TGFβ signalling is required to maintain pluripotency of human naïve pluripotent stem cells
Figures
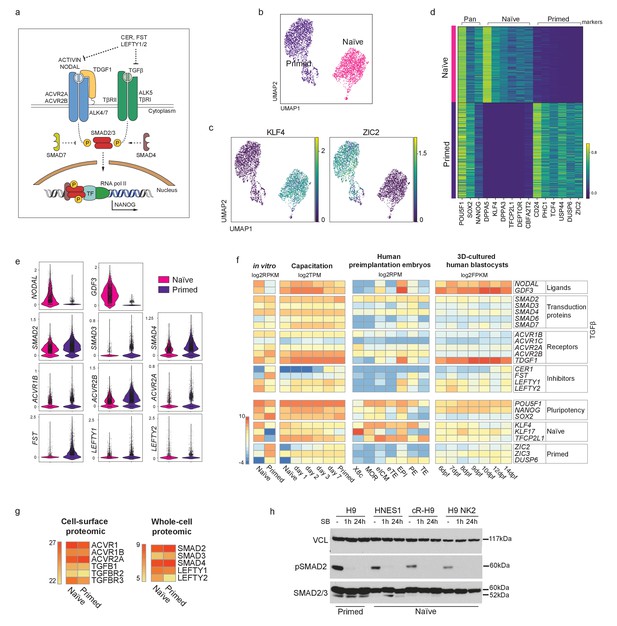
TGFβ signalling pathway is active in human naïve pluripotent stem cells.
(a) Overview of the TGFβ signalling pathway. Extracellular ligands ACTIVIN and NODAL bind to type I (ACVR2A/2B) and type II transmembrane receptors (ALK4/7), and TGFβ binds to TβRI and TβRII/ALK5. NODAL requires the additional transmembrane co-receptor TDGF1 (CRIPTO1). The activated receptor complex phosphorylates the linker region of SMAD2 and SMAD3, which enter the nucleus in complex with SMAD4. They act as transcriptional regulators and induce or repress the transcription of their target loci by recruiting other transcription factors (TF) and epigenetic modifiers. Several negative regulators of the signalling pathway are also shown: LEFTY1/2 block the signalling pathway by binding to the receptors; Cerberus (CER) and Follistatin (FST) block the ligands; SMAD7 inhibits the SMAD2/3 complex. (b) 10X RNA-seq data of naïve and primed hPSCs represented on a UMAP plot. (c) UMAP visualisation of naïve and primed hPSCs reporting the relative expression of respective pluripotent state markers, KLF4 and ZIC2. (d) Heatmap reporting the expression values of selected naïve and primed marker genes divided in pan-pluripotency markers, and naïve- and primed-specific markers within the top 250 differentially expressed genes. (e) Violin plots of the 10X RNA-seq data comparing the transcript expression of TGFβ effectors in naïve and primed hPSCs. (f) Heatmap summarising the transcript expression of TGFβ effectors and pluripotency genes. RNA-seq datasets shown are: in vitro-cultured naïve and primed hPSCs (Collier et al., 2017), hPSCs undergoing naïve to primed state capacitation (Rostovskaya et al., 2019), human pre-implantation embryos (Petropoulos et al., 2016), and epiblast cells within a 3D human blastocyst culture system (Xiang et al., 2020). X8c: 8-cell stage; MOR: morula; eICM: early-ICM; eTE: early-trophectoderm; EPI: epiblast; PE: primitive endoderm; TE: trophectoderm. Dpf: days post-fertilisation. (g) Heatmaps summarising protein abundance levels determined by cell-surface proteomics (Wojdyla et al., 2020) and whole cell proteomics (Di Stefano et al., 2018) for TGFβ effectors in naïve and primed hPSCs. (h) Western blot analysis of TGFβ signalling pathway activation in H9 primed hPSCs (cultured in E8 medium) and in three naïve hPSC lines cultured in t2iLGö medium: embryo-derived HNES1, chemically reset cR-H9, and transgene-reset H9 NK2. Blots show SMAD2 phosphorylation signal (pSMAD2-Ser465/Ser467) and total SMAD2/3 levels in normal conditions (-), and following 1 hr and 24 hr of SB-431542 supplementation to their culture media. Vinculin (VCL) used as a loading control.
-
Figure 1—source data 1
Full uncropped western blot from Figure 1h and Figure 1—figure supplement 1e reporting TGFβ pathway activation in primed H9 cells (cultured in E8 medium) and in three naïve hPSC lines cultured in t2iLGö medium: embryo-derived HNES1, chemically reset cR-H9, and transgene-reset H9 NK2 naïve cells.
Blots show SMAD2 phosphorylation signal and total SMAD2/3 in normal conditions (-), and following 1 hr and 24 hr of SB-431542 supplementation to their culture media. Two separate exposures are shown, Vinculin (VCL) used as loading control. (a) Original files of the full raw unedited blots. (b) Figures with the uncropped blots with the bands reported in the main figures highlighted in red and labelled accordingly.
- https://cdn.elifesciences.org/articles/67259/elife-67259-fig1-data1-v1.pdf
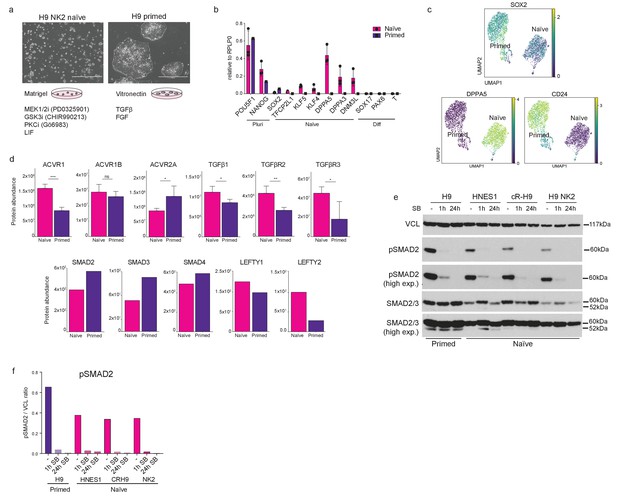
Validation of naïve and primed hPSCs and TGFβ signalling pathway activation.
(a) Overview of the conditions used for culturing naïve and primed hPSCs and representative phase-contrast images. (b) RT-qPCR expression analysis of pan-pluripotency genes (Pluri), naïve markers (naïve) and early-stage germ cell layer markers (Diff) in naïve and primed hPSCs. Data show the mean ± SD of three biological replicates, relative to the housekeeping gene RPLP0. (c) UMAP visualisation of naïve and primed cells reporting the relative expression of pluripotency marker SOX2, naïve marker DPPA5, and primed marker CD24. (d) Protein abundance levels for several TGFβ receptors (upper; Wojdyla et al., 2020) and transduction proteins (lower; Di Stefano et al., 2018) in naïve and primed hPSCs. The upper charts show the mean ± SD of three (naïve) or four (primed) biological replicates and were compared using a LIMMA-moderated t test with Benjamini-Hochberg correction (ns, q > 0.05, *q < 0.05, **q < 0.01, ***q < 0.001, ****q < 0.0001). The lower charts show protein abundance of one biological replicate per cell type. (e) Expanded western blot from Figure 1h comparing TGFβ pathway activation in primed H9 cells (cultured in E8 medium) and in three naïve hPSC lines cultured in t2iLGö medium: embryo-derived HNES1, chemically reset cR-H9, and transgene-reset H9 NK2 naïve cells. Blots show SMAD2 phosphorylation signal and total SMAD2/3 in normal conditions (-), and following 1 hr and 24 hr of SB-431542 supplementation to their culture media. Two separate exposures are shown. Vinculin (VCL) used as loading control. (f) Relative quantification of western blot from Figure 1h performed using the software Fiji (ImageJ) reporting the pSMAD2 / Vinculin (VCL) ratio.
-
Figure 1—figure supplement 1—source data 1
Numerical data that are represented in Figure 1—figure supplement 1.
- https://cdn.elifesciences.org/articles/67259/elife-67259-fig1-figsupp1-data1-v1.xlsx
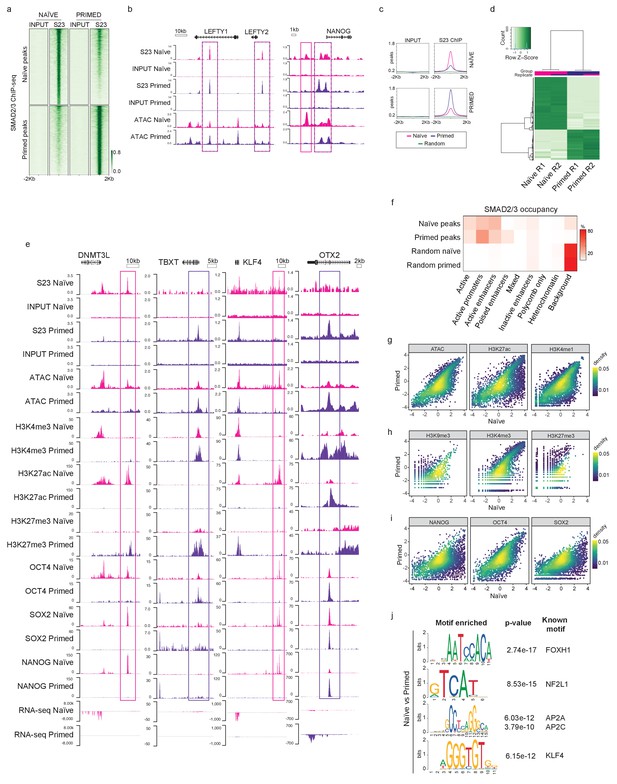
SMAD2/3 binds to chromatin at common and pluripotent state-specific sites.
(a) Heatmap displaying normalised SMAD2/3 (S23) ChIP-seq reads ±2 kb from the centre of SMAD2/3-bound peaks that were independently defined in naïve (H9 NK2 line cultured in t2iLGö medium) and primed (H9 line cultured in E8 medium) hPSCs; two biological replicates per cell line. Top panel shows the regions identified as SMAD2/3-bound peaks in naïve cells; lower panel shows SMAD2/3-bound peaks in primed cells. (b) Genome browser tracks reporting SMAD2/3 (S23) binding (this study) and chromatin accessibility (ATAC-seq; Pastor et al., 2018) at the LEFTY1/2 and NANOG loci in naïve and primed hPSCs. Input tracks are shown as controls. (c) Normalised average meta-plots of SMAD2/3 (S23) ChIP signal ±2 kb from the centre of the peaks in naïve and primed hPSCs, compared to a randomly-selected subset of regions. (d) Heatmap displaying regions that are differentially bound by SMAD2/3 in naïve and primed hPSCs in two biological replicates (R1 and R2). (e) Genome browser tracks reporting expression (RNA-seq), chromatin accessibility (ATAC-seq), and ChIP-seq datasets of SMAD2/3 (S23), histone marks for enhancers (H3K27ac) and promoters (H3K4me3, H3K27me3), and transcription factors (OCT4, SOX2, NANOG) at the DNMT3L, TBXT, KLF4, OTX2 loci. Input tracks are shown as controls. The following data sets are shown: ATAC-seq (Pastor et al., 2018); H3K4me3 (Theunissen et al., 2014); H3K4me1 (Chovanec et al., 2021; Gifford et al., 2013); H3K27me3 (Theunissen et al., 2014); H3K27ac (Ji et al., 2016); OCT4 (Ji et al., 2016); SOX2 (Chovanec et al., 2021); NANOG (Chovanec et al., 2021; Gifford et al., 2013), and RNA-seq (Takashima et al., 2014). (f) Heatmap showing the frequency of SMAD2/3 peak centre locations with respect to ChromHMM states in naïve and primed hPSCs (Chovanec et al., 2021). SMAD2/3 peaks in naïve and primed hPSCs were annotated with their respective ChromHMM states. The annotations associated with the randomly-selected control regions reflect the overall genomic representation of chromatin states. (g-i) Density coloured scatter plots showing indicated ChIP-seq and ATAC-seq values (log2 RPM) in naïve versus primed hPSCs. Each dot corresponds to one naïve-specific SMAD2/3 peak. (j) Differential motif enrichment reporting the top four motifs (ranked by p-value) at SMAD2/3-binding sites in naïve hPSCs that are enriched compared to motifs identified at SMAD2/3-binding sites in primed hPSCs.
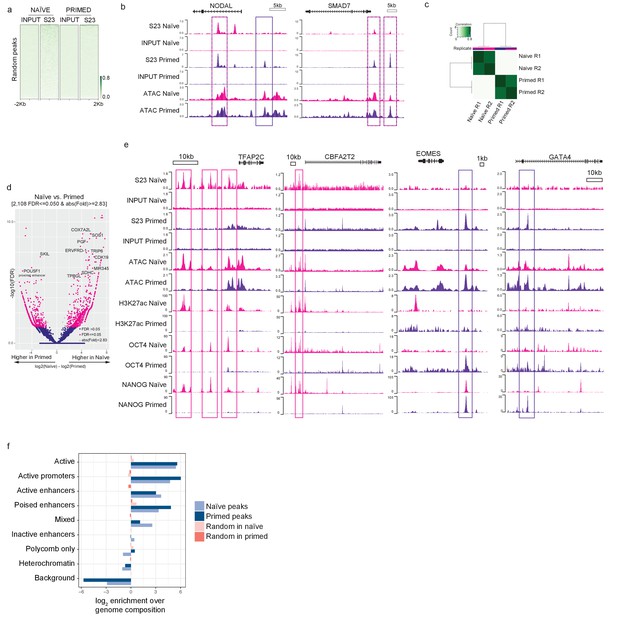
SMAD2/3 binds to chromatin at common and state-specific sites.
(a) Heatmap displaying normalised SMAD2/3 ChIP-seq signal ±2 kb from the centre of the peaks in a randomly selected subset of regions, complementing Figure 2a. (b) Genome browser tracks overlapping SMAD2/3 (S23) binding sites in naïve and primed cells with chromatin accessibility (ATAC-seq) at the NODAL and SMAD7 loci. Input is shown as control. (c) Correlation heatmap reporting the clustering of SMAD2/3 ChIP-seq replicates (R1 and R2) for each cell type, based on the count scores. (d) Volcano plot reporting the fold change in SMAD2/3 ChIP-seq signal at SMAD2/3 peaks between naïve and primed cells and the associated false discovery rate. Each dot represents a SMAD2/3 peak. Dots highlighted in pink are significantly differentially bound sites (log10 FDR ≤ 0.05; log2 fold changes ≥ 1.5). (e) Genome browser tracks exemplify the overlap between SMAD2/3 (S23) ChIP-seq binding sites in naïve and primed cells with chromatin accessibility (ATAC-seq), histone marks for active enhancers (H3K27ac), and OCT4 and NANOG ChIP-seq signal. Input is shown as control. (f) Bar plot reporting the log2 enrichment of SMAD2/3 peaks at ChromHMM-defined promoter and enhancer regions compared to other genomic regions. Related to Figure 2f.
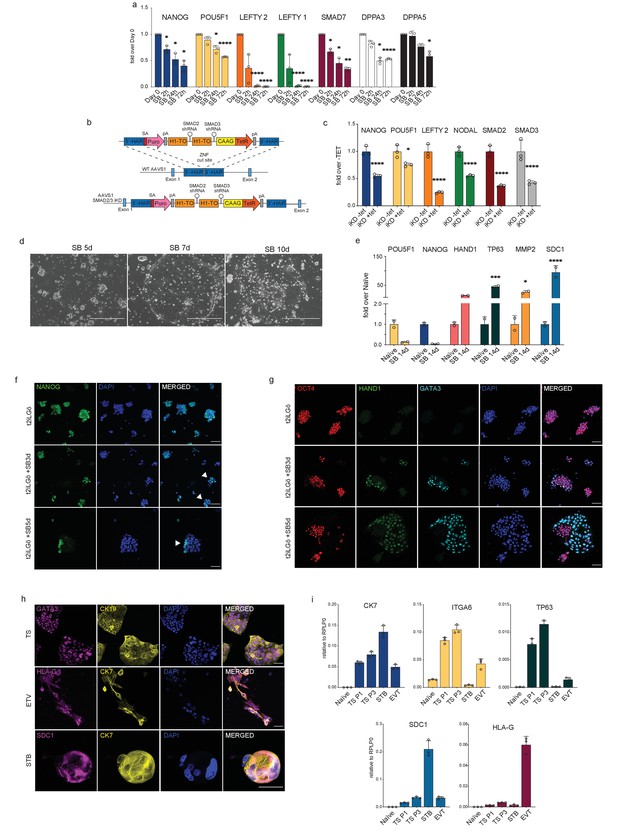
Inhibiting TGFβ signalling induces loss of pluripotency in naïve hPSCs.
(a) RT-qPCR expression analysis of pluripotency-associated genes and TGFβ-associated genes in naïve hPSCs (H9 NK2 line) following SB-431542 treatment (t2iLGö + SB). Expression levels are shown as fold changes relative to day 0. (b) Schematic showing the integration of a single-step optimised inducible knock-down targeting construct into the AAVS1 locus of H9 hPSCs, enabling the expression of SMAD2 and SMAD3 short hairpin RNAs (shRNAs) under the control of a tetracycline inducible promoter. ZFN: zinc-finger nucleases; 5’-HAR/3’-HAR: upstream/downstream homology arm; H1-TO: Tetracycline-inducible H1 Pol III promoter carrying one tet operon after the TATA box; CAAG: CMV early enhancer, chicken β-actin and rabbit β-globin hybrid promoter; TetR: Tetracycline-sensitive repressor protein; SA: splice acceptor; Puro, Puromycin resistance; pA, polyadenylation signal. Schematic adapted from Bertero et al., 2016. (c) RT-qPCR analysis of gene expression levels in SMAD2/3 inducible knock-down (iKD) H9 naïve hPSCs following 5 days of tetracycline (tet) treatment. Expression levels are shown for each gene as fold change relative to iKD -tet. Cells were cultured in t2iLGö medium. (d) Phase contrast pictures of H9 NK2 naïve hPSCs after 5, 7, and 10 days of SB treatment in t2iLGö medium. Scale bars: 400 µm. (e) RT-qPCR analysis of trophoblast (HAND1, TP63, MMP2, and SDC1) and pluripotency (POU5F1, NANOG) gene expression levels in naïve hPSCs following long-term (14 days) SB treatment in t2iLGö medium. Expression levels are shown as fold changes relative to day 0 samples, n = two biological replicates. (f) Immunofluorescence microscopy showing the downregulation of NANOG (green) in naïve hPSCs following 3 and 5 days of SB treatment. DAPI signal in blue. White arrowheads indicate colonies displaying heterogeneous expression of NANOG. Scale bars: 50 µm. (g) Immunofluorescence microscopy for OCT4 (red), HAND1 (green), GATA3 (cyan), and DAPI (blue) in naïve hPSCs following 3 and 5 days of SB treatment in t2iLGö medium. Scale bars: 50 µm. (h) Immunofluorescence microscopy for GATA3, HLA-G, SDC1 (magenta), CK19 and CK7 (yellow), and DAPI (blue) in naïve-derived trophoblast stem cells (TS), extravillous trophoblast (EVT), and syncytiotrophoblast (STB). Scale bars: 50 µm. (i) RT-qPCR analysis of gene expression levels in naïve-derived trophoblast stem cells (TS), extravillous trophoblast (EVT) and syncytiotrophoblast (STB) compared to undifferentiated naïve hPSCs. Expression levels are shown for each gene relative to the housekeeping gene RPLP0. RT-qPCR data show the mean ± SD of three biological replicates (unless specified otherwise) and were compared to their relative control using an ANOVA with Tukey's or Šídák's multiple comparisons test (*p ≤ 0.05, **p≤ 0.01, ***p≤ 0.001, ****p≤ 0.0001).
-
Figure 3—source data 1
Numerical data that are represented in Figure 3.
- https://cdn.elifesciences.org/articles/67259/elife-67259-fig3-data1-v1.xlsx
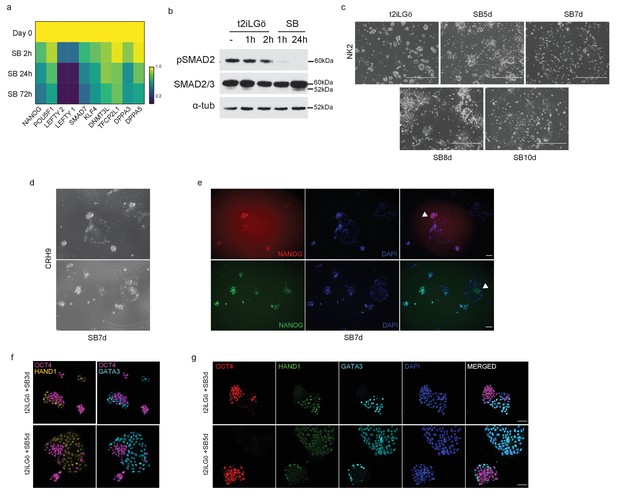
TGFβ signalling inhibition induces loss of pluripotency in different naïve hPSCs.
(a) Heatmap reporting RT-qPCR expression analysis of pluripotency genes and TGFβ-associated genes in naïve cells following the addition of SB to the culture medium (t2iLGö), related to Figure 3a. Data show the mean of three biological replicates as fold changes compared to day 0. (b) Western blot showing TGFβ pathway activation in H9 NK2 naïve cells through the phosphorylation of SMAD2 (pSMAD2) and also total SMAD2/3 in normal conditions (-), after 1 hr and 2 hr of fresh media change (t2iLGö), and following 1 hr and 24 hr of SB treatment (t2iLGö+SB). Alpha-tubulin (α-tub) used as loading control. (c) Phase-contrast images of H9 NK2 naïve cells at day 0 (t2iLGö) and following 5, 7, 8, and 10 days of SB supplementation to the t2iLGö medium. Scale bars: 400 µm. (d) Phase-contrast images of chemically-reset cR-H9 naïve cells after 7 days of SB treatment. (e) Immunofluorescence microscopy of H9 NK2 naïve hPSCs for NANOG (red/green), and DAPI (blue), after 7 days of SB treatment in chemically-reset cR-H9 naïve cells, matching the phase-contrast images in (d). White arrowheads indicate colonies displaying heterogeneous NANOG expression. Scale bars: 50 µm. (f) Immunofluorescence microscopy complementing Figure 3g showing (left) OCT4 (magenta) and HAND1 (yellow) overlap, or (right) OCT4 (magenta) and GATA3 (cyan) overlap after 3 and 5 days of SB treatment. Scale bars: 50 µm. (g) Immunofluorescence microscopy of H9 NK2 naïve hPSCs for OCT4 (red), HAND1 (green), GATA3 (cyan) and DAPI (blue) after 3 (upper) and 5 (lower) days of SB treatment. Scale bars: 50 µm.
-
Figure 3—figure supplement 1—source data 1
Full uncropped western blot from Figure 3—figure supplement 1b reporting TGFβ pathway activation in H9 NK2 naïve cells through the phosphorylation of SMAD2 (pSMAD2) and also total SMAD2/3 in normal conditions (-), after 1 hr and 2 hr of fresh media change (t2iLGö), and following 1 hr and 24 hr of SB treatment (t2iLGö+SB).
Alpha-tubulin (α-tub) used as loading control. Additional lanes (not shown in the main figure for clarity) report as positive control naïve (N) and primed (P) hPSC treated for 1 hr with Activin. (a) original files of the full raw unedited blots. (b) figures with the uncropped blots with the bands reported in the main figures highlighted in red and labelled accordingly.
- https://cdn.elifesciences.org/articles/67259/elife-67259-fig3-figsupp1-data1-v1.pdf
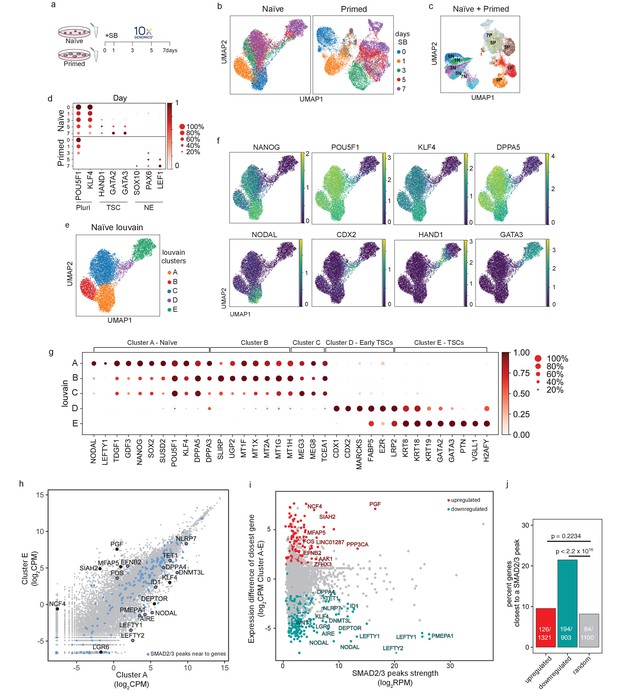
Single-cell transcriptional analysis reveals a trophoblast-like population arising in response to TGFβ inhibition in naïve hPSCs.
(a) Overview of the experimental procedure. Naïve and primed hPSCs were cultured in the presence of SB-431542 (SB), a potent TGFβ inhibitor, and samples were collected at days 0, 1, 3, 5, and 7. Single-cell transcriptomes were obtained by 10X sequencing. (b) UMAP visualisation of naïve and primed cells during the SB time-course experiment, separated by days of treatment. (c) UMAP visualisation of the combined naïve and primed data set, separated by days of SB treatment (indicated by the number in the labels). N, naïve; P, primed. (d) Dot plot of selected gene expression values in naïve and primed cells during the SB time-course experiment, plotted by days of treatment (in rows). Each dot represents two values: mean expression within each category (visualised by colour) and fraction of cells expressing the gene (visualised by the size of the dot). Genes are indicative of pluripotent cells (Pluri), trophoblast stem cells (TSC), and neuroectoderm cells (NE). (e) UMAP visualisation of naïve hPSCs during the SB time-course experiment, separated by Louvain clustering (five clusters, A to E). (f) UMAP visualisation of naïve cells during the SB time-course experiment, showing the relative expression of pluripotency markers, NANOG, POU5F1, KLF4, and DPPA5; TGFβ effectors, NODAL; and trophoblast markers, CDX2, HAND1, GATA3. (g) Dot plot of expression values in naïve cells during the SB time-course experiment, separated by the five Louvain clusters. The genes shown represent a subset of the top 25 differentially expressed genes between the five clusters, as reported in Figure 5—figure supplement 1e. Each dot represents two values: mean expression within each category (visualised by colour) and fraction of cells expressing the gene (visualised by the size of the dot). (h) Scatter plot reporting pseudobulk RNA-seq values (from 10X data) for cells in Louvain clusters A and E. Each dot represents one gene. Genes that have SMAD2/3 ChIP-seq peaks (log2 RPM > 5) within 12 kb of their transcription start site (TSS) are highlighted in blue and annotated. Several differentially expressed genes that are the closest gene to a SMAD2/3 peak (but are further away than 12 kb) are also named. (i) Scatter plot showing SMAD2/3 ChIP-seq peak strength (log2 RPM) versus the expression difference (cluster A – cluster E; log2 CPM) of the gene nearest to the SMAD2/3 peak. Upregulated genes, red; downregulated genes, green. (j) SMAD2/3 peaks were annotated with their nearest genes. Bar plot showing the percentage of genes that are the closest gene to a SMAD2/3 peak for genes that are upregulated (red) or downregulated (green) between cells in clusters A and E. A randomly selected set of control genes are shown in grey. The number of closest genes and the set size are reported within the bars. Statistical testing was performed using Chi-square test with Yates continuity and Bonferroni multiple testing correction.
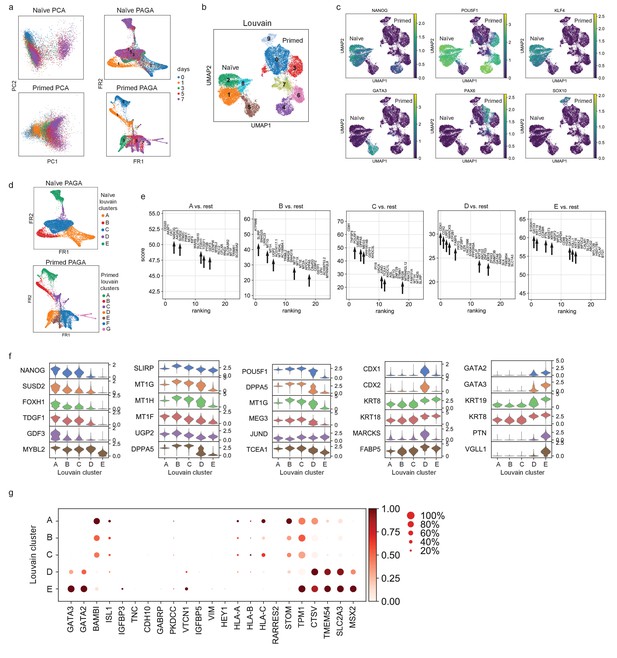
Single-cell transcriptional analysis reveals different trajectories between naïve and primed hPSCs following TGFβ inhibition.
(a) PCA and partition-based graph abstraction (PAGA) connectivity visualisation of the 10X RNA-seq data in naïve and primed hPSCs during the SB time-course, separated by days of treatment. (b) UMAP visualisation of the combined naïve and primed cell 10X dataset during the SB time-course experiment, separated by Louvain clustering. (c) UMAP visualisation of the combined naïve and primed cell 10X dataset reporting the relative expression of pluripotency markers NANOG, POU5F1, KLF4, trophoblast marker GATA3, and neuroectoderm markers PAX6, SOX10. (d) PAGA connectivity visualisation of naïve and primed cells during the SB time-course experiment, separated by Louvain clustering. (e) Summarised results showing the top 25 differentially expressed genes between Louvain clusters, identified by applying a Wilcoxon-Rank-Sum test. Black arrows highlight informative genes relative to each cluster. (f) Violin plots reporting the expression of a subset of genes identified in Figure 4g and (e). (g) Dot plot of expression values in naïve cells during the SB time-course experiment, separated by the five Louvain clusters. The genes shown represent a subset of amnion marker genes reported in Guo et al., 2021; Io et al., 2021; Zhao et al., 2021. Note that some genes are also expressed in trophoblast cells. Each dot represents two values: mean expression within each category (visualised by colour) and fraction of cells expressing the gene (visualised by the size of the dot).
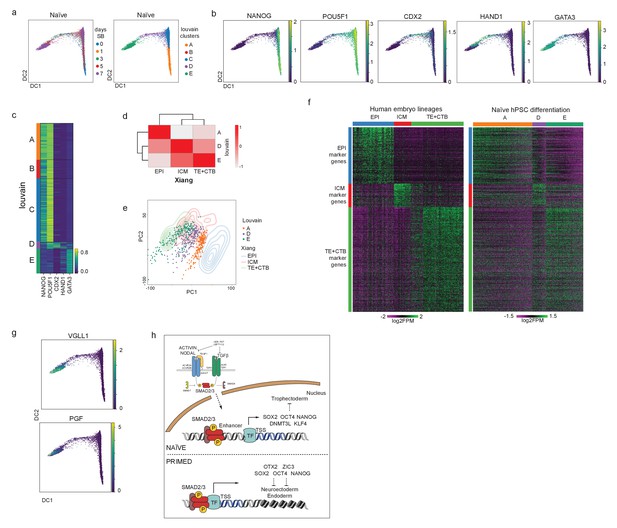
Differentiation of TGFβ-inhibited naïve hPSCs transcriptionally recapitulates early trophectoderm specification in human embryos.
(a) Diffusion maps of naïve cells during the SB time-course experiment, separated by days of treatment (left) and Louvain clustering (right). (b) Overlay of the diffusion maps with the relative expression of pluripotency markers NANOG, and POU5F1, and trophoblast markers CDX2, HAND1, GATA3. (c) Heatmap of the expression values of genes reported in (b) separated by the Louvain clusters. Note the overlap in the expression of pluripotency and trophoblast markers in cells within cluster D. (d) Correlation plot between pseudobulk data from Louvain clusters A/D/E and EPI (Epiblast), ICM (Inner Cell Mass), and TE+CTB (Trophectoderm+Cytotrophoblast) from cultured human pre-gastrulation embryos (Xiang et al., 2020). (e) PCA plot overlapping 200 randomly selected cells from each of the Louvain clusters A/D/E (individual dots) and data from 3D-cultured human pre-gastrulation embryos (Xiang et al., 2020), based on EPI, ICM, and TE+CTB cells (contour lines). PC1 variance 2.15, PC2 variance 1.41. (f) Heatmaps visualising the expression of genes in EPI, ICM, and TE+CTB (Xiang et al., 2020) and cells in Louvain clusters A/D/E. Note that the genes are in the same order for both plots. (g) Diffusion maps of naïve cells during the SB time-course experiment showing the relative expression of CTB markers – VGLL1 and PGF. (h) We propose there is a continuum of TGFβ/Activin/Nodal signalling that spans a developmental window of human pluripotent states from naïve to primed. In both states, active TGFβ signalling promotes the expression of common pluripotency genes, such as NANOG and POU5F1, and contributes to the maintenance of pluripotency. SMAD2/3 are additionally required in naïve hPSCs to sustain the expression of naïve pluripotency factors, including KLF4 and DNMT3L. Inactivating TGFβ signalling in naïve hPSCs leads to the downregulation of pluripotency genes, thereby enabling the induction of trophoblast differentiation.
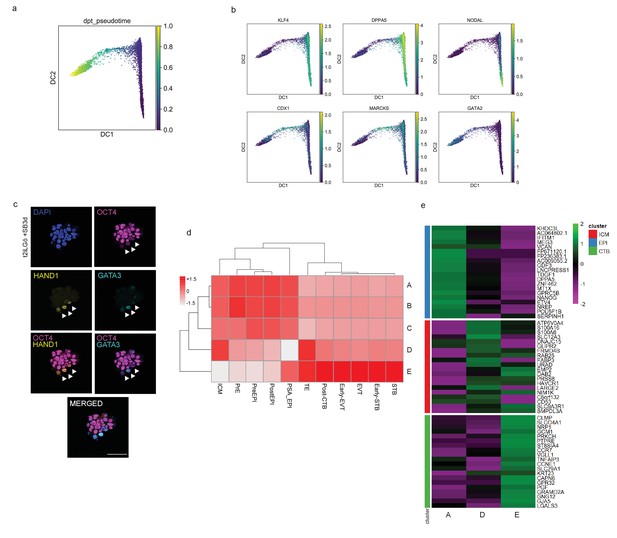
Pseudotime trajectories of TGFβ-inhibited naïve hPSCs recapitulates early trophectoderm specification in human embryos.
(a) Diffusion maps of naïve hPSC 10X scRNA-seq data during the SB time-course, reporting diffusion pseudotime scores. (b) Diffusion map visualisation of naïve cells during the SB time-course reporting the relative expression of additional pluripotency markers KLF4 and DPPA5, TGFβ effector NODAL, and trophoblast markers CDX1, MARCKS, and GATA2. (c) Immunofluorescence microscopy for OCT4 (magenta), HAND1 (yellow), GATA3 (cyan) and DAPI (blue) in H9 NK2 naïve cells following 3 days of SB supplementation to t2iLGö medium. White arrowheads indicate cells that co-express low levels of OCT4 and HAND1 or GATA3. Scale bars: 50 µm. (d) Correlation heatmap between the pseudobulked datasets from all Louvain clusters (A/B/C/D/E) and the identified cell lineages from the cultured human pre-gastrulation embryos (Xiang et al., 2020). CTB: Cytotrophoblast; EVT: Extravillous trophoblast; STB: Syncytiotrophoblast; EPI: Epiblast; ICM: Inner Cell Mass; PrE: Primitive Endoderm, TE: Trophectoderm. (e) Heatmap reporting the expression in the Louvain clusters A, D, and E of the top 20 differentially expressed genes between EPI, ICM, and CTB cell lineages (based on data published by Xiang et al., 2020).

UMAP visualisation of gene expression in naïve cells following TGFβ inhibition, as measured by 10X scRNA-seq.
a) Overview of the louvain clusters described in the manuscript. The plots show that reported b) amnion markers such as ISL1 and CDH10 (Guo et al., 2021) and c) BAP markers such as HLA-A/B/C (Io et al., 2021) are largely undetectable in this time course data set. d) TMP1 and CTSV are reported to be expressed in both trophoblast and amnion cells, and e) CCKBR is reported to be more abundant in trophoblast compared to amnion cells (Guo et al., 2021).

UMAP visualisation of gene expression in naïve cells following TGFβ inhibition, as measured by 10X scRNA-seq.
The plots show that trophoblast markers such as GATA3 are uniformly expressed in ‘Cluster E’, but markers of syncytiotrophoblast (STB) such as SDC1 and extravillous trophoblast (EVT) such as MMP2 are expressed in only a small number of cells in this population (indicated by the arrows).
Tables
Reagent type (species) or resource | Designation | Source or reference | Identifiers | Additional information |
---|---|---|---|---|
Gene (Homo sapiens) | SMAD2 | GenBank | Gene ID:4087 | |
Gene (Homo sapiens) | SMAD3 | GenBank | Gene ID:4088 | |
Gene (Homo sapiens) | NODAL | GenBank | Gene ID:4838 | |
Gene (Homo sapiens) | POU5F1 | GenBank | Gene ID:5460 | |
Gene (Homo sapiens) | NANOG | GenBank | Gene ID:79923 | |
Gene (Homo sapiens) | KLF4 | GenBank | Gene ID:9314 | |
Gene (Homo sapiens) | GATA3 | GenBank | Gene ID:2625 | |
Gene (Homo sapiens) | CDX2 | GenBank | Gene ID:1045 | |
Cell line (Homo sapiens) | WA09/H9 | WiCell | RRID:CVCL_9773 | Human Embryonic Stem Cell line |
Cell line (Homo sapiens) | HNES1 | DOI:10.1016/j.stemcr.2016.02.005 | RRID:CVCL_9R98 | Human Embryonic Stem Cell line |
Transfected construct (Homo sapiens) | SMAD2 shRNA | Sigma | TRCN0000010477 | CCGGCAAGTACTCCTTGCTGGATTGCTCGAGCAATCCAGCAAGGAGTACTTGTTTTTG |
Transfected construct (Homo sapiens) | SMAD3 shRNA | Sigma | TRCN0000330055 | CCGGGCCTCAGTGACAGCGCTATTTCTCGAGAAATAGCGCTGT CACTGAGGCTTTTTG |
Antibody | SMAD2/3 (Goat polyclonal) | R&D | AF3797; RRID:AB_2270778 | ChIP 10 µg |
Antibody | SMAD2/3 (Rabbit monoclonal Biotinylated) | Cell signaling | 12470S; RRID:AB_2797930 | WB 1:5,000 |
Antibody | pSMAD2 (Rabbit monoclonal) | Cell signaling | 3108S; RRID:AB_490941 | WB 1:1,000 |
Antibody | α-Tubulin (Mouse monoclonal) | Sigma | T6199; RRID:AB_477583 | WB 1:10,000 |
Antibody | Vinculin (Mouse monoclonal) | Sigma | SAB4200080; RRID:AB_10604160 | WB 1:20,000 |
Antibody | GATA3 (D13C9) (Rabbit monoclonal) | Cell signaling | 5852S; RRID:AB_10835690 | IF 1:100 |
Antibody | NANOG (Rabbit polyclonal) | Abcam | ab21624; RRID:AB_446437 | IF 1:100 |
Antibody | OCT3/4 (C-10) (Mouse monoclonal) | Santa Cruz Biotechnology | sc-5279; RRID:AB_628051 | IF 1:200 |
Antibody | HAND1 (Goat polyclonal) | R&D | AF3168; RRID:AB_2115853 | IF 1:200 |
Antibody | CK7 (Rabbit monoclonal) | Abcam | ab68459; RRID:AB_1139824 | IF 1:100 |
Antibody | CK19 (Mouse monoclonal) | Abcam | ab7754; RRID:AB_306048 | IF 1:100 |
Antibody | SDC1 (Mouse monoclonal) | Abcam | ab34164; RRID:AB_778207 | IF 1:100 |
Antibody | HLA-G (Mouse monoclonal) | Santa Cruz Biotechnology | sc-21799; RRID:AB_627938 | IF 1:100 |
Antibody | Secondary antibodies | Supplementary file 1 | ||
Sequence-based reagent | RT-qPCR primers | Supplementary file 2 | ||
Chemical compound, drug | SB-431542 | Tocris | 1614 | 10–20 µM |
Software, algorithm | Fiji | https://doi.org/10.1038/nmeth.2019 | RRID:SCR_002285 | |
Software, algorithm | GraphPad Prism | http://www.graphpad.com/ | RRID:SCR_002798 | |
Software, algorithm | SeqMonk | http://www.bioinformatics.babraham.ac.uk/projects/seqmonk/ | RRID:SCR_001913 | |
Software, algorithm | RStudio | http://www.rstudio.com/ | RRID:SCR_000432 | R packages used specified in Materials and Methods |
Software, algorithm | CellRanger | https://support.10xgenomics.com/single-cell-gene-expression/software/pipelines/latest/what-is-cell-ranger | RRID:SCR_017344 | |
Software, algorithm | Scanpy | https://github.com/theislab/scanpy | RRID:SCR_018139 | |
Other | DAPI stain | Invitrogen | D1306 | 0.1 µg/ml |
Additional files
-
Supplementary file 1
Table of antibodies used for Western Blots, Immunofluorescence and Chromatin Immunoprecipitation and relative dilutions.
- https://cdn.elifesciences.org/articles/67259/elife-67259-supp1-v1.xlsx
-
Supplementary file 2
Table of primer sequences for RT-qPCR.
- https://cdn.elifesciences.org/articles/67259/elife-67259-supp2-v1.xlsx
-
Transparent reporting form
- https://cdn.elifesciences.org/articles/67259/elife-67259-transrepform-v1.docx