Inhibitors of the small membrane (M) protein viroporin prevent Zika virus infection
Figures
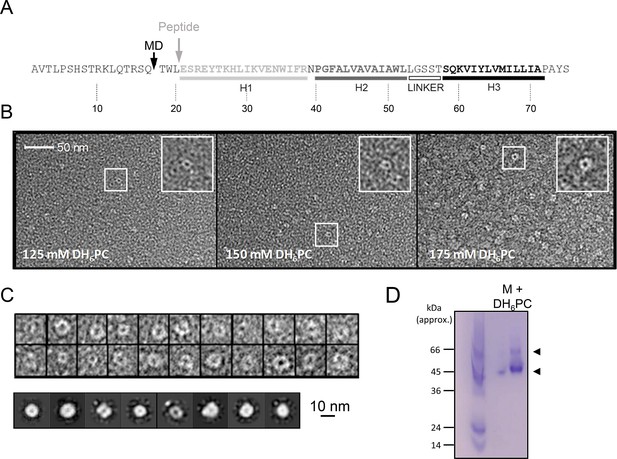
in vitro characterisation of M.
(A) M sequence showing helical regions and peptide truncation (in vitro synthesis (grey arrow) and molecular dynamics (MD) simulations (black arrow)). (B) Visualisation of M peptide with increasing concentrations of detergent, stained with uranyl acetate. Fields are representative of multiple images with~9000 particles collected in total across all conditions. Insets show zoomed images of particles with accumulation of stain within central cavity, consistent with channel formation. (C) Top panel – examples of particles visualised at 150mM illustrating heterogeneity. 9907 particles from 150mM samples were resolved into two-dimensional (2D) class averages with 25 iterations (bottom panel) yet rotational symmetry could not be determined. (D) Native polyacrylamide gel electrophoresis (PAGE) of M peptide (5μg) reconstituted in DH6PC (300mM).
-
Figure 1—source data 1
TIFF file of scanned native polyacrylamide gel electrophoresis (PAGE) gel photographed after staining with Coomassie Blue.
Wells not featured in the main figure contained detergents that were unable to reconstitute M peptides properly, namely (n-dodecyl-β-D-maltoside) DDM and (lysomyristoylphosphatidylglycerol, aka ‘Lyso PG’) LMPG.
- https://cdn.elifesciences.org/articles/68404/elife-68404-fig1-data1-v2.zip
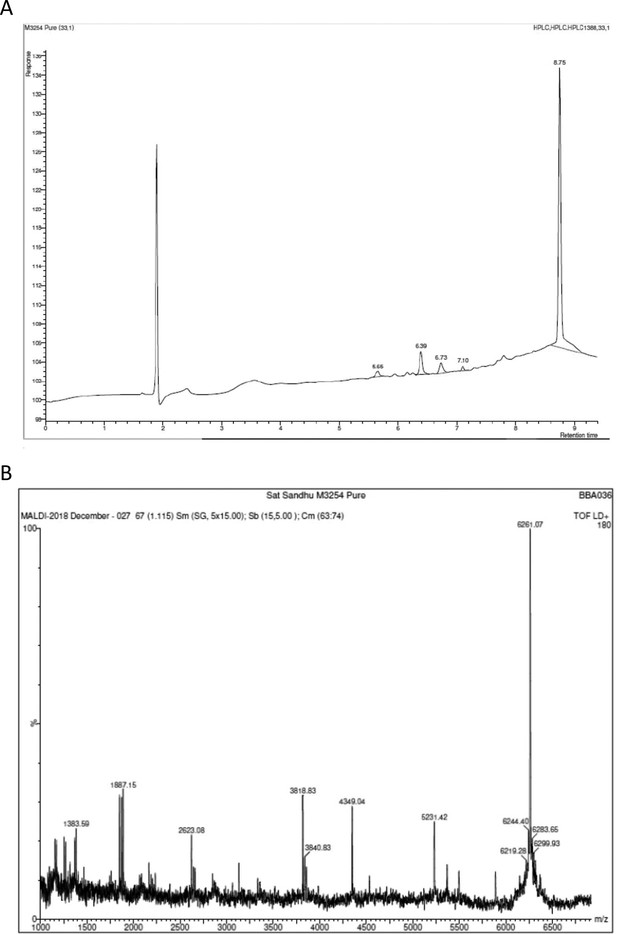
Example High Performance Liquid Chromatography (HPLC) and mass spectrometry analysis of synthetic M peptides supplied by Alta Biosciences.
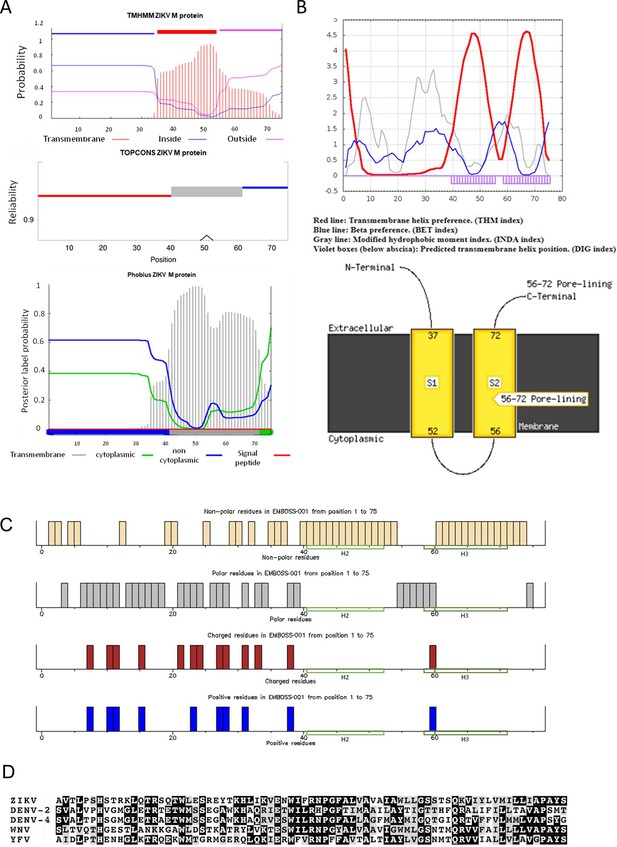
Predicted membrane protein properties for ZIKV M protein.
(A) Prediction of M protein trans-membrane regions, topology and hydrophobic profiles generated in different online server packages where single trans-membrane domain (TMD) topology was supported: TMHMM, TOPCONS, and Phobius. (B) As for A, but dual TMD topology: SPLIT v4.0 and MEMSAT-SVM. (C) M sequence amino acid characteristics visualised using EMBOSS Pepinfo. (D) Alignment of consensus Flavivirus M protein sequences, black – 100% conserved, grey – conserved amino acid properties.
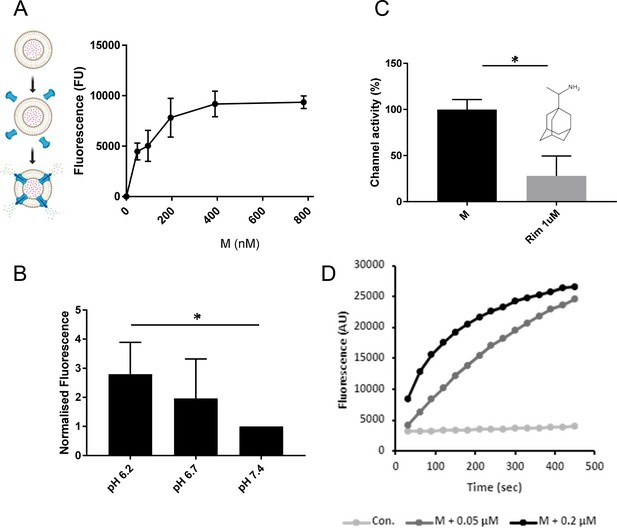
M induces rimantadine-sensitive membrane permeability, augmented by reduced pH.
(A) Titration of Dimethyl Sulphoxide (DMSO)-reconstituted M peptide in endpoint liposome carboxyfluorescein (CF) release assay. Graph represents a single biological repeat representative of at least three others, comprising triplicate technical repeats at each concentration. Error bars show standard deviation. (B) Modified endpoint CF assay undertaken with altered external buffer pH as indicated. CF content of re-buffered clarified assay supernatants were detected as a single endpoint measurement. Data comprise three biological repeats for each condition, error bars show normalised standard error, *p ≤ 0.05, Student’s t-test. (C) Effect of rimantadine (1μM) upon M activity. Results are from three biological repeats normalised to 100% activity for solvent control. Error bars show adjusted % error. *p ≤ 0.05, Student’s t-test. (D) Real-time CF assay data for M peptides in ‘virion-sized’ liposomes, produced by extrusion through a 0.05-μm, rather than a 0.2-μm filter.
© 2024, BioRender Inc. Panel A was created using BioRender, and is published under a CC BY-NC-ND license. Further reproductions must adhere to the terms of this license.
-
Figure 2—source data 1
Prism or Excel files containing summary endpoint (A–C) or real-time (D) fluorescence data for dye release assays.
- https://cdn.elifesciences.org/articles/68404/elife-68404-fig2-data1-v2.zip
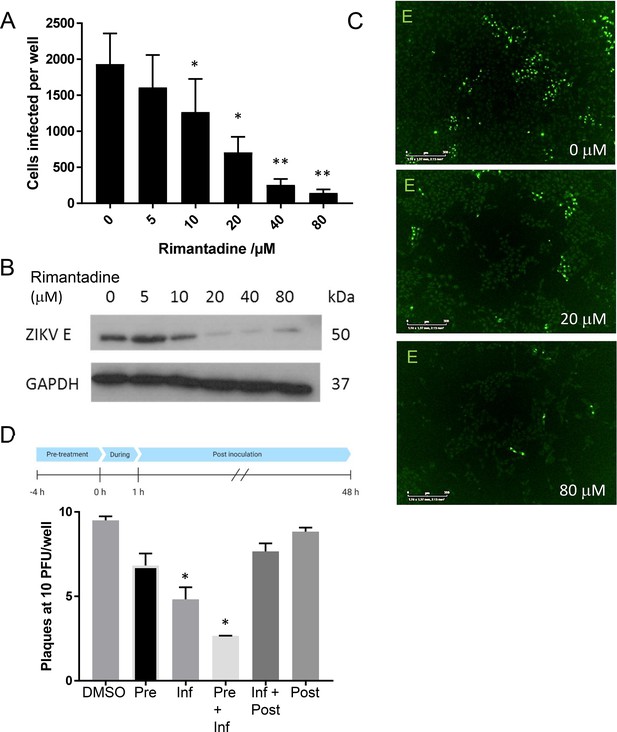
Rimantadine blockade of ZIKV infection.
(A) Vero cells were infected at a multiplicity of infection (MOI) of 0.1 pfu/cell with increasing rimantadine concentrations in 96-well plates. Infectious units were quantified 48hr post-infection using an IncuCyte Zoom to count ZIKV infected cells stained with antibody to ZIKV E and an Alexafluor 488nm secondary antibody. Counts were averaged over four image panels per well and results are from three biological replicates performed in triplicate. Error bars represent standard error of the mean between experiments. *p ≤ 0.01, **p ≤ 0.001, paired Student’s t-test relative to solvent control. (B) Representative anti-E western blot of n = 3 biological repeats of 6-well plate experiments run in parallel to fluorescence-based assays. Infections and timings as for A. (C) Example representative fluorescence images from IncuCyte analysis shown in A, scale bars represent 500μm. (D) Plaque-reduction assay using BHK21 cells infected with 10 pfu/well in the presence of 80μM rimantadine during different stages of infection shown by schematic. N = 2 biological replicates. Error bars represent standard error of the mean between experiments, *p ≤ 0.01, paired Student’s t-test relative to solvent control.
-
Figure 3—source data 1
Prism files summarising fluorescence focus assays for rimantadine-mediated inhibition during multi-step infection, or time of addition assays.
Also original scan PDF for B and loading control western blots.
- https://cdn.elifesciences.org/articles/68404/elife-68404-fig3-data1-v2.zip
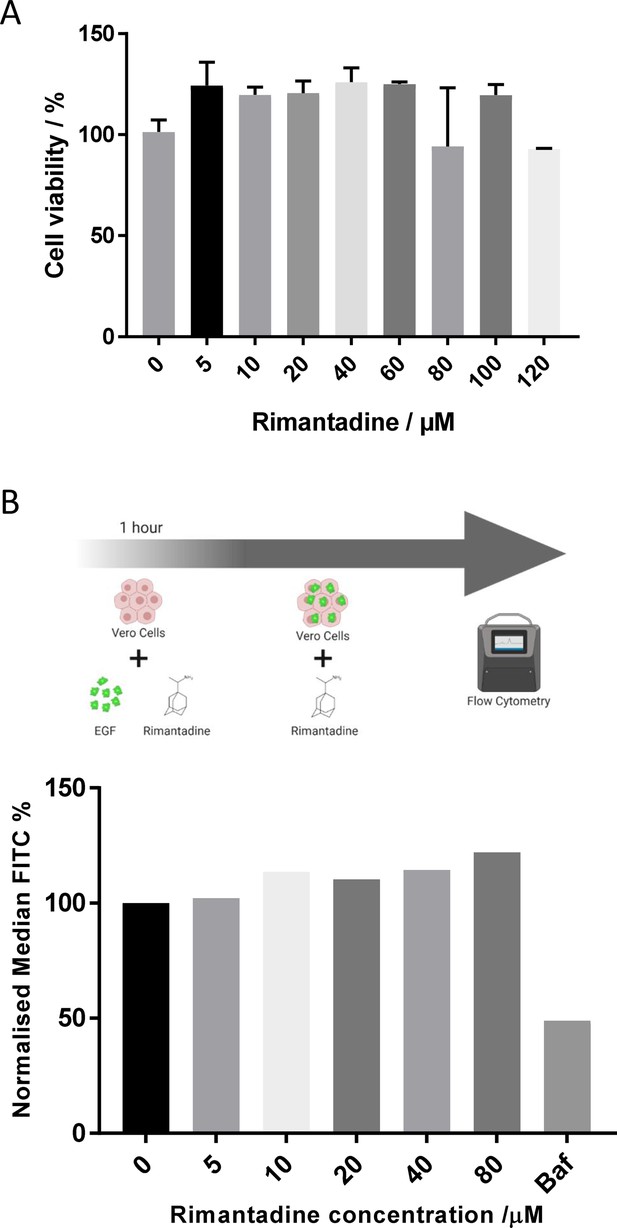
Controls for off-target effects of rimantadine during infectious ZIKV culture.
(A) Viability of Vero cells measured using (3-(4,5-dimethylthiazol-2-yl)-2,5-diphenyltetrazolium bromide) MTT assay after 48hr incubation with rimantadine or DMSO control for 48hr. Graph summarises a single representative biological repeat with three technical repeats. Error bars represent one standard deviation. (B) Lack of effect of rimantadine upon uptake of fluorescently labelled EGF by endocytosis. Top – experimental schematic. Bottom – endocytic uptake of fluorescently labelled (FITC) EGF in Vero cells pre-treated with 5–80μM rimantadine. Uptake was measured using flow cytometry and quantified in 25,000cells per condition. Data is expressed as median FITC, displayed as a % of maximum fluorescent EGF uptake at 0μM rimantadine. Bafilomycin A1 was used at 1μM as a positive control for blocking endocytic uptake.
© 2024, BioRender Inc. Panel B was created using BioRender, and is published under a CC BY-NC-ND license. Further reproductions must adhere to the terms of this license.
-
Figure 3—figure supplement 1—source data 1
Prism files with data for rimantadine MTT assays and fluorescent EGF uptake assays.
- https://cdn.elifesciences.org/articles/68404/elife-68404-fig3-figsupp1-data1-v2.zip
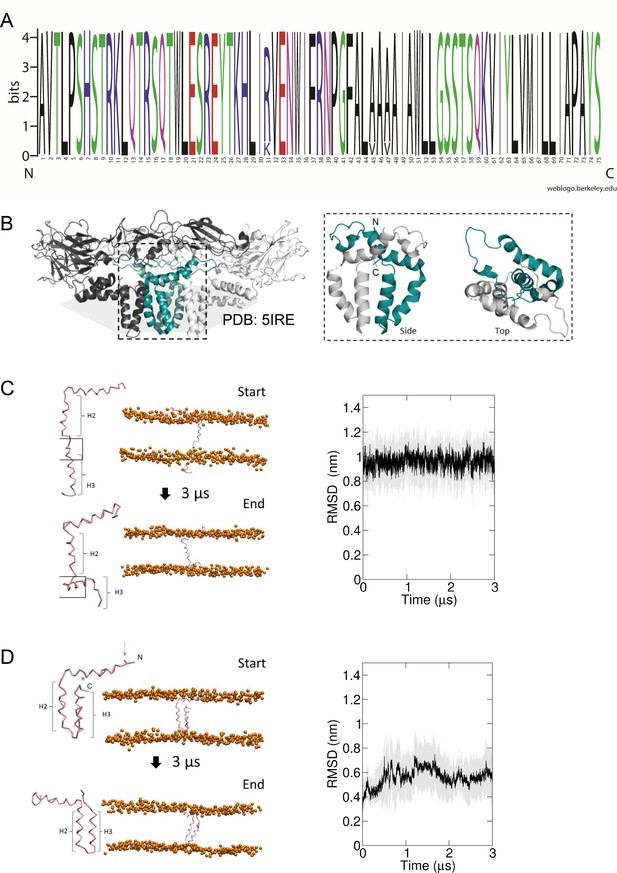
Predicted membrane topology for M protomers.
(A) M sequence ‘logo’ (https://weblogo.berkeley.edu/logo.cgi) showing relative conservation at each amino acid position. 929 sequences were retrieved by a Uniprot search for ‘Zika virus’, then aligned using CLUSTAL Omega. Alignments were exported to Jalview where removal of partial/irrelevant sequences resulted in analysis of~700 sequences. Conservation is high (>95%) across the majority of the protein, with the exception of Arg31, Ala45, and Ala47, but even these remain present within>80% sequences. (B) Image of an energy minimised M protomer taken from PDB: 5IRE within a simulated hydrated lipid membrane system (see methods). (C) Straightened, single TM M protomer inserted into a POPC bilayer and subjected to coarse-grained (CG) simulations for 3 μs, running from start (top) to finished (bottom) pose. (root mean square deviation) RMSD over time (five repeat simulations, standard deviations in grey) showed a high degree of movement and flexibility exhibited by this conformation (~1nm throughout), as the protein appears to attempt moving the C-terminal helix (H3) back along the plain of the membrane. Into close proximity of the bilayer. (D) By comparison, the cryo-EM-derived conformer structure was simulated in a model bilayer as for C. This conformation remained stable for the duration of the simulation (five repeat simulations, standard deviations in grey).
-
Figure 4—source data 1
Folder containing all ZIKV sequences obtained from Uniprot, aligned in ClustalW and visualised using Jalview.
Also, RMSD plots with errors shown for single/dual membrane-pass M protomers with error shown in grey.
- https://cdn.elifesciences.org/articles/68404/elife-68404-fig4-data1-v2.zip
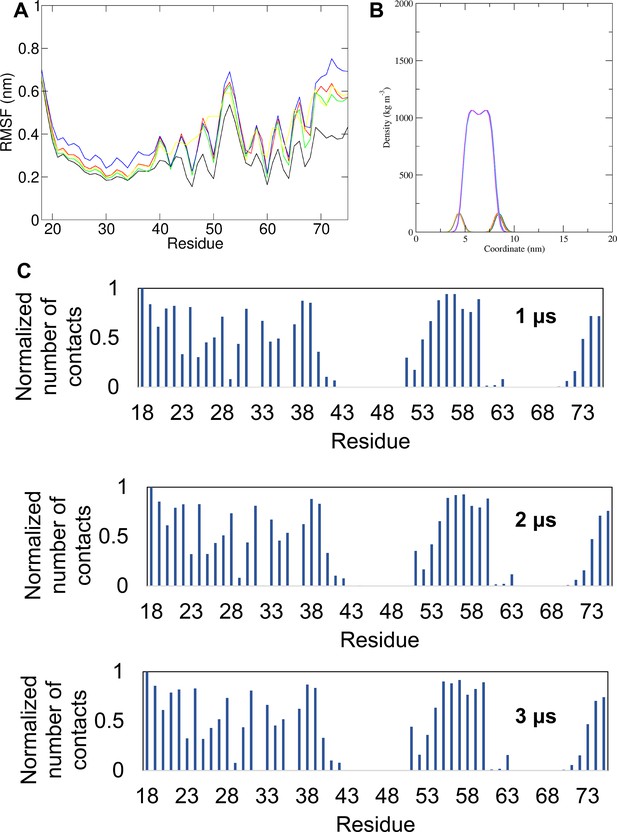
Simulation data for straightened M protein monomer in POPC membranes.
(A) Root mean square fluctuation (RMSF) per residue for simulations in which the two transmembrane helices were straightened into a membrane-spanning helix containing a small unstructured region between helices 2 and 3, prior to insertion into a POPC membrane; this was designed to emulate secondary structure predictions where a single trans-membrane domain (TMD) was predicted. The different colours represent different simulations. (B) Density profiles along the membrane normal of the POPC headgroup and the POPC tails for the same simulations. The different colours represent different simulations. (C) Normalised average number of contacts between the protein and the POPC lipid headgroup across all repeat simulations of the simulation in which the cryo-EM-derived conformer was inserted in a POPC membrane shown for 1 μs of the simulation ensemble, 2 μs of the simulation ensemble and for all the simulation ensemble (3 μs). For the normalisation the number of contacts of each residue with POPC headgroup was divided by the largest number of contacts.
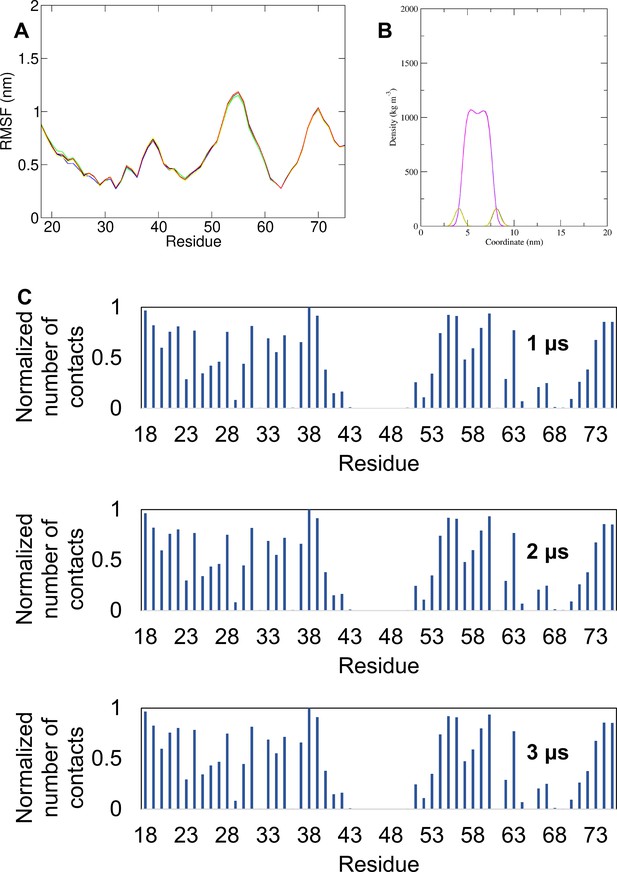
Simulation data for double trans-membrane cryo-EM derived M conformer in POPC membranes.
(A) Root mean square fluctuation (RMSF) per residue for the simulations in which the cryo-EM-derived conformer (i.e. double trans-membrane domain [TMD] monomeric M from PDB: 5IRE) was inserted in a POPC membrane. The different colours represent different simulations. (B) Density profiles along the membrane normal of the POPC headgroup and the POPC tails for the same simulations. The different colours represent different simulations. (C) Normalised average number of contacts between the protein and the POPC lipid headgroup across all repeat simulations of the simulation in which the cryo-EM-derived conformer was inserted in a POPC membrane shown for 1 μs of the simulation ensemble, 2 μs of the simulation ensemble and for all the simulation ensemble (3 μs). For the normalisation, the number of contacts of each residue with POPC headgroup was divided by the largest number of contacts.
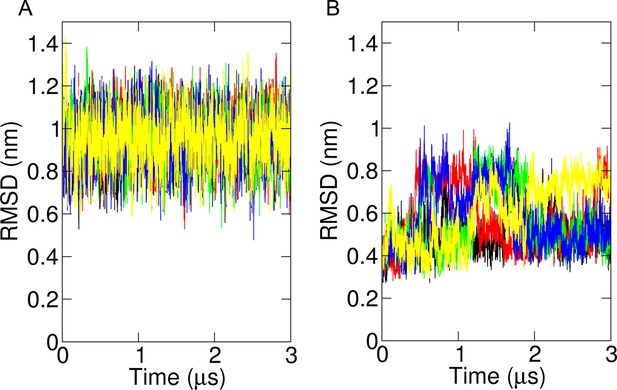
RMSD over time from simulations shown in Figure 4C, D, highlighting each of the five repeats as different colours.
(A) Simulations in which the two trans-membrane domains (TMDs) were straightened into a membrane-spanning helix containing a small unstructured region between helices 2 and 3. (B) Simulations with the cryo-EM-derived conformer.
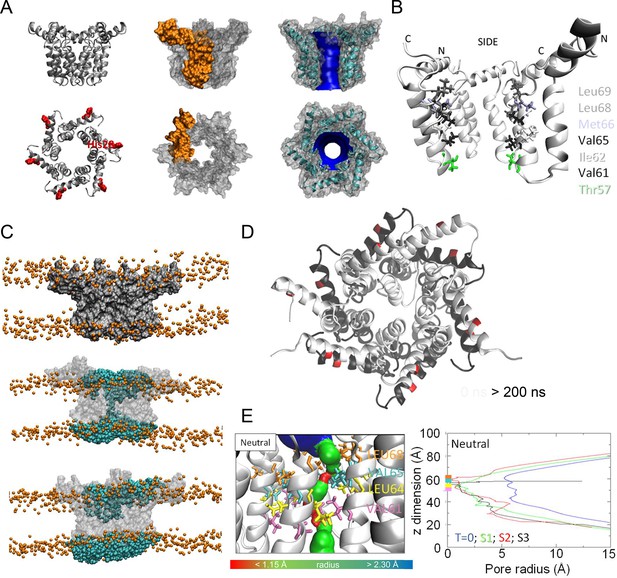
Simulated hexameric M channel complex in a compact formation, lined by helix 3.
(A) Assembly of protomers into compact hexamer model using Maestro. Left – ribbon diagram illustrating position of His28 (red) in helix 1 for orientation; middle – space filling model showing individual protomer (gold); right – space filling model showing pore diameter using HOLE. (B) Cutaway of channel viewed from the side showing pore-lining residues predicted for the compact hexamer from the side. (C) Snapshots of compact hexamers in POPC bilayers from a representative atomistic simulation showing the energy minimised system at t = 0 (top), formation of a conductive water column during early times and eventual closing of the channels. (D) Compact hexameric complex over a 200-ns atomistic simulation overlaying start (white) and endpoint (black) conformations. (E) HOLE profiles for lumenal aperture showing differences between starting configuration (blue line) and final structures over three separate 200 ns atomistic simulations (green, red, and black) at neutral external pH.
-
Figure 5—source data 1
Folder containing MP4 files of two ‘Compact hexamer, H3’ simulations.
Prism file detailing effect of acidic pH upon M channel activity for both WT and His28 peptides.
- https://cdn.elifesciences.org/articles/68404/elife-68404-fig5-data1-v2.zip
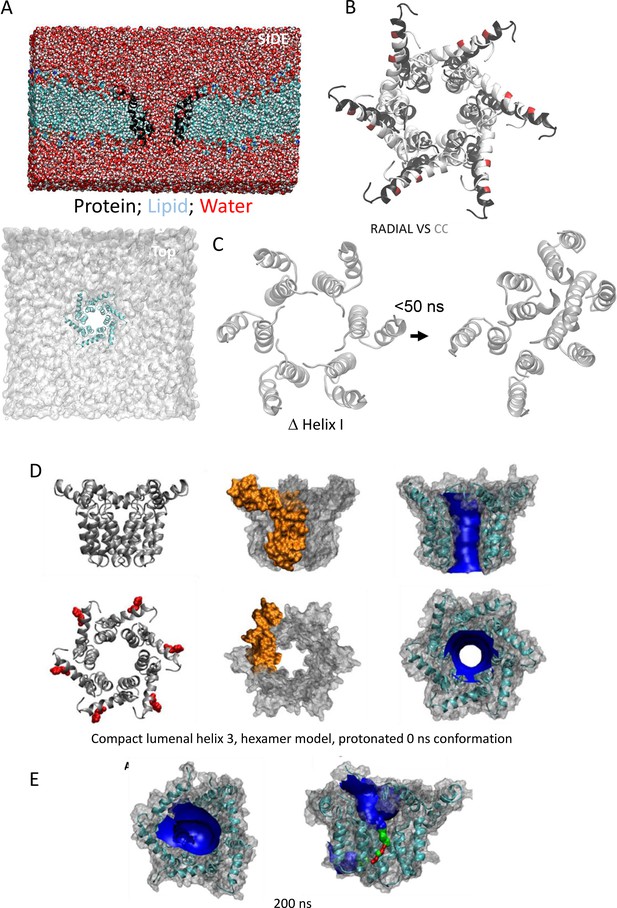
MD simulations of hexameric conformers under different conditions.
(A) Simulation system box for M protein hexamers in hydrated POPC bilayers, visualised from the side (protein: black; lipids: green and silver; water red and silver), or the top (protein: cyan; lipid: grey). (B) Overlay of compact (white) versus radial (black) hexamer conformation at neutral pH. (C) Simulation of M complexes where the N-terminal helix was removed, leading to rapid (<50ns) dissociation of the channel complex. (D) Starting conformations for His28++ compact hexamer with lumenal helix 3, showing high similarity to non-protonated forms. (E) Structures of protonated channels at 200 ns including HOLE profile of channel lumen.
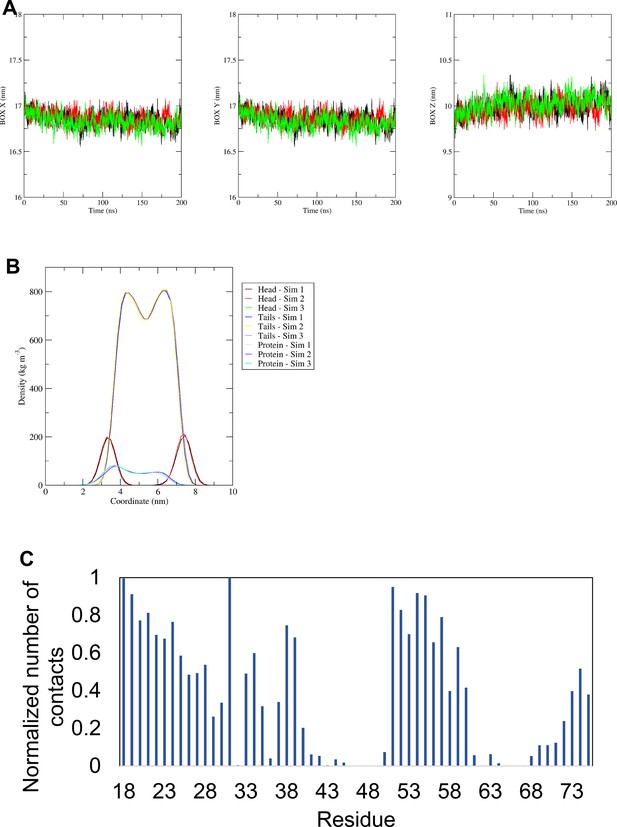
MD simulation data for "radial" hexameric conformers.
(A) Size (in nm) of the X, Y, and Z sites of the simulation box for the simulations in which helix 1 was orientated almost perpendicular to the channel pore with helix 3 facing towards the pore (radial) of the hexameric channel. (B) Density profiles along the membrane normal of the POPC headgroup, the POPC tails, and of the proteins for the same simulations. In A and B, the different colours represent different simulations. (C) Normalised average number of contacts between the protein and the POPC lipid headgroup across all repeat simulations of the same simulation. For the normalisation, the number of contacts of each residue with POPC headgroup was divided by the largest number of contacts.
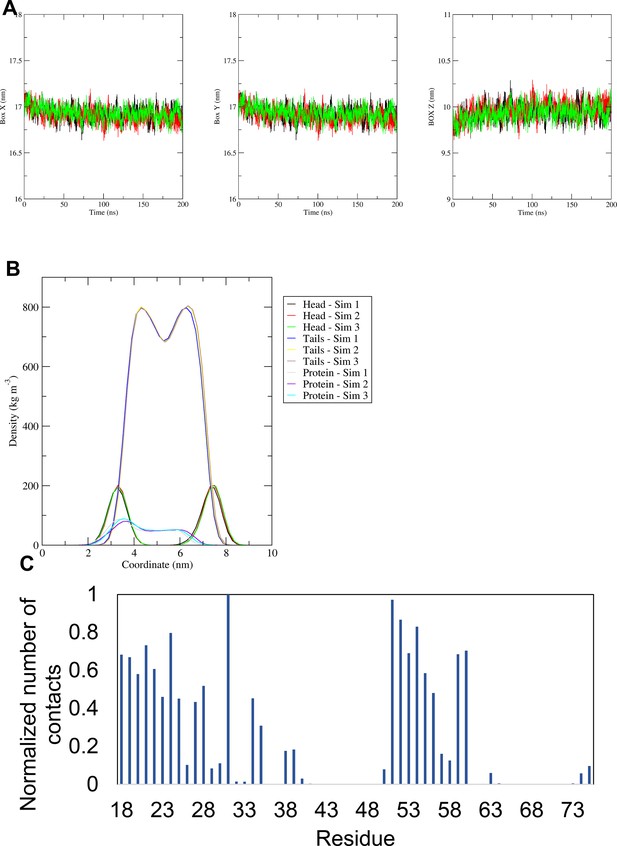
MD simulation data for "compact" hexameric conformers.
(A) Size (in nm) of the X, Y, and Z sites of the simulation box for the simulations with the more compact structure (compact) of the hexameric channel. (B) Density profiles along the membrane normal of the POPC headgroup, the POPC tails, and of the proteins for the same simulations. In A and B, the different colours represent different simulations. (C) Normalised average number of contacts between the protein and the POPC lipid headgroup across all repeat simulations of the same simulation. For the normalisation, the number of contacts of each residue with POPC headgroup was divided by the largest number of contacts.
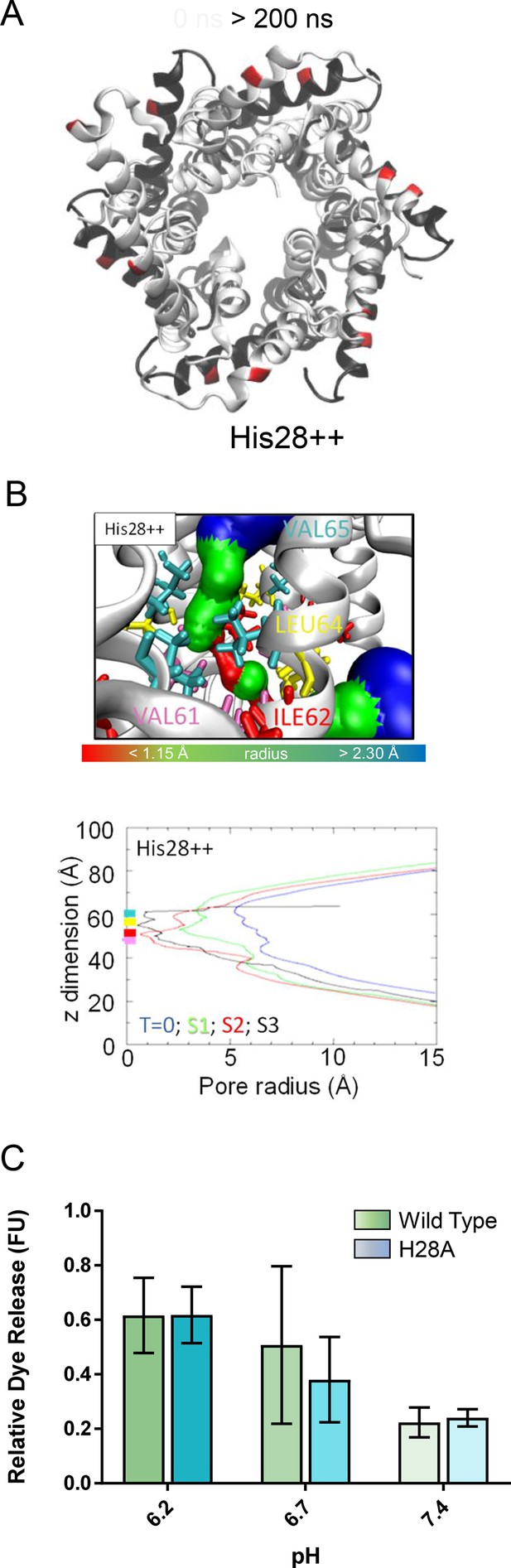
Exploration of mimicking acidic external pH via dual His28 protonation (His28++).
(A) Compact hexameric His28++ complex over a 200-ns atomistic simulation overlaying start (white) and endpoint (black) conformations. (B) HOLE profiles for lumenal aperture showing differences between starting configuration (blue line) and final structures over three separate 200-ns atomistic simulations (green, red, and black) where His28++ mimickedlow external pH. (C) Repeat of pH experiment in Figure 2B comparing wild-type (green) and His28Ala (blue) peptides. Results support that His28 is not responsible for pH activated increases of in vitro membrane permeability.
-
Figure 5—figure supplement 4—source data 1
Prism file for dye release assay data.
- https://cdn.elifesciences.org/articles/68404/elife-68404-fig5-figsupp4-data1-v2.zip
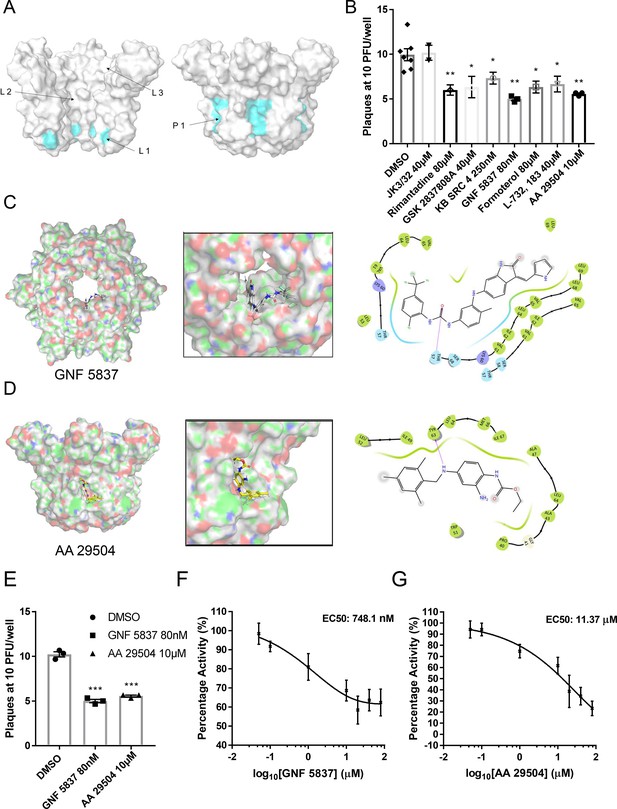
Inhibition of ZIKV replication by in silico enriched repurposed drugs.
(A) Compact M channel complex models were assessed using the SiteMap package within Maestro for cavities corresponding to high druggability scores. Three sites were found within the lumen (L1–L3),with L1 retaining the most favourable score. An additional site upon the membrane-exposed channel periphery was also evident (P1),yet would not score highly in SiteMap due to solvent exposure of compounds during simulated docking. Notably, both L1 and P1 reside within close proximity to the predicted gating region near Leu64. (B) Compounds selected to bind the L1 and P1 sites in silico and screened in vitro (Figure 6—figure supplement 2) were tested for effects in ZIKV culture, using a BHK21 cell plaque-reduction assay. Compounds were used at concentrations shown not to evoke cellular toxicity by MTT assays (Figure 6—figure supplement 3). Experiments are biological triplicates with error bars representing the standard error of the mean. **p ≤ 0.01, *p ≤ 0.05, Student’s t-test. (C) Predicted binding pose of GNF 5837 at L1 site generated using Glide (Maestro, Schrodinger) with associated binding site interactions. (D) As C, but for AA 29504. (E) Example biological repeat with triplicate technical repeats for the two top compounds targeting the L1 and P1 sites, GNF 5837 (L1)and AA 29504 (P1). (F) In vitro dye release assays showing 8-point log2-fold titration of GNF 5837 enabling calculation of 50% effective concentration (EC50). (G) As F, for AA 29504.
-
Figure 6—source data 1
Prism and excel files for both plaque assay and in vitro dye release EC50 experiments using lead lumenal and peripherally targeted M inhibitors.
- https://cdn.elifesciences.org/articles/68404/elife-68404-fig6-data1-v2.zip
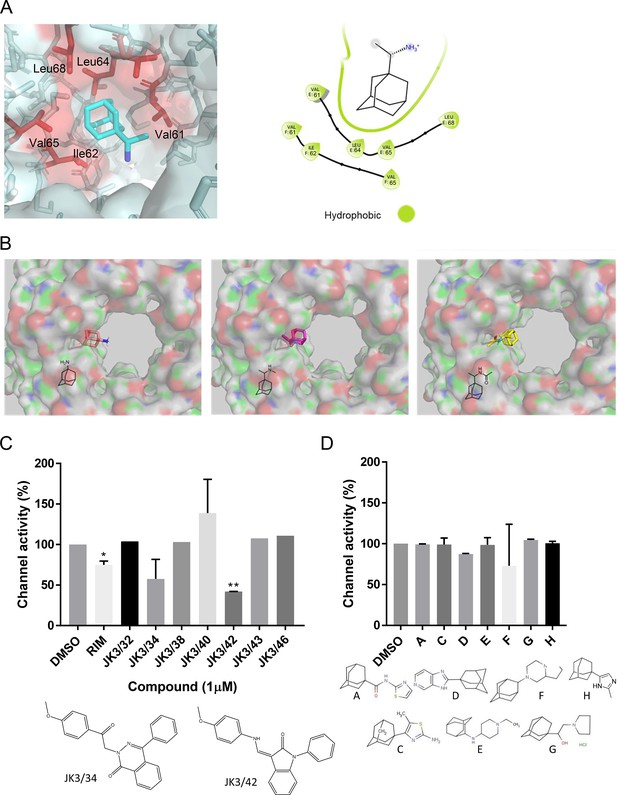
Lack of inhibitory activity for other adamantane derivatives versus M in carboxyfluorescein (CF) release assays.
(A, B) Rimantadine and derivatives showed overlapping binding modes at the L1-binding site, but derivatives adopted opposite orientations such that the hydrophobic adamantyl cage was predicted to project into the lumen. (C) Compounds targeting a peripheral site upon the hepatitis C virus (HCV) p7 channel complex were tested using CF release assays to ascertain the potential relevance of the ostensibly similar P1 site and would be unlikely to occupy the lumenal sites as a result; these have a more planar structure compared with rimantadine. Two compounds (JK3/34 and JK3/42) were effective at inhibiting M channel activity, distinct from the HCV series lead (JK3/32), which had no effect. (D) In contrast, a series of adamantane derivatives shown previously to have variable efficacy against both M2 and p7 had no effect upon M channels in dye release assays. *p≤0.05, **p≤0.01 Student's t-test. Error bars represent standard deviations based upon three technical replicates.
-
Figure 6—figure supplement 1—source data 1
Prism file for liposome dye release assay using M peptides with adamantane analogues and JK series compounds.
- https://cdn.elifesciences.org/articles/68404/elife-68404-fig6-figsupp1-data1-v2.zip
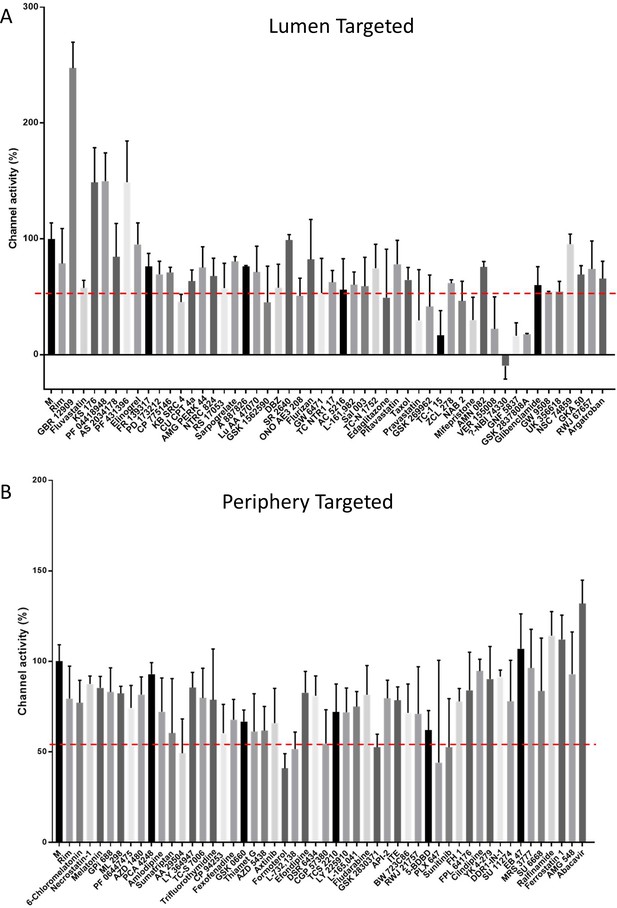
Screening data for lumenally (A) and peripherally targeted (B) M-binding compounds, enriched using in silico high-throughput screening (HTS). The top 50 compounds based upon predicted binding scores and drug-like properties derived from the TOCRIS repurposing library were tested versus M at 1μM using carboxyfluorescein (CF) release assays. Results show the average of three biological repeats, with error bars representing the standard error of the mean.
-
Figure 6—figure supplement 2—source data 1
Prism file with data for repurposing library screen using dye release assays.
- https://cdn.elifesciences.org/articles/68404/elife-68404-fig6-figsupp2-data1-v2.zip
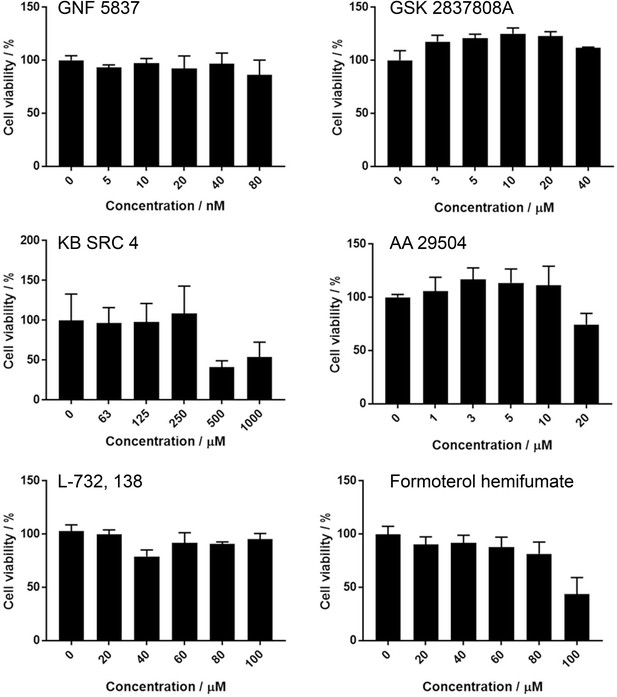
Effects of screening hits upon cell metabolic activity measured by MTT assay in Vero cells.
Compound titrations were performed according to remain below cytotoxic doses described in the literature. Results show the average of three biological repeats, with error bars representing the standard error of the mean.
-
Figure 6—figure supplement 3—source data 1
Prism file with data for hit compound MTT assays in BHK cells.
- https://cdn.elifesciences.org/articles/68404/elife-68404-fig6-figsupp3-data1-v2.zip
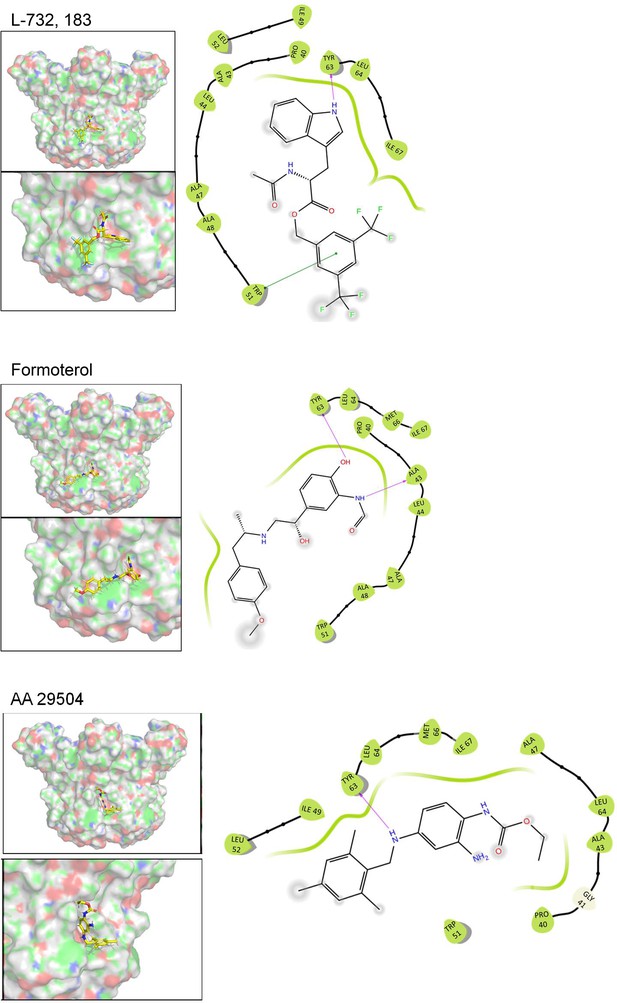
Predicted binding poses and residue interactions for peripherally targeted hit compounds.
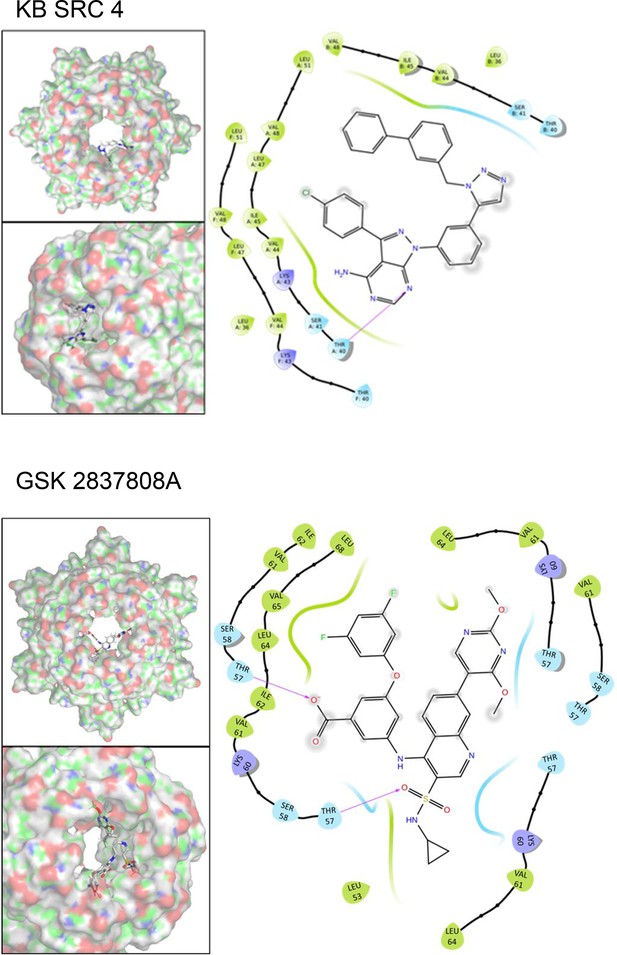
Predicted binding poses and residue interactions for lumenally targeted hit compounds.
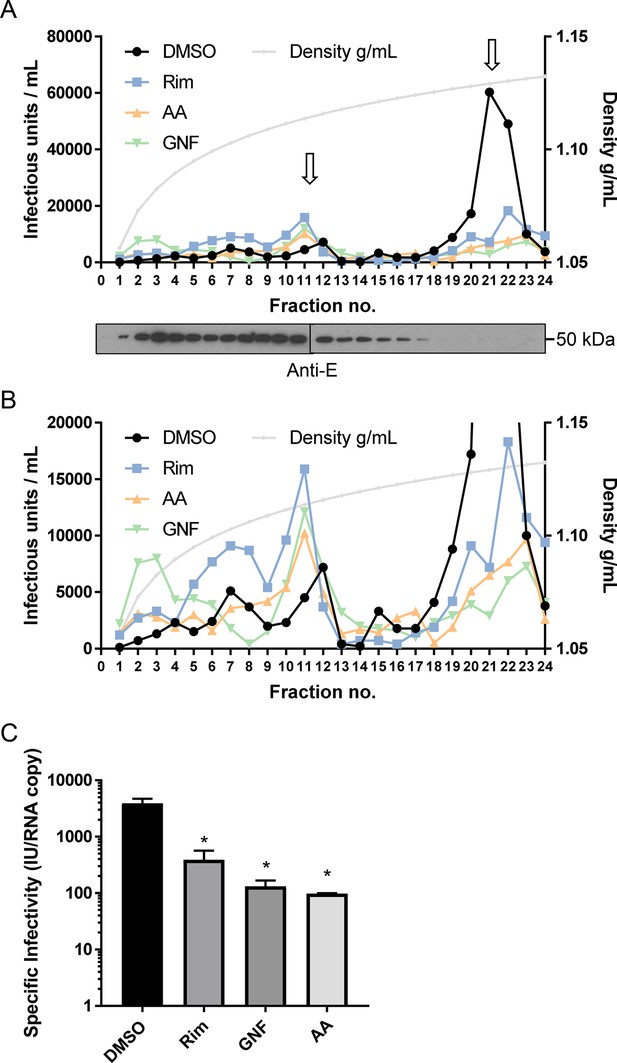
M channel inhibitors lack virus-lytic or cell-targeted effects.
To exclude the possibility that early effects upon ZIKV infection were due to a directly damaging effect of compounds upon virion integrity, particles were concentrated and purified from Vero cell supernatants by PEG-precipitation and ultracentrifugation through a sucrose cushion. Virions were then dosed with inhibitors prior to ultracentrifugation through a continuous iodixinol gradient. This not only purifies virions according to buoyant density, but separates them from small molecules, precluding off-target effects upon cells during ensuing infection (see methods for details). (A) Infectivity profile from a representative gradient experiment where purified virions were treated with rimantadine (40μM), GNF 5837 (1 μM), or AA 29504 (10 μM) prior to ultracentrifugation (or, DMSO at equivalent concentrations). 10μl from each fraction was then used to infect naive Vero cells in a 96-well plate, representing a 10-fold dilution. Infected cells were detected by immunofluorescence and counted as described elsewhere. A further 15μl was analysed by western blotting for the (E)nvglycoprotein (lower panel). (B) Zoomed in view of gradient in A, with Y-axis capped at 20,000 units to delineate infectivity profiles. (C) Specific infectivity of virions in the presence/absence of inhibitors based upon q-RT-PCR normalisation confirms the reduction of infectivity rather than disrupted virions. p≤0.05, Student's t-test. Error bars represent standard deviations based upon n=3 biological replicates.
-
Figure 7—source data 1
Infectivity data and fraction density (normalised) for iodixinol gradients with untreated or inhibitor treated purified ZIKV particles, titred on Vero cells by fluorescence focus forming assay.
Calculation of specific infectivity within ‘peak fractions’ normalised to RNA content, determined by RT-qPCR.
- https://cdn.elifesciences.org/articles/68404/elife-68404-fig7-data1-v2.zip
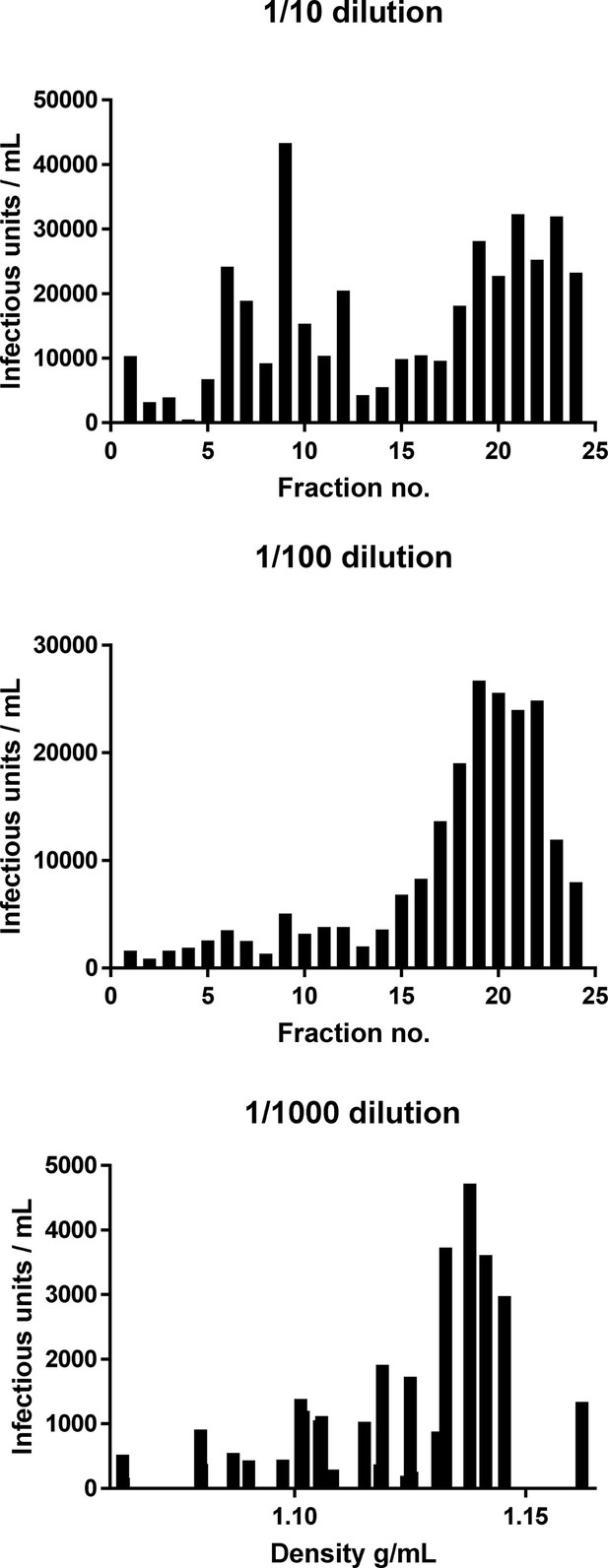
Effect of dilution upon measured titre for ZIKV purified through iodixinol gradients, as described in Figure 7.
Identical gradients were titred upon Vero cells across a 10-fold dilution series. More concentrated innoculae (1:10) resulted in a detectable lower-density peak, whereas further dilution (1:100, 1:1000) led to this diminishing whilst the higher-density peak infectivity remained. Investigations into the composition of the low-density peak are ongoing.
-
Figure 7—figure supplement 1—source data 1
Prism file with infectivity data for serially diluted gradient fractions containing purified ZIKV particles, titred on Vero cells.
- https://cdn.elifesciences.org/articles/68404/elife-68404-fig7-figsupp1-data1-v2.zip
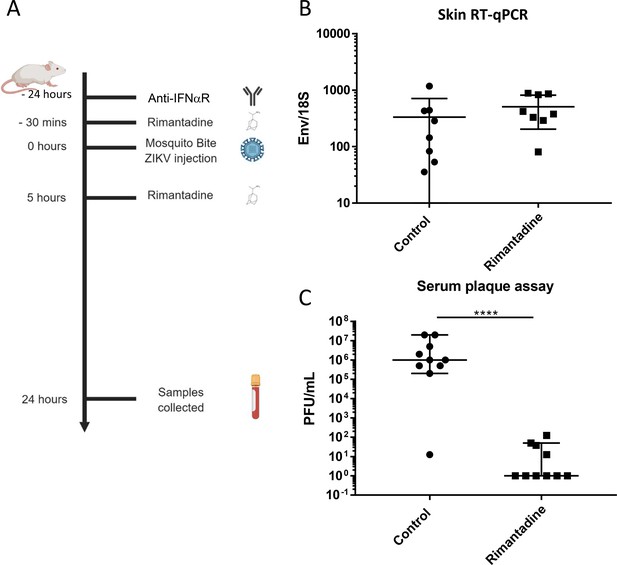
Anti-ZIKV activity in preclinical models.
(A) Schematic of preclinical experiments involving transient IFNAR blockade, augmentation of ZIKV infection through mosquito bites and treatment with rimantadine. (B) RT-qPCR for ZIKV E relative to 18S RNA from skin tissue at injection site derived from one of two representative experiments. (C) Infectious titre of serum derived from rimantadine treated or control mice, determined by plaque assay in BHK21 cells. ****p ≤ 0.0001, Student’s t-test.
© 2024, BioRender Inc. Panel A was created using BioRender, and is published under a CC BY-NC-ND license. Further reproductions must adhere to the terms of this license.
-
Figure 8—source data 1
Infectivity and RT-qPCR data for serum and skin, respectively, for mice infected with ZIKV alongside transient blockade of IFNAR1 and mosquito bites, with or without rimantadine treatment as per schematic.
- https://cdn.elifesciences.org/articles/68404/elife-68404-fig8-data1-v2.zip
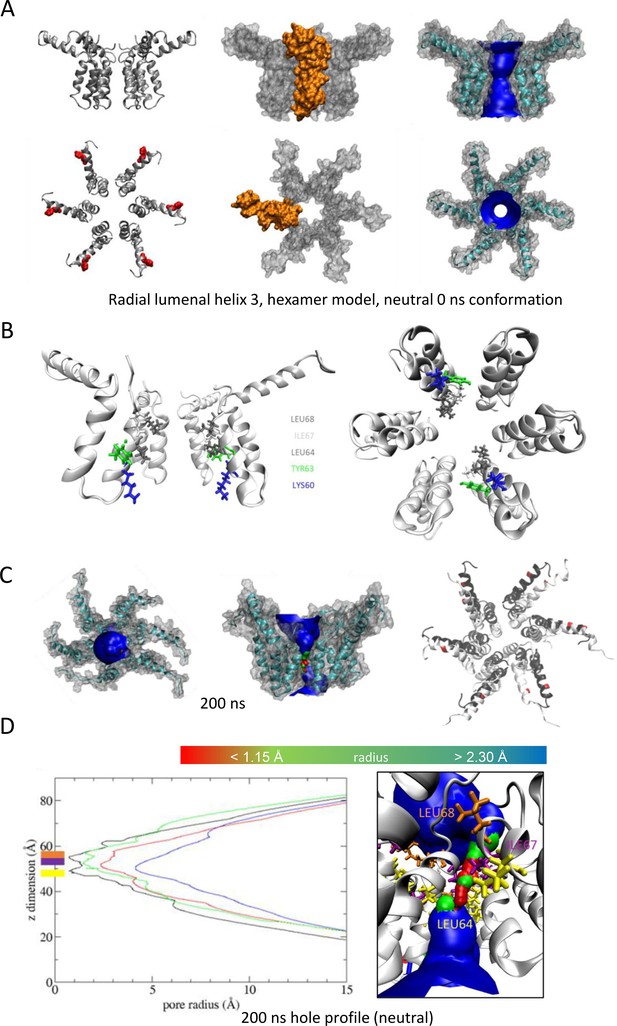
'Radial’ hexameric channel model with the lumen lined by helix 3.
(A) Side (top) and plan (bottom) views of channel complexes as ribbon, space-fill (with protomer highlighted in gold), and ribbon/space-fill with HOLE lumenal profile. Structures energy minimised at neutral pH prior to simulation (i.e. 0 ns). His28 highlighted in red. (B) Cut-away (left) and top view of complex illustrating residues lining the lumen prior to simulation. (C) Examples of structures at 200 ns from four atomistic simulations, showing plan, side/cut-away, and top-ribbon views. (D) Hole profiles of the lumen following four separate atomistic simulations, with illustration of lumenal constriction.
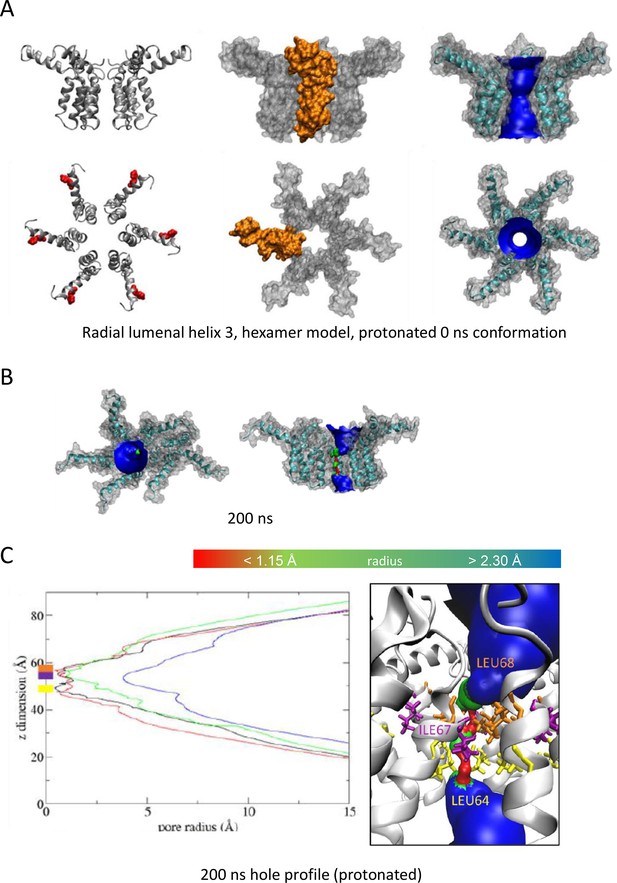
As Figure 1, modelling acidic environment using dual protonation of the His28 residue.
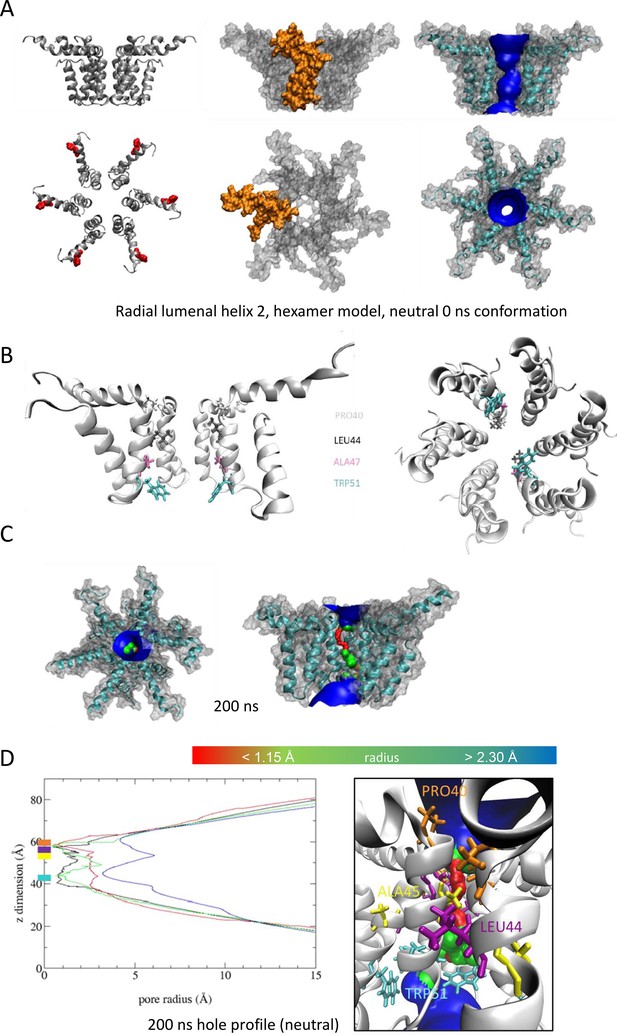
'Radial’ hexameric channel model with the lumen lined by helix 2.
(A–D) as per Figure 1.
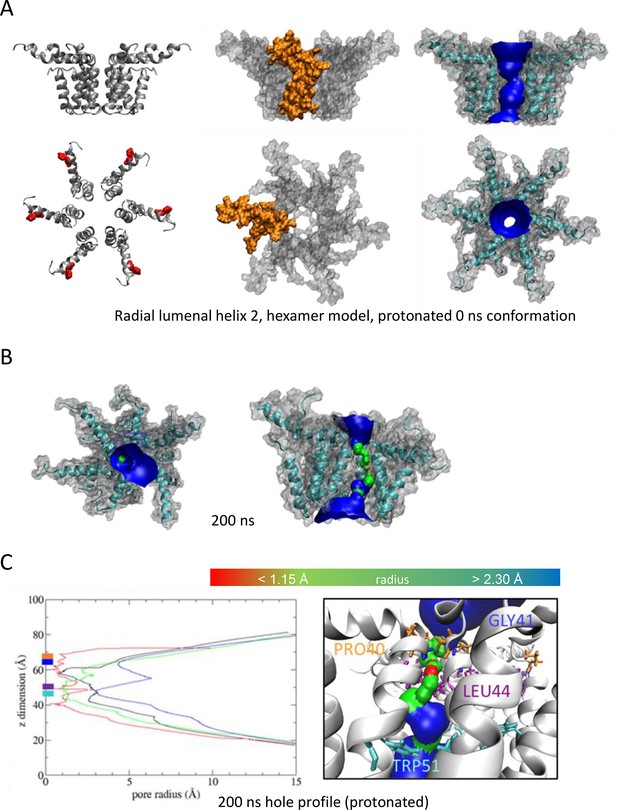
As Figure 3, modelling acidic environment using dual protonation of the His28 residue.
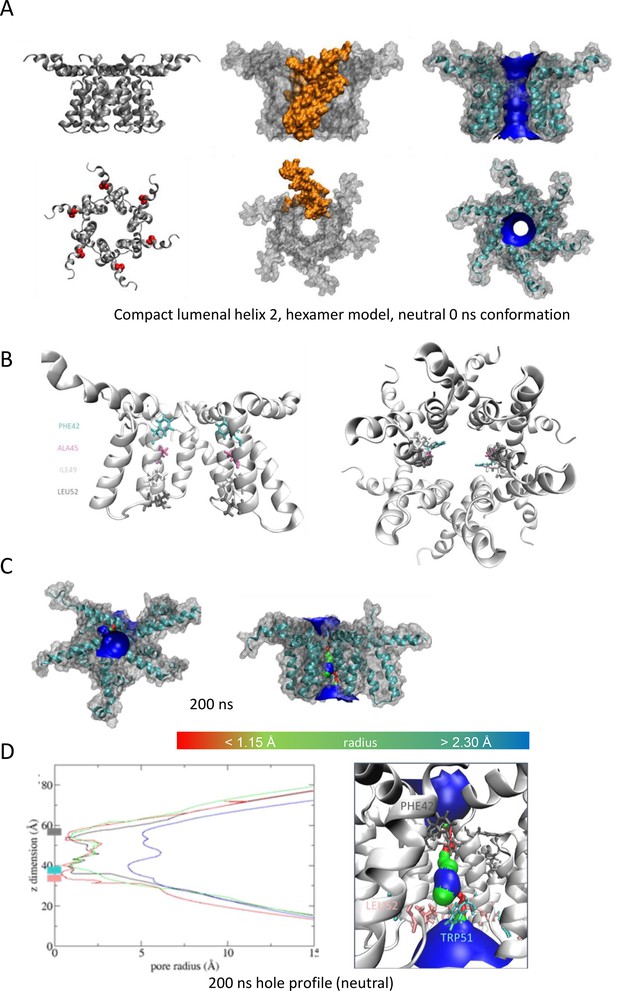
'Compact’ hexameric channel model with the lumen lined by helix 2.
(A–D) as per Figure 1.
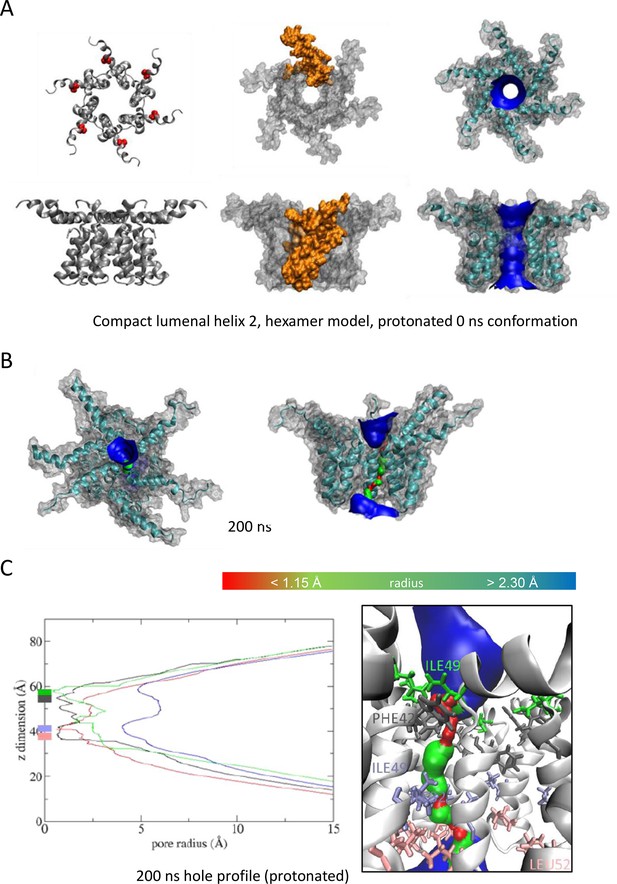
As Figure 5, modelling acidic environment using dual protonation of the His28 residue.
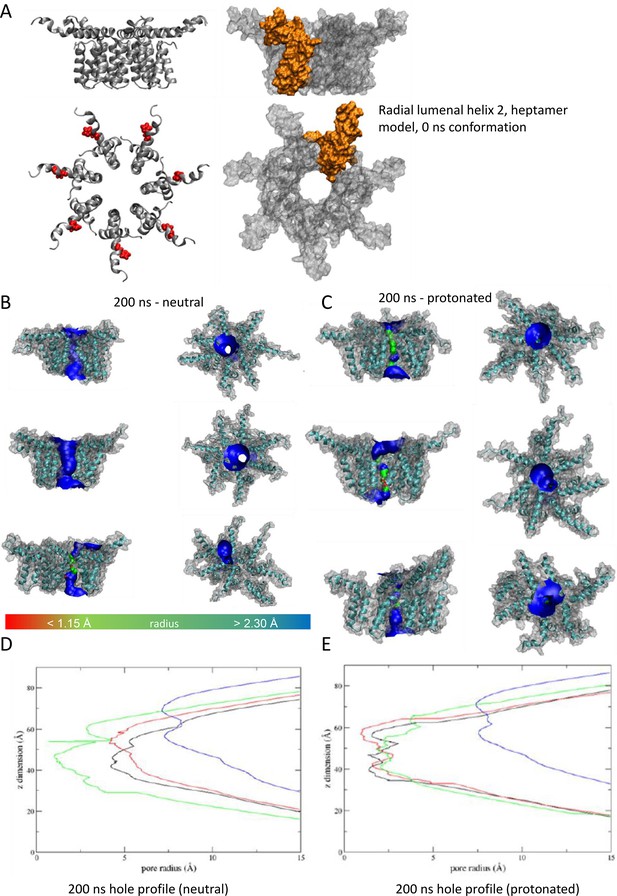
'Radial’ heptameric channel model with the lumen lined by helix 2.
(A) Side (top) and plan (bottom) views of channel complexes as ribbon or space-fill (with protomer highlighted in gold). (B) Example conformers following 200 ns simulation at neutral pH, or (C) with double-protonated His28. (D, E) HOLE programme lumenal profiles corresponding to B and C.
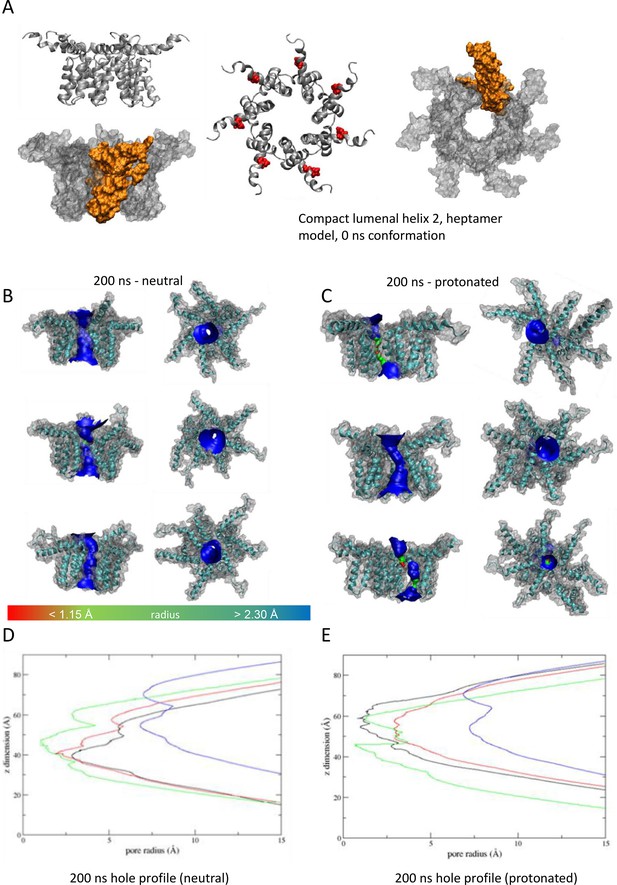
'Compact’ heptameric channel model with the lumen lined by helix 2.
(A–E) as per Figure 7.
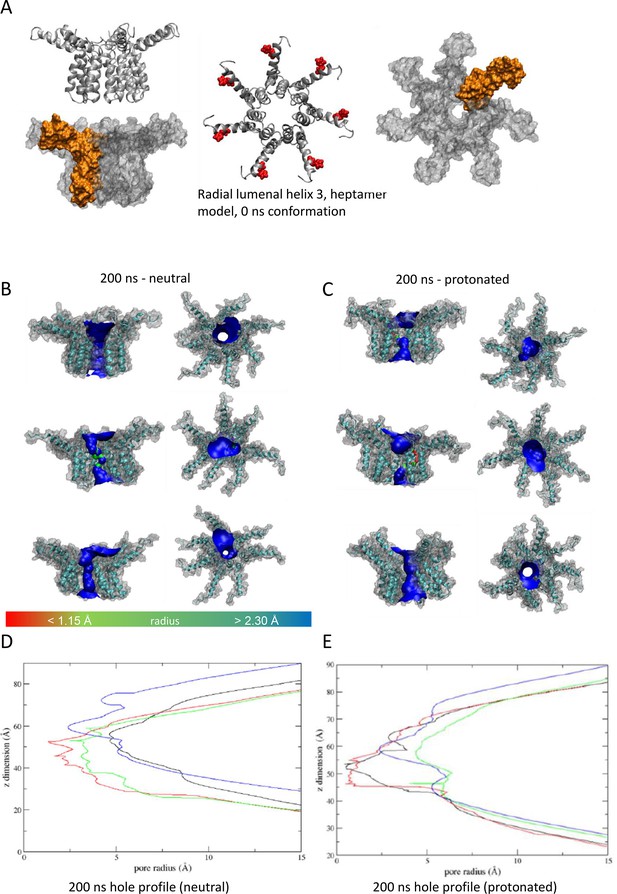
'Radial’ heptameric channel model with the lumen lined by helix 3.
(A–E) as per Figure 7.
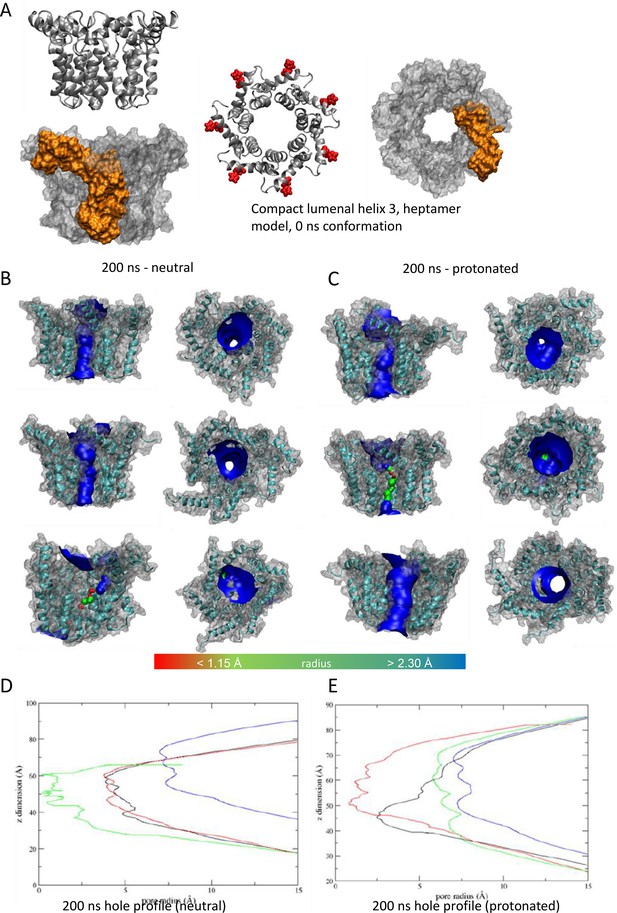
'Compact’ heptameric channel model with the lumen lined by helix 3.
(A–E) as per Figure 7.
Tables
Top 50 lumenally targeted compounds from the TOCRIS screen library, ranked by glide score.
‘Rank’ | Compound name | Tocris ID | Glide gscore |
---|---|---|---|
1 | FR 139317 | 1210 | −10.227 |
2 | ZCL 278 | 4794 | −9.294 |
3 | Elinogrel | 5316 | −8.829 |
4 | Taxol | 1097 | −8.711 |
5 | UK 356618 | 4187 | −8.401 |
7 | TC-1 15 | 4527 | −8.216 |
8 | AS 2034178 | 5035 | −8.032 |
9 | CP 775146 | 4190 | −7.963 |
10 | GW 6471 | 4618 | −9.092 |
11 | Pravastatin sodium salt | 2318 | −7.825 |
12 | Fluvastatin sodium | 3309 | −7.717 |
13 | TC NTR1 17 | 5087 | −7.683 |
14 | VER 155008 | 3803 | −7.686 |
15 | AMG PERK 44 | 5517 | −7.618 |
16 | Glibenclamide | 0911 | −7.537 |
17 | Argatroban | 1637 | −7.454 |
18 | KB SRC 4 | 4660 | −7.444 |
19 | GBR 12909 dihydrochloride | 0421 | −7.965 |
20 | (±)-NBI 74330 | 4528 | −7.366 |
21 | CU CPT 4a | 4883 | −7.331 |
22 | A 887826 | 4249 | −7.315 |
23 | SR 2640 hydrochloride | 1804 | −7.296 |
24 | NSC 74859 | 4655 | −7.258 |
25 | RWJ 67657 | 2999 | −7.217 |
26 | Lu AA 47070 | 4783 | −7.659 |
27 | Edaglitazone | 4784 | −7.176 |
29 | GSK 1562590 hydrochloride | 5110 | −7.11 |
30 | Flurizan | 4495 | −7.098 |
31 | GW 9508 | 2649 | −7.096 |
32 | GSK 269962 | 4009 | −7.051 |
33 | AC 5216 | 5281 | −6.986 |
34 | DBZ | 4489 | −6.973 |
35 | PF 04418948 | 4818 | −6.957 |
36 | GSK 2837808A | 5189 | −6.942 |
37 | Sal 003 | 3657 | −6.935 |
38 | PD 173212 | 3552 | −7.029 |
39 | NTRC 824 | 5438 | −9.012 |
40 | ONO AE3 208 | 3565 | −6.889 |
41 | RS 17053 hydrochloride | 0985 | −6.88 |
42 | Pitavastatin calcium | 4942 | −6.864 |
43 | L-161,982 | 2514 | −6.858 |
44 | AMN 082 dihydrochloride | 2385 | −6.871 |
45 | TC-N 1752 | 4435 | −6.815 |
46 | PF 431396 | 4278 | −6.799 |
47 | GNF 5837 | 4559 | −6.754 |
48 | KS 176 | 4169 | −6.731 |
49 | Sarpogrelate hydrochloride | 3739 | −6.769 |
50 | GKA 50 | 5133 | −6.689 |
N1 | Mifepristone | 1479 | 0.286 |
Top 50 peripherally targeted compounds from the TOCRIS screen library, ranked by glide score.
‘Rank’ | Compound | Cat No | Glide gscore |
---|---|---|---|
1 | RWJ 21757 | 2719 | −6.475 |
2 | Ferrostatin 1 | 5180 | −6.309 |
3 | AA 29504 | 3972 | −6.31 |
4 | L-732,138 | 0868 | −6.167 |
5 | API-2 | 2151 | −6.158 |
6 | 5-BDBD | 3579 | −6.13 |
7 | LY 225910 | 1018 | −6.082 |
8 | Formoterol hemifumarate | 1448 | −6.065 |
9 | TC-S 7006 | 5240 | −5.979 |
10 | TCS 2210 | 3877 | −6.005 |
11 | Sumatriptan succinate | 3586 | −5.952 |
12 | MRS 3777 hemioxalate | 2403 | −6.964 |
13 | Thiamet G | 4390 | −6.079 |
14 | Abacavir hemisulfate | 4148 | −5.894 |
15 | DDR1-IN-1 | 5077 | −5.99 |
16 | Fexofenadine hydrochloride | 2429 | −5.859 |
17 | 6-Chloromelatonin | 0443 | −5.844 |
18 | GSK 0660 | 3433 | −5.864 |
19 | PCA 4248 | 0571 | −5.783 |
20 | Axtinib | 4350 | −5.765 |
21 | DSR 6434 | 4809 | −5.971 |
22 | Necrostatin-1 | 2324 | −5.756 |
23 | Trifluorothymidine | 4460 | −5.838 |
24 | Cilndipine | 2629 | −5.729 |
25 | Efondipine hydrochloride monoethanolate | 3733 | −5.693 |
26 | ITE | 1803 | −5.69 |
27 | L-165,041 | 1856 | −5.689 |
28 | EB 47 | 4140 | −6.033 |
29 | GSK 2830371 | 5140 | −5.657 |
30 | AZD 1480 | 5617 | −5.738 |
32 | Amlodipine besylate | 2571 | −5.638 |
33 | Melatonin | 3550 | −5.628 |
34 | Fludarabine | 3495 | −5.626 |
35 | PF 06447475 | 5716 | −5.609 |
36 | SU 6668 | 3335 | −5.608 |
37 | AZD 5438 | 3968 | −5.836 |
38 | SU 11274 | 4101 | −6.145 |
39 | FPL 64176 | 1403 | −5.543 |
40 | Sunitinib malate | 3768 | −5.543 |
41 | YK 4-279 | 4067 | −5.535 |
42 | Ralfinamide mesylate | 4029 | −5.678 |
43 | ML 298 hydrochloride | 4895 | −5.504 |
44 | FH 1 | 5254 | −5.485 |
45 | PLX 647 dihydrochloride | 5102 | −6.23 |
46 | GPi 688 | 3967 | −5.438 |
47 | CP 94253 hydrochloride | 1317 | −5.441 |
48 | CGP 57380 | 2731 | −5.44 |
49 | BW 723C86 hydrochloride | 1059 | −5.433 |
50 | LY 364947 | 2718 | −5.84 |
Properties of hits taken forward into ZIKV cell culture screens, including effects versus canonical targets.
Drug | M-binding site (EC/IC50) | Canonical target (Ki/EC/IC50) | MTT effect? |
---|---|---|---|
Rimantadine | Lumen (80μM) | IAV M2 (variable) | No |
GNF 5837 | Lumen (~80nM) | Tropomyosin receptor kinases (TrK) A, B, and C (10nM) | No |
KB SRC 4 | Lumen (~250nM) | c-Src (Ki = 44nM) | Yes, 500nM |
GSK 2837808A | Lumen (~40μM) | Lactate dehydrogenase A and B (LDHA/B), 2.6/43nM | No |
Formoterol | Periphery (~80μM) | β2-Adrenergic receptor agonist (pM range) | Yes, 100μM |
L-732, 183 | Periphery (~40μM) | Tachykinin NK1 receptor antagonist (IC50 = 2.3nM) | No |
AA 29504 | Periphery (~10μM) | GABAA receptor agonist (IC50 = 9–13μM) | Marginal, 20μM |
Summary of course-grain simulations.
CG simulation | Membrane composition | Duration (µs) |
---|---|---|
1 TMD POPC | POPC (100) | 5 × 3 |
2 TMD POPC | POPC (100) | 5 × 3 |
Summary of all-atom simulations.
AA simulation | Membrane composition | Duration (ns) |
---|---|---|
Hexamer (lumenal helix; compact vs radial; pH) | ||
H2 Radial | POPC (100) | 2 × 200 |
H2 Radial protonated | POPC (100) | 2 × 200 |
H2 Compact | POPC (100) | 2 × 200 |
H2 Compact protonated | POPC (100) | 2 × 200 |
H3 Radial | POPC (100) | 3 × 200 |
H3 Radial protonated | POPC (100) | 3 × 200 |
H3 Compact | POPC (100) | 3 × 200 |
H3 Compact protonated | POPC (100) | 3 × 200 |
Heptamer (lumenal helix; compact vs radial; pH) | ||
H2 Radial | POPC (100) | 2 × 200 |
H2 Radial protonated | POPC (100) | 2 × 200 |
H2 Compact | POPC (100) | 2 × 200 |
H2 Compact protonated | POPC (100) | 2 × 200 |
H3 Radial | POPC (100) | 2 × 200 |
H3 Radial protonated | POPC (100) | 2 × 200 |
H3 Compact | POPC (100) | 2 × 200 |
H3 Compact protonated | POPC (100) | 2 × 200 |