Evolution of pathogen tolerance and emerging infections: A missing experimental paradigm
Figures
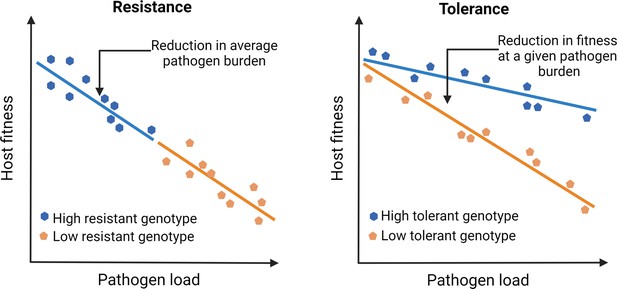
Outlining the difference between resistance vs. tolerance.
Defense mechanisms against invading pathogens can either include eliciting immune responses to detect and eliminate pathogens (resistance) or mitigate the fitness costs of infection or immune activation without directly reducing the pathogen load (tolerance). Different genotypes are initially exposed to the same number of pathogens. Figure plotted based on hypothetical data and adapted from Figure 1 of Råberg et al., 2007.
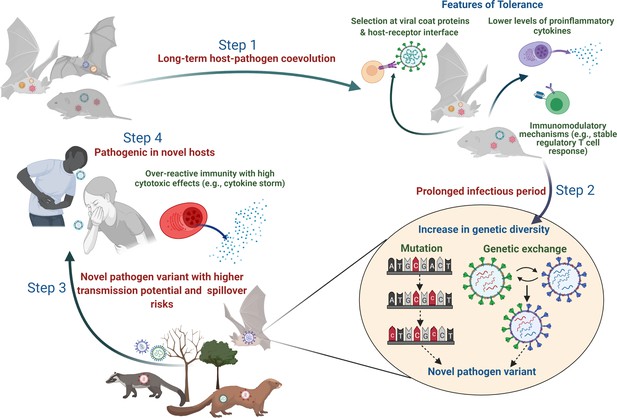
Plausible sequence of events leading to the emergence of zoonotic diseases from tolerant reservoir hosts.
Step 1: Long-term coevolution with natural pathogens might lead to specific adaptation of the immune system of reservoir species such that they can tolerate pathogens by reducing their counteractive inflammatory responses or evolving various immunomodulatory responses (e.g., altered activity of regulatory T-cells; Pavlovich et al., 2018; Robertson and Hasenkrug, 2006). Step 2: Tolerant hosts with reduced inflammatory responses can support the circulation of diverse pathogen species and strains. A longer infectious period within tolerant hosts also provides an accurate ecological niche where pathogens can acquire newer mutations over time or undergo genetic exchanges and re-assortments to produce novel variants (Bhat et al., 2021; Domingo-Calap, 2019). Step 3: Although the risk of infecting a new host species (other than the natural reservoir species) might be low, some of these pathogen variants with altered genetic backgrounds might be able to cross the species barrier and infect a new host more effectively (Mandl et al., 2015). Step 4: When novel hosts (e.g., humans or domestic animals) come into contact with these pathogens, they might face severe illness as they are unable to tolerate the impacts of infection or lack mechanisms to reduce the cytotoxic effects of inflammatory responses (e.g., cytokine storm during SARS-CoV-2; Azkur et al., 2020).
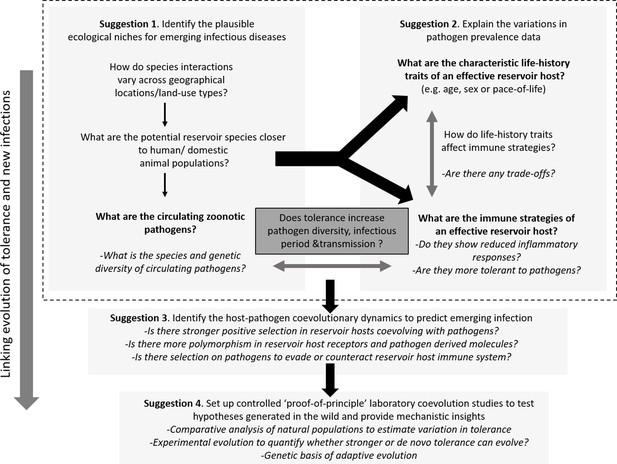
Outline of the proposed experimental paradigm, linking host ecology, tolerance and immune responses, and life-history to understand the natural contexts of emerging infections.
While performing the traditional disease surveillance program, information on ecology, life-history, and immune strategies of potential reservoirs can be gathered in parallel to explain why and how zoonotic pathogens are distributed in the wild (Suggestions 1 and 2). Once potential reservoir hosts and zoonotic pathogens are identified, their associations can be tested for signatures of coevolution and compared with overlapping populations of novel hosts (e.g., humans or domestic animals) to analyze the observed polymorphisms of key molecules involved in reservoir host-zoonotic pathogen interactions, divergence in infection outcomes, or immune evasion strategies of pathogens (Suggestion 3). Finally, controlled laboratory experiments can be performed to test causal links between host-pathogen coevolution, pathogen tolerance, and prevalence (Suggestion 4).
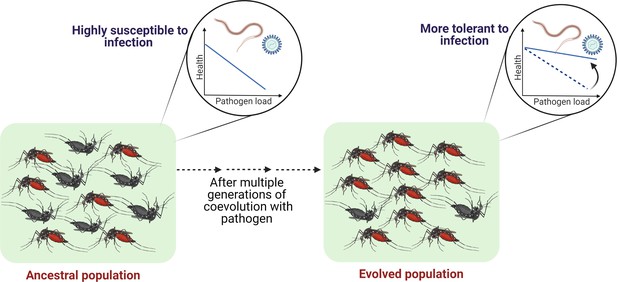
Outlining the controlled laboratory experimental evolution framework to test the link between coevolutionary history and tolerance.
Susceptible host populations can be exposed to the coevolving pathogens at every generation and assayed periodically to estimate the changes in the tolerance level.
Tables
Plausible effects of different life-history traits on immune responses and tolerance.
Although resource availability and nutrition are not life-history traits, we listed them as they impact body condition and fitness (Huang et al., 2013). ‘↑’ denotes increase in tolerance while ‘↓’ denotes decrease in tolerance.
Traits | Plausible effects on infection and immunity | Reference | Predicted role in tolerance |
---|---|---|---|
Reproduction | Higher investment in reproductive output trades-off with immune responses | Short et al., 2012 | Higher reproduction↑ |
Mating effort | Increased mating activity leads to immune suppression | Rolff and Siva-Jothy, 2002 | Increased mating effort↑ |
Pace of life | Fast pace of life reduces investment in immunity and allocate more resources to early-sexual maturity and -reproductive output | Previtali et al., 2012 | Animals with fast pace of life↑ |
Sex | Option 1: Males invest less in immunity due to high intra-sexual competition for females and variation in reproductive success | Bateman, 1948; Zuk and Stoehr, 2002 | Males↑* |
Option 2: Males disperse more and hence, are exposed to increasing number of (diverse) pathogens | Streicker et al., 2016 | Males↑ | |
Option 3: Organ-specific localization and impacts of pathogens across sexes; e.g., pathogens that could infect and colonize only ovary might show female-specific pathology | Brandt and Schneider, 2007 | Males↑ | |
Age | Younger individuals maximize their reproduction by minimizing investment in immune activation and pathogen clearance, thereby avoiding fitness-reducing immunopathological consequences | Khan et al., 2017a; Medzhitov et al., 2012 | Younger individuals↑ |
Starvation/ availability of resource | Option 1: Organisms invest less in immune defenses when deprived of resources | Stucki et al., 2019 | Starving individuals↑ |
Option 2: Well-fed individuals can withstand the effects of pathogens without clearing them because of their better body condition | Knutie et al., 2016 | Well-fed individuals↑ | |
Nutrition | Option 1: Access to a proteinaceous diet might boost the immune system and pathogen clearance ability | Povey et al., 2009 | Higher protein content↓ |
Option 2: Access to a proteinaceous diet might allow hosts to compensate for the costs of harboring pathogens | Knutie et al., 2017 | Higher protein content↑ |
-
*
Also see contrasting examples in Jarefors et al., 2006 or Cousineau and Alizon, 2014; females invest more in anti-inflammatory responses which might increase their tolerance.