GABAB receptor auxiliary subunits modulate Cav2.3-mediated release from medial habenula terminals
Figures
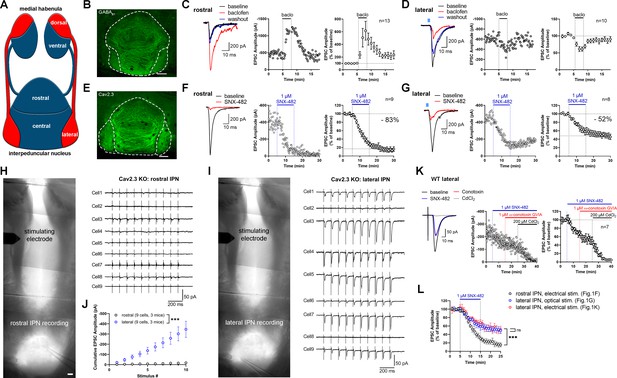
Expression and function of GABAB receptors and Cav2.3 at two parallel MHb-IPN pathways.
(A) Schematic drawing of the two MHb-IPN pathways. In red: the dorsal part of the MHb projects to the lateral subnuclei of the IPN. In blue: the ventral part of the MHb projects to the rostral/central subnuclei of the IPN. (B) Confocal image of GABAB1 immunofluorescence signal indicates the presence of GABAB receptors (GBRs) in all IPN subnuclei. (C) In whole-cell recordings of rostral IPN neurons, activation of GBRs by baclofen (1 µM) produced a potentiation of electrically evoked EPSC amplitudes. Left: example EPSC traces before (black) and during the application of baclofen (red) and after washout of baclofen (blue); middle: example time course of EPSC amplitudes in one cell; right: averaged time course of relative EPSC amplitude change after baclofen (n = 13 cells/9 mice). (D) Baclofen reduced the amplitude of light-evoked glutamatergic EPSCs in lateral IPN neurons (n = 10 cells/5 mice). (E) Confocal image of Cav2.3 immunofluorescence signal indicates Cav2.3 presence in MHb axonal projections of both MHb-IPN pathways. (F) Pharmacological inhibition of Cav2.3 with SNX-482 in whole-cell recordings of rostral IPN neurons. Left: example traces before and after the application of SNX-482; middle: example time course of EPSC amplitude reduction by SNX-482; right: averaged time course of relative EPSC amplitude reduction by SNX-482. EPSC amplitudes were reduced by 83% on average (n = 9 cells/9 mice). (G) In Tac1-ChR2-EYFP mice, SNX-482 reduced light-evoked glutamatergic EPSC amplitudes on average by 52% (n = 8 cells/4 mice). (H, I) Left: Positions of recording and stimulating electrodes in acute thick-slice preparations from Cav2.3 KO mice. The stimulating electrode was placed on the fasciculus retroflexus just below the MHb, 2–3 mm from the recording sites. The position of the stimulating electrode and the stimulation intensity remained unchanged between rostral and lateral IPN recordings. Right: 10 Hz EPSC traces of all recorded neurons (9 cells/3 mice). (J) Cumulative EPSC amplitude plot shows significantly higher EPSC amplitudes in lateral compared to rostral IPN neurons. ***p<0.0001 two-way ANOVA; (K) FR stimulation and lateral IPN recording in 1 mm thick slice of wild-type mice. Sequential application of SNX-482, ω-conotoxin GVIA, and CdCl2. (L) Time course overlay of (F), (G), and (K) to compare SNX-482 time courses between minutes 0 and 25. For simplicity, application of ω-conotoxin GVIA starting from minute 15 in (K) not indicated in the graph. ***p<0.0001 two-way ANOVA with Tukey’s post hoc test. Scale bars in(B), (E), (H), and (I) are 100 µm. Averaged data is presented as mean ± SEM. See also Figure 1—figure supplement 1.
-
Figure 1—source data 1
Expression and function of GABAB receptors and Cav2.3 at two parallel MHb-IPN pathways.
- https://cdn.elifesciences.org/articles/68274/elife-68274-fig1-data1-v2.xlsx
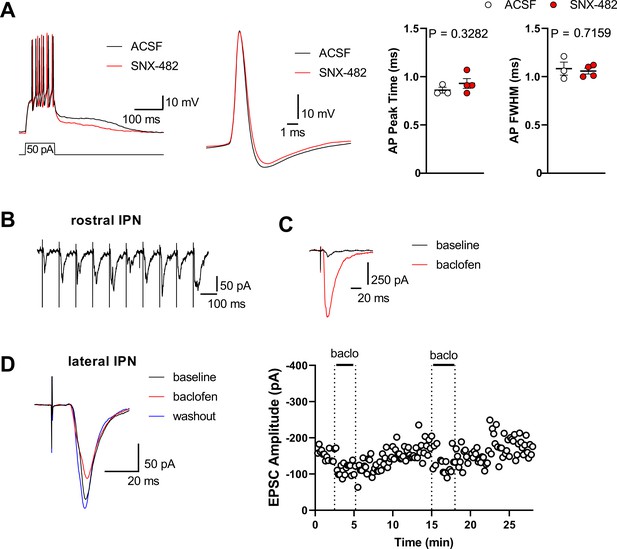
Effect of SNX-482 on action potential properties and confirmation of pathway specificity in thick slice recordings.
(A) Current clamp recording from ventral MHb neurons. Injection of 50 pA for 100 ms evoked trains of action potentials in the presence and absence of SNX-482 (1 µM). Neither peak times nor full-widths at half maximum (FWHM) of action potentials were affected by SNX-482. (B, C) Recording of electrically evoked EPSCs in a rostral IPN neuron in a thick slice from a wild-type mouse. (B) An example trace of EPSCs evoked by 10 Hz electrical stimulation of the fasciculus retroflexus. (C) Baclofen-mediated potentiation of the EPSC amplitude in the same neuron. (D) Example trace and time course of the application of baclofen on electrically evoked EPSCs in the same thick slice preparation recorded from a lateral IPN neuron. Baclofen reduced EPSC amplitude.
-
Figure 1—figure supplement 1—source data 1
Effect of SNX-482 on action potential properties and confirmation of pathway specificity in thick slice recordings.
- https://cdn.elifesciences.org/articles/68274/elife-68274-fig1-figsupp1-data1-v2.xlsx
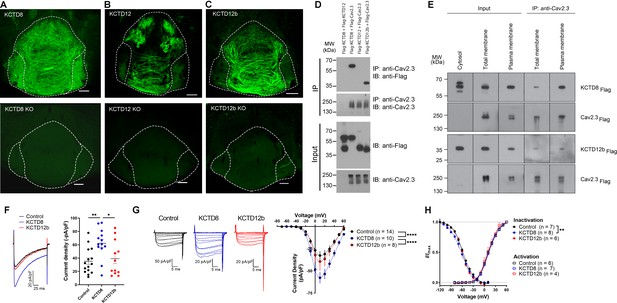
KCTD subtype expression in the IPN and interaction of Cav2.3 with KCTD8 and KCTD12b in vitro.
(A–C) Confocal images of immunofluorescence signals of KCTD8, KCTD12, and KCTD12b in the IPN of WT (upper panels) and respective KO mice (lower panels). KCTD8 immunofluorescence was present in all IPN subnuclei, whereas KCTD12 and KCTD12b signals were observed only in the rostral/central but not the lateral IPN subnuclei. Scale bars: 100 µm. (D) Co-immunoprecipitation from total cell lysates of HEK293T cells transfected with Flag-tagged KCTDs and Cav2.3. Immunoprecipitation of Cav2.3 co-precipitated KCTD8 and KCTD12b, but not KCTD12. Input lanes (bottom) indicate expression of the tagged proteins in the cell lysates. (E) KCTDs are co-localized and interact with Cav2.3 at the cell surface of HEK293T cells. The three input lanes to the right show expression of Flag-tagged KCTD8 (top) and Flag-tagged KCTD12b (bottom) in the cytosol, the total membrane fraction (‘total membrane’) and the plasma membrane fraction, from left to right. The two IP lanes to the left show that immunoprecipitation of Cav2.3 in the total membrane fraction (‘total membranes’) and the plasma membrane fraction co-precipitated KCTD8 (top) and KCTD12b (bottom), from left to right. Membrane-bound Cav2.3 (bottom lanes) is expressed in the total membrane fraction and the plasma membrane fraction, but absent from the cytosol fraction. (F) Whole-cell recordings from HEK293 cells stably expressing Cav2.3. Ba2+ current densities measured in response to a single depolarizing voltage step from −80 to 10 mV were significantly increased in KCTD8 co-transfected cells. *p<0.05, **p<0.01 one-way ANOVA with Tukey post hoc test. (G) Current density-to-voltage relationship demonstrating higher current densities in KCTD8-transfected cells compared with Control- and KCTD12b-transfected cells. ****p<0.0001 two-way ANOVA with Tukey post hoc test. (H) Activation and inactivation curves in Control-, KCTD8-, and KCTD12b-transfected cells. **p<0.01, two-way ANOVA with Tukey post hoc test. See also Figure 2—figure supplement 1.
-
Figure 2—source data 1
KCTD subtype expression in the IPN and interaction of Cav2.3 with KCTD8 and KCTD12b in vitro.
- https://cdn.elifesciences.org/articles/68274/elife-68274-fig2-data1-v2.xlsx

Quantification of KCTDs at the plasma and total membrane in HEK cells co-expressing Cav2.3, auxiliary β3 and α2δ1 subunits.
Fold increase of KCTD signal at the plasma membrane (PM) compared to the averaged KCTD signal in total membrane (TM).
-
Figure 2—figure supplement 1—source data 1
Quantification of KCTDs at the plasma and total membrane in HEK cells co-expressing Cav2.3, auxiliary β3 and α2δ1 subunits.
- https://cdn.elifesciences.org/articles/68274/elife-68274-fig2-figsupp1-data1-v2.xlsx
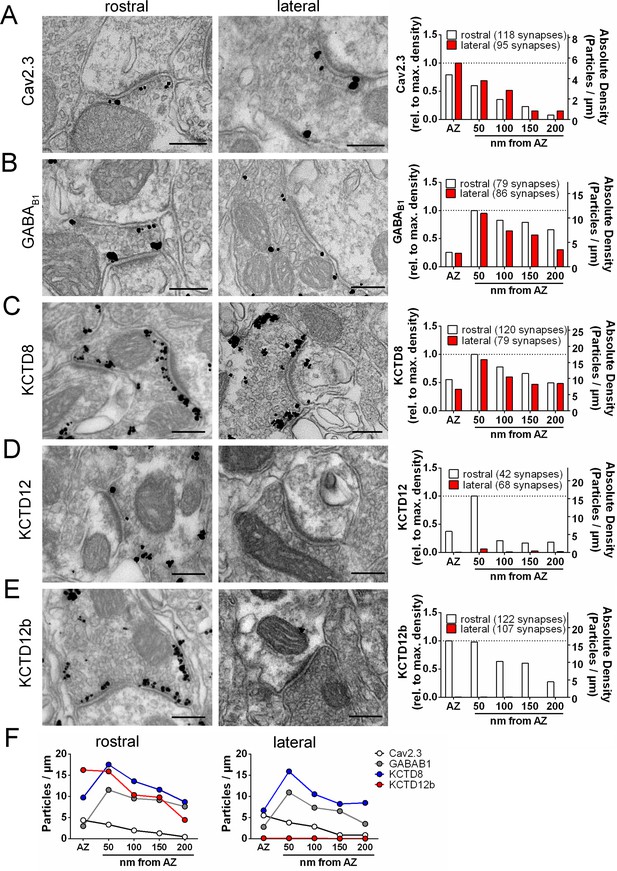
Quantification of the presynaptic localization of Cav2.3, GBRs, and KCTDs along the ventral and dorsal MHb-IPN pathways.
Transmission electron microscopy images of 70 nm thick sections following pre-embedding immunolabeled IPN slices for Cav2.3 (A), GABAB1 (B), KCTD8 (C), KCTD12 (D), and KCTD12b (E) from synapses in the rostral (left images) and lateral (right image) IPN subnuclei. Scale bars: 200 nm. Graph on the right displays quantification of relative and absolute silver-enhanced gold particle densities in the active zone and at distances of 50–200 nm from the edge of the active zone (50 nm bins). (F) Absolute labeling densities are summarized for synapses in the rostral (left) and lateral IPN (right). Note the absence of KCTD12 and KCTD12b particles in presynaptic terminals inside the lateral IPN subnuclei. KCTD12 was not included in panel F because of predominantly postsynaptic localization inside the rostral IPN. Data was pooled from two animals, showing no significant difference in gold particle distribution patterns with Kolmogorov-Smirnov test (see Figure 3—figure supplement 1 and Figure 3—figure supplement 2).
-
Figure 3—source data 1
Quantification of the presynaptic localization of Cav2.3, GBRs, and KCTDs along the ventral and dorsal MHb-IPN pathways.
- https://cdn.elifesciences.org/articles/68274/elife-68274-fig3-data1-v2.xlsx
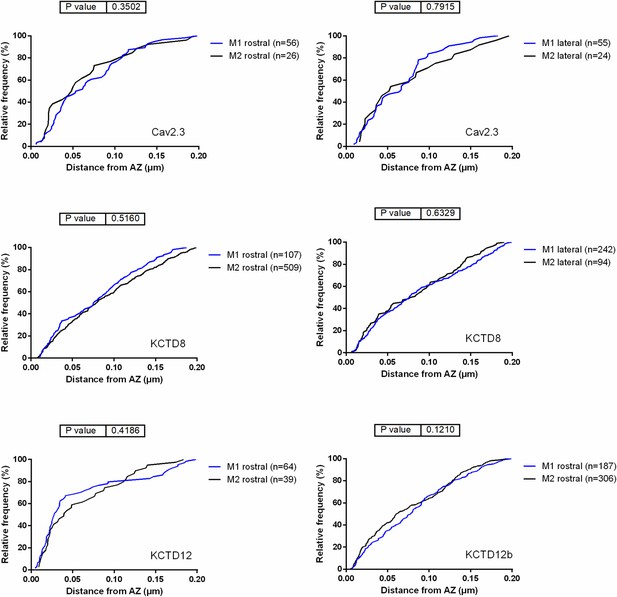
Comparison of distribution of silver-enhanced immunogold particles outside the active zone for Cav2.3, KCTD8, KCTD12, and KCTD12b in MHb terminals inside the rostral and lateral IPN.
Distribution of particles in samples from two mice was not significantly different. p-values above graph resulted from Kolmogorov–Smirnov test. M1 = mouse 1, M2 = mouse 2, n indicates the total number of particles analyzed.
-
Figure 3—figure supplement 1—source data 1
Comparison of distribution of silver-enhanced immunogold particles outside the active zone for Cav2.3, KCTD8, KCTD12, and KCTD12b in MHb terminals inside the rostral and lateral IPN.
- https://cdn.elifesciences.org/articles/68274/elife-68274-fig3-figsupp1-data1-v2.xlsx
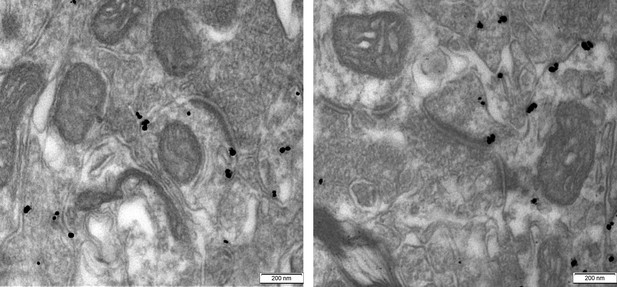
Two example pictures of postsynaptic immunogold labeling for KCTD12 in the rostral IPN.
Scale bar = 200 nm.
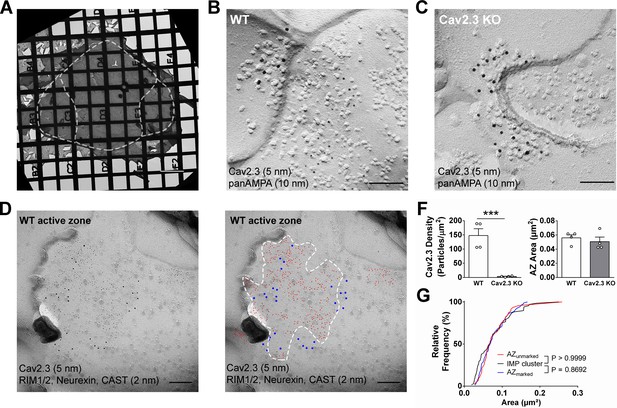
SDS-digested freeze-fracture replica labeling confirms Cav2.3 in the active zone of medial habenula terminals in the IPN.
(A) Example image of a grid-glued replica containing the whole IPN. White dashed line indicates demarcation of rostral/central and lateral subnuclei. Scale bar: 20 µm. (B) Example image of a presynaptic P-face and a postsynaptic E-face of a habenular synapse in the rostral IPN that was double labeled with antibodies against AMPA receptors (10 nm gold) and Cav2.3 (5 nm gold). Scale bar: 100 nm. (C) Example image of a similar synaptic profile double labeled with antibodies against AMPA receptors (10 nm gold) and Cav2.3 (5 nm gold) in the rostral IPN of a Cav2.3 KO mouse. Scale bar: 100 nm. (D) Left: double labeling of a WT carbon-only replica with antibodies against Cav2.3 (5 nm gold) and a mixture of active zone proteins (2 nm gold), including RIM1/2, CAST, and neurexin. Right: the same image with additional coloring of 2 nm (red) and 5 nm (blue) particles and demarcation of the active zone area based on active zone-marker labeling. Scale bars: 100 nm. (F) Left: quantification of Cav2.3 labeling densities in the presynaptic P-face in WT and Cav2.3 KO mice. ***p<0.001, unpaired t-test. Right: areas of demarcated active zones, including incomplete profiles, were not significantly different between replicas from WT and Cav2.3 KO mice. Data were obtained from four replicas from four mice of each genotype. (G) Comparison of active zone area demarcated with or without active zone markers and the size of the glutamatergic postsynaptic IMP clusters. p-value indicates result of Kolmogorov–Smirnov test.
-
Figure 4—source data 1
SDS-digested freeze-fracture replica labeling confirms Cav2.3 in the active zone of medial habenula terminals in the IPN.
- https://cdn.elifesciences.org/articles/68274/elife-68274-fig4-data1-v2.xlsx
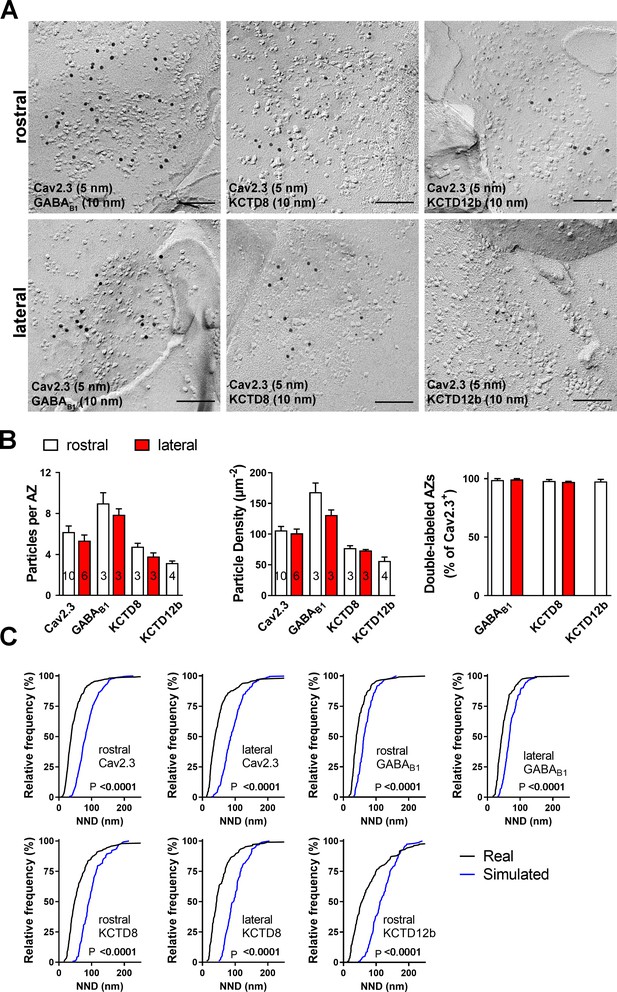
Co-localization of Cav2.3 with GBR and KCTDs in the active zone of medial habenula terminals.
(A) Active zones double labeled for Cav2.3 and either GABAB1 (left), KCTD8 (middle), or KCTD12b (right) in IPN replicas. Top row images are from presynaptic terminals in the rostral IPN; bottom row images are from presynaptic terminals in the lateral IPN. Scale bar: 100 nm. (B) Quantification of active zone immunolabeling in the rostral and lateral IPN. With the exception of the absence of KCTD12b in lateral IPN terminals, absolute particle numbers per active zone (left graph) and particle densities (middle graph) are comparable between MHb terminals in the rostral and lateral IPN. Right graph: Over 97% of active zones positive for Cav2.3 labeling also show labeling for one of the other molecules (GABAB1, KCTD8, or KCTD12b), suggesting co-localization of all presynaptic molecules inside the same active zone. Numbers inside the bars indicate the number of replicas used for each quantification. (C) Nearest-neighbor distance (NND) for all presynaptic molecules in MHb terminals inside the rostral and lateral IPN based on the real (black line) and simulated random distribution (blue line). Smaller NND values in real distributions compared to simulation suggest clustering of all presynaptic molecules. p-values calculated via Kolmogorov–Smirnov test.
-
Figure 5—source data 1
Co-localization of Cav2.3 with GBR and KCTDs in the active zone of medial habenula terminals.
- https://cdn.elifesciences.org/articles/68274/elife-68274-fig5-data1-v2.xlsx
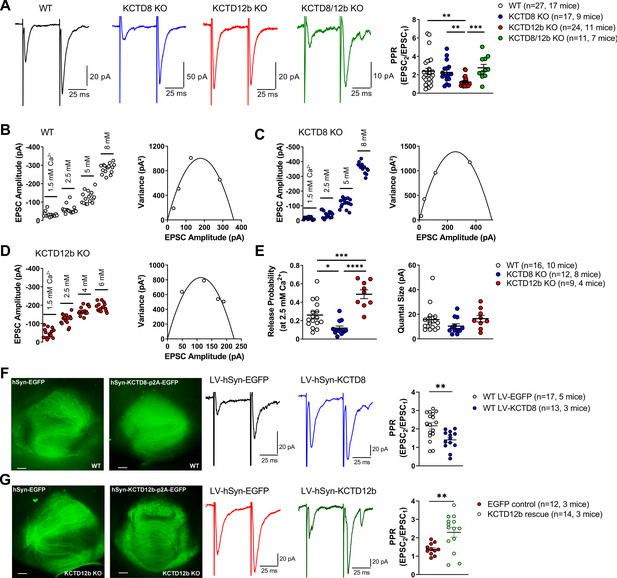
KCTDs modulate release probability of ventral MHb terminals.
(A) In whole-cell recordings from rostral IPN neurons, paired-pulse ratios (PPR) of electrically evoked glutamatergic excitatory postsynaptic currents (EPSCs) were significantly lower in KCTD12b KO mice compared with WT, KCTD8 KO, and KCTD8/12b double KO mice; **p<0.01, ***p<0.001, Kruskal–Wallis with Dunn’s post hoc test. (B–D) Example variance-mean measurements of electrically evoked EPSC amplitudes at varying external Ca2+ concentrations in recorded in rostral IPN neurons of WT (B), KCTD8 KO (C), and KCTD12b KO mice (D). (E) Quantification of release probability (left graph) and quantal size (right graph) indicates significantly reduced release probability in KCTD8 KO mice and increased release probability in KCTD12b KO mice. Quantal size remained unaffected. *p<0.05, ***p<0.001, ****p<0.0001 one-way ANOVA with Tukey’s post hoc test. (F) WT animals were injected with lentivirus expressing either EGFP (WT LV-EGFP) or KCTD8/EGFP (WT LV-KCTD8) into the MHb. Left: Example images of acute IPN slices after electrophysiological recordings showing EGFP signal in fibers inside the IPN. Right: Overexpression of KCTD8 resulted in significantly lower PPR values compared with EGFP controls **p<0.01, unpaired t-test. (G) Left: Lentiviral expression of EGFP or KCTD12b/EGFP in acute slices of KCTD12b KO mice. Right: Lentiviral expression of KCTD12b significantly increased PPR values compared with EGFP controls **p<0.01, unpaired t-test. See also Figure 6—figure supplement 1.
-
Figure 6—source data 1
KCTDs modulate release probability of ventral MHb terminals.
- https://cdn.elifesciences.org/articles/68274/elife-68274-fig6-data1-v2.xlsx
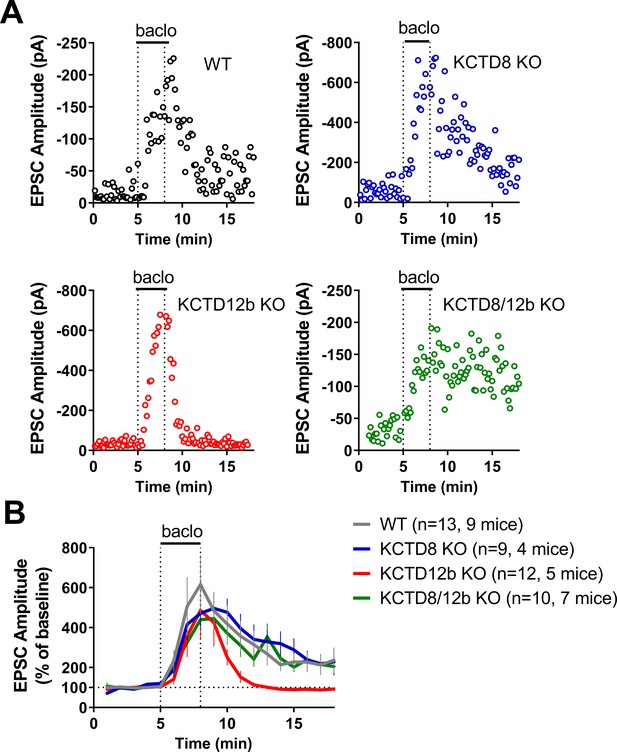
Baclofen still potentiated release in all KCTD KO lines.
(A) Example time courses of GBR-mediated potentiation in WT, KCTD8 KO, KCTD12b KO, and KCTD8/12b dKO mice. (B) Average time course of baclofen-mediated EPSC enhancement.
-
Figure 6—figure supplement 1—source data 1
Baclofen still potentiated release in all KCTD KO lines.
- https://cdn.elifesciences.org/articles/68274/elife-68274-fig6-figsupp1-data1-v2.xlsx
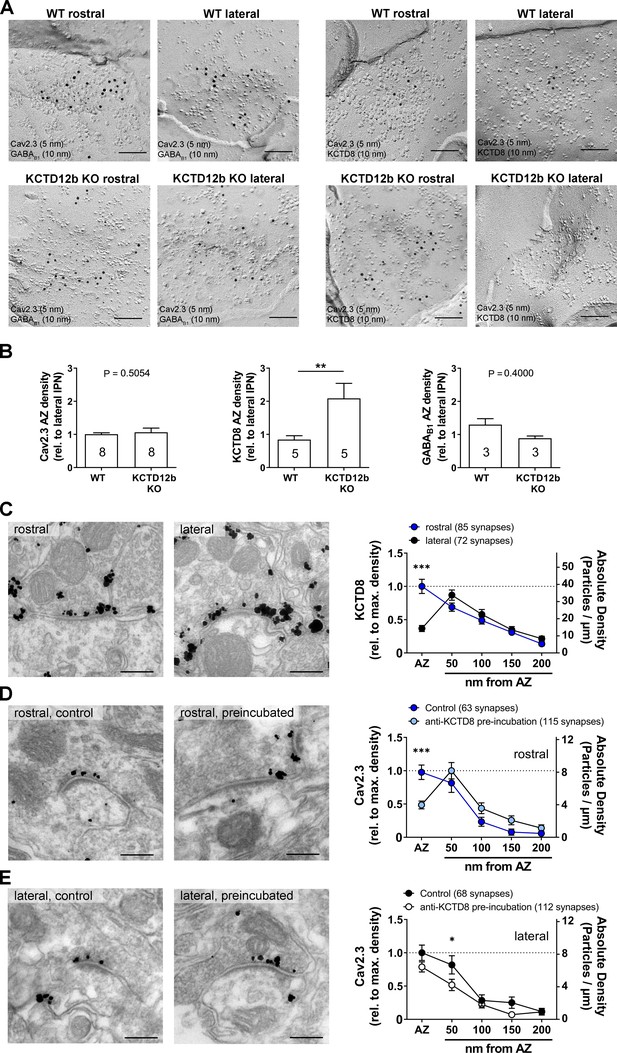
Absence of KCTD12b leads to a compensatory increase of KCTD8 inside the active zone of ventral MHb terminals.
(A) Example images of active zones containing Cav2.3 and either GABAB1 (left) or KCTD8 (right) in replicas of WT (upper row) and KCTD12b KO IPN tissue (lower row). Scale bars: 100 nm. (B) Quantification of relative densities for Cav2.3, KCTD8, and GABAB1 in active zones located in the rostral IPN of WT and KCTD12b KO mice. Densities were normalized to the average density in MHb terminals inside the lateral IPN of the same replica. The number inside the bars indicates the number of replicas used for quantification. **p<0.01 in a Mann–Whitney test. (C) Pre-embedding EM labeling for KCTD8 in KCTD12b KO mice (data from three mice). The density of KCTD8-labeled gold particles was significantly higher in the AZ of ventral MHb terminals in the rostral IPN compared with dorsal MHb terminals in the lateral IPN. ***p<0.0001 two-way ANOVA with Bonferroni post hoc test. (D, E) Comparison of pre-embedding EM labeling for Cav2.3 in MHb terminals in KCTD12b KO mice of control sections (standard labeling, four mice) with sections pre-incubated with anti-KCTD8 primary antibody and biotinylated secondary antibody (three mice). Pre-incubation resulted in significant reduction in labeling densities for Cav2.3 selectively in the active zone of ventral MHb terminals in the rostral IPN as well as in the peri-synaptic region of dorsal MHb terminals in the lateral IPN. ***p<0.001, *p<0.05 two-way ANOVA with Bonferroni post hoc test.
-
Figure 7—source data 1
Absence of KCTD12b leads to a compensatory increase of KCTD8 inside the active zone of ventral MHb terminals.
- https://cdn.elifesciences.org/articles/68274/elife-68274-fig7-data1-v2.xlsx
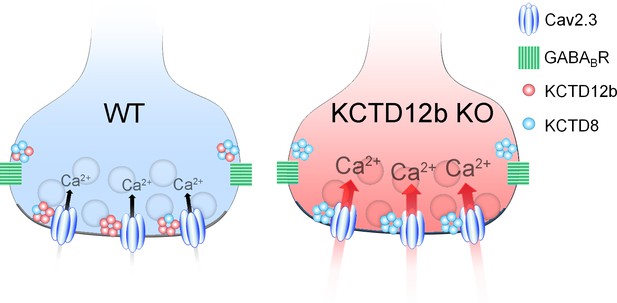
Summary of presynaptic localization of Cav2.3, KCTDs, and GBRs in ventral MHb neurons of WT and KCTD12b KO mice.
Schematic representation of the distribution and function of Cav2.3, GBRs, and KCTDs in ventral MHb terminals of WT and KCTD12b KO mice. Left: In WT terminals, the active zone contains Cav2.3 and hetero-pentameric rings comprising KCTD12b in excess over KCTD8, whereas KCTD8 and GBRs are located peri-synaptically. Right: In absence of KCTD12b, KCTD8 invades the active zone and compensates for the loss of KCTD12b, resulting in increased release probability, potentially via increased Ca2+ influx. See Figure 8—figure supplement 1.
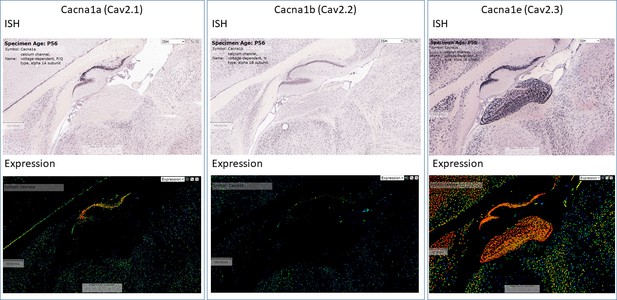
Allen Brain Atlas images showing lack of expression of Cav2.1 (http://mouse.brain-map.org/gene/show/12071) and Cav2.2 (http://mouse.brain-map.org/gene/show/12072) mRNA in MHb neurons, whereas Cav2.3 shows strong expression in MHb neurons (http://mouse.brain-map.org/gene/show/12075).
Tables
Reagent type (species) or resource | Designation | Source or reference | Identifiers | Additional information |
---|---|---|---|---|
Strain, strain background (Mus musculus, ♂) | C57BL/6J | The Jackson Laboratory | #000664 | |
Strain, strain background (Mus musculus, ♂) | BALB/cJ | The Jackson Laboratory | #000651 | |
Strain, strain background (Mus musculus, ♂) | Cav2.3 KO (gene: Cacna1e) | Prof. Tsutomu Tanabe, (Saegusa et al., 2000) | ||
Strain, strain background (Mus musculus, ♂) | KCTD8 KO (gene: Kctd8) | Prof. Bernhard Bettler, (Schwenk et al., 2010) | ||
Strain, strain background (Mus musculus, ♂) | KCTD12 KO (gene: Kctd12) | Prof. Bernhard Bettler, (Schwenk et al., 2010) | ||
Strain, strain background (Mus musculus, ♂) | KCTD12b KO (gene: Kctd12b) | Prof. Bernhard Bettler, (Schwenk et al., 2010) | ||
Strain, strain background (Mus musculus, ♂) | KCTD8/12b double KO (genes: Kctd8/Kctd12 b) | This paper | ||
Strain, strain background (Mus musculus, ♂♀) | Tac1-IRES-Cre (B6;129S-Tac1tm1.1(cre)Hze/J) | The Jackson Laboratory | #021877 | |
Strain, strain background (Mus musculus, ♂♀) | Ai32 (B6;129S-Gt(ROSA)26Sortm32(CAG-COP4*H134R/EYFP)Hze/J) | The Jackson Laboratory | #012569 | |
Strain, strain background (Mus musculus, ♂) | Tac1-ChR2-EYFP | Offspring of Tac1-IRES-Cre and Ai32 mice | ||
Genetic reagent (virus) | LV–hSyn–3xFlag/mKCTD8[NM_175519.5]/P2A/EGFP | VectorBuilder GmbH | Custom-made | |
Genetic reagent (virus) | LV–hSyn–3xFlag/mKCTD12b[NM_175429.4]/P2A/EGFP | VectorBuilder GmbH | Custom-made | |
Genetic reagent (virus) | LV–hSyn–EGFP | VectorBuilder GmbH | Custom-made | |
Cell line (human) | HEK293T | ATCC, (Seddik et al., 2012) | https://web.expasy.org/cellosaurus/CVCL_0063 | |
Cell line (human) | HEK293 cells stably expressing α1E-3 | Prof. David J. Adams, (Berecki et al., 2014) | ||
Antibody | Guinea pig polyclonal anti-Cav2.3 | Genovac, (Parajuli et al., 2012) | Custom-made antibody, 1 µg/ml for IHC, 8 µg/ml for SDS-FRL | |
Antibody | Rabbit polyclonal anti-GABAB1 | Prof. Akos Kulik, (Kulik et al., 2002) | Custom-made antibody, final concentration: (1 µg/ml) for IHC, (2 µg/ml) for SDS-FRL | |
Antibody | rabbit polyclonal anti-KCTD8 | Prof. Bernhard Bettler, (Schwenk et al., 2010) | Custom-made antibody, final concentration: (1 µg/ml) for IHC, (4 µg/ml) for SDS-FRL | |
Antibody | Rabbit polyclonal anti-KCTD12 | Prof. Bernhard Bettler, (Schwenk et al., 2010) | Custom-made antibody, final concentration: (1 µg/ml) for IHC, (4 µg/ml) for SDS-FRL | |
Antibody | Rabbit polyclonal anti-KCTD12b | Prof. Bernhard Bettler, (Schwenk et al., 2010) | Custom-made antibody, final concentration: (1 µg/ml) for IHC, (4 µg/ml) for SDS-FRL | |
Antibody | Rabbit polyclonal anti-RIM1/2 | Synaptic Systems | 140 203 | final concentration: (5 µg/ml) for SDS-FRL |
Antibody | Rabbit polyclonal anti-CAST | Prof. Watanabe, (Hagiwara et al., 2018) | final concentration: (3 µg/ml) for SDS-FRL | |
Antibody | Rabbit polyclonal anti-Neurexin | Prof. Watanabe, (Miki et al., 2017) | final concentration: (5 µg/ml) for SDS-FRL | |
Peptide, recombinant protein | SNX-482 | hellobio | HB1235 | |
Peptide, recombinant protein | ω-Conotoxin GVIA | Alomone Labs | 106375-28-4 | |
commercial assay or kit | Plasma membrane extraction kit | Abcam | ab 65400 | |
Chemical compound, drug | R(+)-Baclofen hydrochloride | Merck | G013 | |
Chemical compound, drug | 1(S),9(R)-(−)-Bicucullin-Methiodid | Merck | 14343 | |
Chemical compound, drug | Hexamethonium bromide | Tocris | 4111 | |
Chemical compound, drug | Mecamylamine hydrochloride | Tocris | 2843 | |
Chemical compound, drug | Cadmiumchloride | Merck | 202908 | |
Software, algorithm | Graphpad Prism 8 | Graphpad | https://www.graphpad.com/scientific-software/prism/ | |
Software, algorithm | MATLAB | MathWorks | https://www.mathworks.com/products/matlab.html?s_tid=hp_products_matlab | |
Software, algorithm | Reconstruct | John C. Fiala, Ph.D. | https://synapseweb.clm.utexas.edu/software-0 |
Additional files
-
Supplementary file 1
Contains Table 1 showing parameters of the Boltzmann fit shown in Figure 8C.
Gmax is the maximal conductance density. *Gmax was significantly increased in KCTD8-transfected cells compared with Control (p=0.0340, one-way ANOVA with Tukey post hoc test); Vrev is the reversal potential; V0.5 act is the potential at which current density was half-maximal; Kα is the slope factor.
- https://cdn.elifesciences.org/articles/68274/elife-68274-supp1-v2.docx
-
Transparent reporting form
- https://cdn.elifesciences.org/articles/68274/elife-68274-transrepform-v2.docx