinked peptides) identified for MT-DCX construct using LC-SDA photo-chemical crosslinking.
- https://cdn.elifesciences.org/articles/66975/elife-66975-supp1-v2.xlsx
-
Supplementary file 2
The analysis output of all integrative structural modeling runs.
Column titles with star are defined at the bottom of the table.
- https://cdn.elifesciences.org/articles/66975/elife-66975-supp2-v2.xlsx
-
Supplementary file 3
Complete list of unique DCX-DCX crosslinking sites (and their associated crosslinked peptides) identified using isotope-assisted chemical crosslinking.
- https://cdn.elifesciences.org/articles/66975/elife-66975-supp3-v2.xlsx
-
Transparent reporting form
- https://cdn.elifesciences.org/articles/66975/elife-66975-transrepform1-v2.pdf