Translational control of polyamine metabolism by CNBP is required for Drosophila locomotor function
Figures
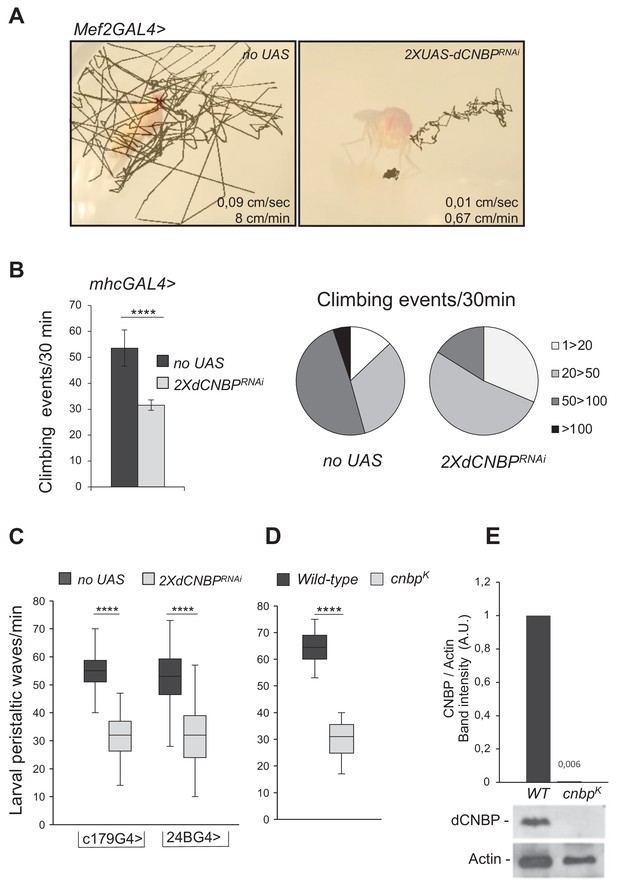
Specific dCNBP depletion in muscle tissues results in locomotor defects.
(A) Locomotion activity in escapers adult flies expressing (B) UAS-dCNBPRNAi-16283; UAS-dCNBPRNAi-16284 (2XdCNBPRNAi) driven by the myocyte enhancer factor 2 (Mef2)-GAL4 at 25°C, compared to control flies (no UAS). Fly movements were recorded (Figure 1—video 1, 2, 3) and then analyzed by the animal tracker ImageJ plugin, to quantify both fly speed (average, cm/s) and distance covered in 1 min (Gulyás et al., 2016). (C) Climbing activity defects in adult flies expressing 2XdCNBPRNAi driven by the mhc-GAL4 at 29°C. The locomotion activity was measured by the Drosophila activity monitoring (DAM) system, as the number of climbing events in 30 min ≥ 80 males tested for each genotype. On the left, climbing performance of control flies (no UAS) or dCNBP-depleted flies (2XdCNBPRNAi) 7 days after eclosion represented as the average of climbing events (CEs) in 30 min (error bars represent SEM; ****p<0.0001, Mann-Whitney-Wilcoxon test). On the right, quantitative grouping of climbing performances in four different classes. Classes 1–20 (white area) and 20–50 (light gray area) CEs are highly represented in RNAi flies (2XdCNBPRNAi), while classes 50–100 CEs (dark gray area) are more frequent in control flies (no UAS). Only control flies have the ability to perform more than 100 CEs in 30 min (black area). Full data in Figure 1—source data 1. (D) Box plot representation of the distribution of peristaltic contraction rates performed in 1 min by control (no UAS) or UAS-dCNBPRNAi-16283; UAS-dCNBPRNAi-16284 (2XdCNBPRNAi) third instar larvae under the control of either c179GAL4 or 24BGAL4 driver at 25°C (****p<0.0001, t-test); ≥10 larvae tested for each genotype in at least three independent experiments. Full data in Figure 1—source data 1. (E) Box plot representation of the distribution of peristaltic contraction rates performed by control (wild-type [WT]) or cnbpk mutant second instar larvae in 1 min; ≥30 larvae tested for each genotype (p < 0.0001, Mann-Whitney-Wilcoxon test). In (C and D) the line inside the box indicates the median for each genotype and box boundaries represent the first and third quartiles; whiskers are min and max in the 1.5 interquartile range. Full data in Figure 1—source data 1. (F) Immunoblot showing the levels of dCNBP in extract obtained from cnbpk mutant second instar or from WT control larvae with the corresponding band quantification normalized on the loading control (IMAGE J 1.50i; quantification data in source data for western blot [WB] quantification). Actin, loading control. A.U., arbitrary unit.
-
Figure 1—source data 1
Adult and larval movement measurements as shown in Figure 1B-D.
- https://cdn.elifesciences.org/articles/69269/elife-69269-fig1-data1-v1.xlsx
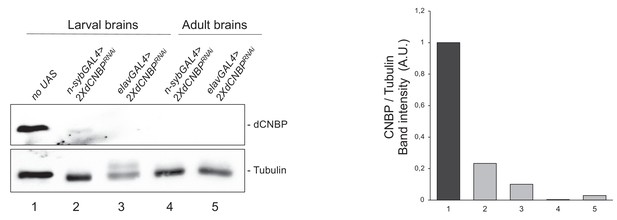
dCNBP knockdown efficacy in absence of phenotype.
Immunoblot showing the levels of dCNBP in extract obtained from larval brains or adult heads of UAS-dCNBPRNAi-16283; UAS-dCNBPRNAi-16284 (2XdCNBPRNAi) individuals, where the knockdown is driven by either the n-syb or the elav GAL4 drivers at 25°C, compared to control (no UAS). On the right a graph showing the corresponding band quantification, normalized on the loading control (IMAGE J 1.50i; quantification data in source data for western blot [WB] quantification). Tubulin, loading control. A.U., arbitrary unit.
Example of locomotor movement of a control adult fly (myocyte enhancer factor 2 [Mef2]-GAL4 > no UAS at 25°C).
Example of locomotor defects of a dCNBP-interfered adult fly (myocyte enhancer factor 2 [Mef2]-GAL4 > UAS-dCNBPRNAi-16283; UAS-dCNBPRNAi-16284 at 25°C).
Second example of locomotor defects of a dCNBP-interfered adult fly (myocyte enhancer factor 2 [Mef2]-GAL4>UAS-dCNBPRNAi-16283; UAS-dCNBPRNAi-16284at25°C).
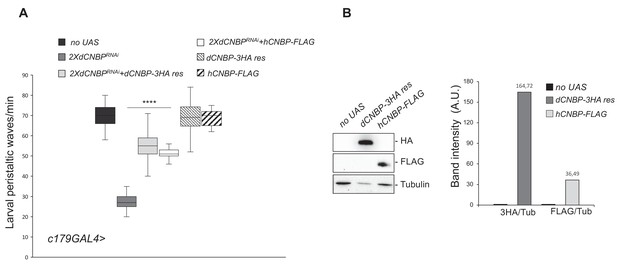
Cellular nucleic acid-binding protein (CNBP) overexpression rescues the locomotion phenotype induced by muscular dCNBP depletion.
dCNBP knockdown in embryonic mesoderm causes a significant reduction of larval peristaltic waves rescued by the expression of either dCNBP or hCNBP transgenes (25°C). (A) Box plot representation of the distribution of peristaltic contraction rates performed by third instar larvae of the following genotypes: only c179GAL4 driver (no UAS), c179GAL4>UAS-dCNBPRNAi-16283; UAS-dCNBPRNAi-16284 (2XdCNBPRNAi), c179GAL4>2XdCNBPRNAi + UAS-dCNBP-3HA-res (a dCNBP-3HA transgene resistant to 2XUASdCNBP-induced RNAi), c179GAL4>2XdCNBPRNAi + UAS-hCNBP-FLAG. The line inside the box indicates the median for each genotype and box boundaries represent the first and third quartiles; whiskers are min and max in the 1.5 interquartile range (****p<0.0001, Kruskal-Wallis with post hoc Dunn’s test); ≥10 larvae tested for each genotype in at least three independent experiments. Full data in Figure 2—source data 1. (B) The expression levels of both UAS-dCNBP-3HA-res and UAS-hCNBP-FLAG were analyzed by immunoblotting using antibodies against either the HA or the FLAG tag, compared to controls (no UAS). Bands were quantified by IMAGE J 1.50i and normalized on the loading control (quantification data in source data for western blot [WB] quantification). Tubulin, loading control. A.U., arbitrary unit.
-
Figure 2—source data 1
Larval movement measurements as shown in Figure 2A.
- https://cdn.elifesciences.org/articles/69269/elife-69269-fig2-data1-v1.xlsx
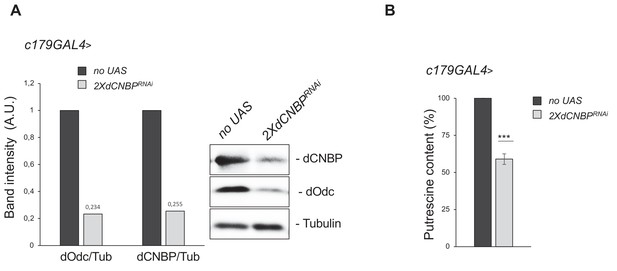
CNBP regulates the ornithine decarboxylase (ODC)/polyamine axis.
Levels of both Odc and putrescine are significantly reduced in dCNBP-depleted larvae compared to wild-type controls. (A) Immunoblot showing the levels of both dCNBP and dOdc in extract obtained from tubGAL4>2XdCNBPRNAi third instar larvae compared to control (no UAS), with the corresponding band quantification normalized on the loading control (IMAGE J 1.50i; quantification data in source data for western blot [WB] quantification). Actin, loading control. A.U., arbitrary unit. (B) Columns represent the fold difference of putrescine content in third instar larvae bearing the c179GAL4 driver alone (no UAS) or in combination with double copy dCNBP RNAi-expressing larvae (UAS-dCNBPRNAi-16283; UAS-dCNBPRNAi-16284, named 2XdCNBPRNAi). Error bars represent SEM; ***p>0.001, ** p>0.002, in unpaired t-test. A pool of 10 larvae has been tested for each genotype in three independent experiments. Full data in Figure 3—source data 1.
-
Figure 3—source data 1
Putrescine content quantification as shown in Figure 3B and Figure 3—figure supplement 1B.
- https://cdn.elifesciences.org/articles/69269/elife-69269-fig3-data1-v1.xlsx
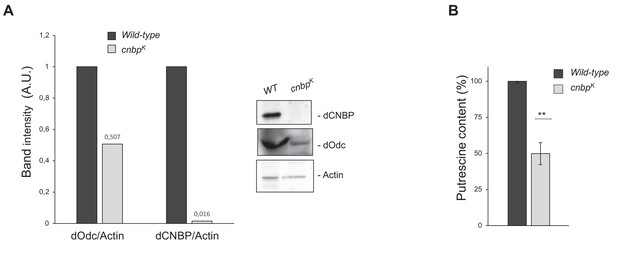
Larval locomotor defect observed in cnbpk mutants correlates with the reduction of ornithine decarboxylase (Odc) protein and polyamine levels.
Levels ofOdc protein and polyamine are significantly reduced in dCNBP mutant larvae compared to wild-type (WT) controls. (A) Immunoblot showing the levels of both dCNBP and dOdc in extract obtained from WT and cnbpk mutant second instar larvae, with the corresponding band quantification normalized on the loading control (IMAGE J 1.50i; quantification data in source data for western blot [WB] quantification). Actin, loading control. (B) Columns represent the fold difference of putrescine content in second instar larvae of cnbpk mutant compared to controls (WT). Error bars represent SEM; **p>0.002, t-test. A pool of 10 larvae has been tested for each genotype in three independent experiments. Full data in Figure 3—source data 1.
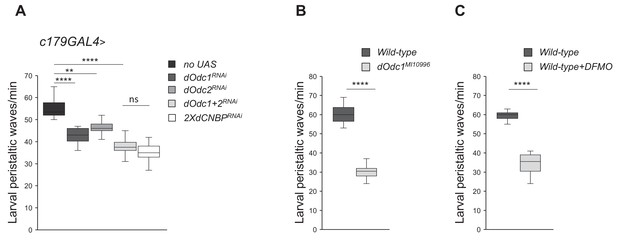
Ornithine decarboxylase (Odc) depletion phenocopies the dCNBP locomotor defects.
Box plot representation of the distribution of peristaltic contraction rates performed by third instar larvae of the reported genotypes in 1 min. (A) c179GAL4>no UAS, UAS-Odc1RNAi-30039, UAS-Odc2RNAi-10459, UAS-Odc2RNAi-10459; UAS-Odc1RNAi-30039, or UAS-dCNBPRNAi-16283; UAS-dCNBPRNAi-16284. In the graph legend UAS in transgenic RNAi lines is omitted for simplicity. (B) Controls (wild type) and dOdc1MI10996 mutant larvae. (C) Controls fed with standard fly food (wild type) or after DFMO treatment (5 mM/day; wild type + DFMO). The line inside the box indicates the median for each genotype and box boundaries represent the first and third quartiles; whiskers are min and max in the 1.5 interquartile range (**p<0.001; ****p<0.0001; ns, not significant, Kruskal-Wallis with post hoc Dunn’s test for multiple comparison or Mann-Whitney-Wilcoxon test for); ≥10 larvae tested for each genotype in at least three independent experiments. All full data in Figure 4—source data 1.
-
Figure 4—source data 1
Larval movement measurements as shown in Figure 4A-C and putrescine content quantification as shown in Figure 4—figure supplement 1.
- https://cdn.elifesciences.org/articles/69269/elife-69269-fig4-data1-v1.xlsx
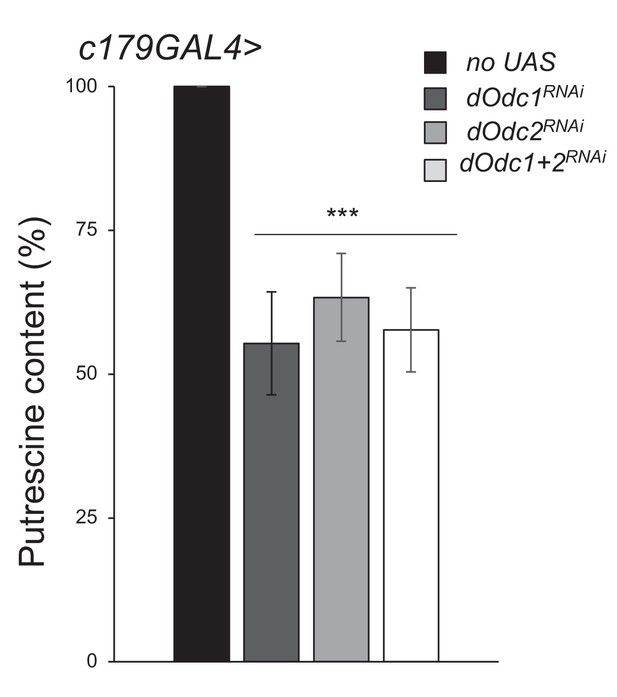
Larval locomotor defect observed as a consequence of ornithine decarboxylase (Odc) depletion correlates with the reduction of polyamine levels.
Columns represent the fold difference of putrescine content in third instar larvae of the following genotypes: only c179GAL4 driver (no UAS), c179GAL4>UAS-dOdc1RNAi-30039 (dOdc1RNAi), c179GAL4>UAS-dOdc2RNAi-10459 (dOdc2RNAi), c179GAL4>UAS-dOdc2RNAi-10459; UAS-dOdc1RNAi-30039 (dOdc1+2RNAi) (error bars represent SEM; ***p>0.001 with respect to the no UAS control, t-test). A pool of 10 larvae has been tested for each genotype in at least three independent experiments. Full data in Figure 4—source data 1.
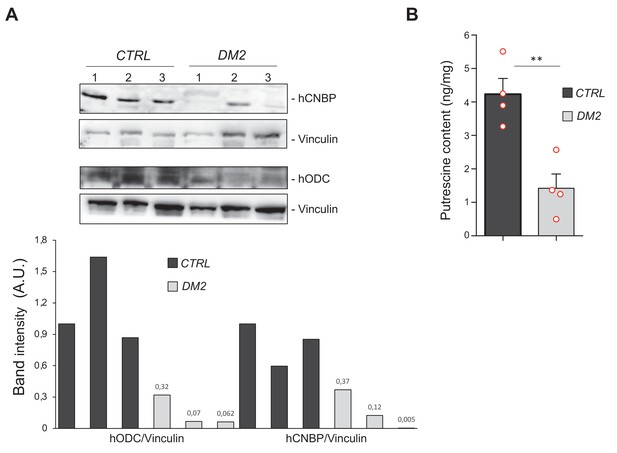
Polyamine metabolism is impaired also in myotonic dystrophy type 2 (DM2) muscles.
Cellular nucleic acid-binding protein (CNBP) and ornithine decarboxylase (ODC) content correlates with polyamine levels in muscle cells from DM2 patients. (A) Immunoblot showing the levels of both human CNBP (hCNBP) and human ODC (hODC) proteins in three DM2 or control muscle cells, with the corresponding band quantification normalized on the loading control (IMAGE J 1.50i; quantification data in source data for western blot [WB] quantification). Vinculin, loading control. A.U., arbitrary unit. (B) Columns represent putrescine content in muscle cells obtained from four DM2 patients (CTRL) or from four healthy individuals (DM2), expressed in ng/mg of tissue. Error bars represent SEM; **p>0.001, in unpaired t-test. Full data in Figure 5—source data 1.
-
Figure 5—source data 1
Putrescine content quantification as shown in Figure 5B.
- https://cdn.elifesciences.org/articles/69269/elife-69269-fig5-data1-v1.xlsx
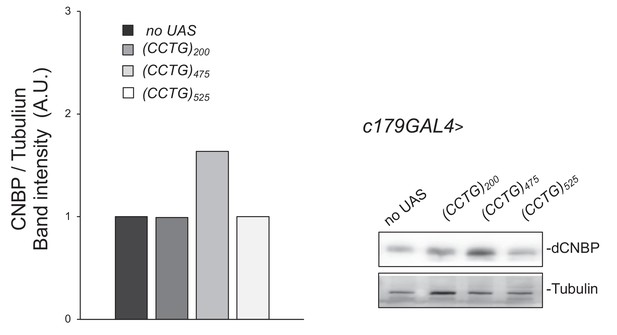
Expression levels of dCNBP are not affected by the expression of CCUG-expanded repeat RNA.
Immunoblot showing the levels of dCNBP in larval extract obtained from controls (no UAS) or UAS-(CCTG)200, UAS-(CCTG)475, UAS-(CCTG)525 driven by the c179GAL4 driver, with the corresponding band quantification normalized on the loading control (IMAGE J 1.50i; quantification data in source data for western blot [WB] quantification). Tubulin, loading control. A.U., arbitrary unit. A pool of 10 larvae has been tested for each genotype in at least two independent experiments.
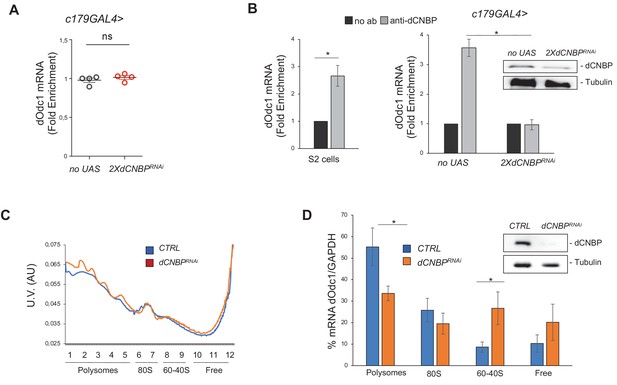
dCNBP controls polyamine metabolism through the binding and the translational control of dOdc mRNA.
(A) dOdc1 mRNA levels (qPCR), normalized with the housekeeping RPL11 mRNA third instar larvae bearing c179GAL4 driver alone (no UAS) or in combination with UAS-dCNBPRNAi-16283; UAS-dCNBPRNAi-16284 (2XdCNBPRNAi). ns, not significant in unpaired t-test. Dots correspond to four independent biological replicates; bars indicate the mean and SEM. (B) Cellular nucleic acid-binding protein (CNBP) binds dOdc1 mRNA. qRT-PCR analysis on mRNAs immunoprecipitated by anti-dCNBP antibody or control IgG antisera in S2 cells extracts (left graph), or in dCNBP-depleted (2XdCNBPRNAi) or not (no UAS) larval extracts (right graph). The results are indicated as fold difference, relative to IgG. Error bars represent SEM of three independent experiments; *p < 0.05, in t-test. The presence of dCNBP in c179GAL4>2XUASdCNBPRNAi or control (no UAS) larval carcasses was analyzed by western blotting (right). Tubulin, loading control. (C) Representative polysome profiles (of at least three independent experiments) of dCNBP-deficient (dCNBPRNAi) or control (CTRL) S2 cells. Cytoplasmic lysates were fractionated on 15–50% sucrose gradients. (D) qPCR analysis of dOdc1 mRNA loaded in the different polysome fractions, GADPH was used to normalize the values. (*p < 0.05, t-test. Error bars represent SEM of experiments performed in quadruplicates and repeated at least three times.) The presence of dCNBP in interfered or not interfered S2 cells was analyzed by western blotting (right). Tubulin, loading control. All full data in Figure 6—source data 1.
-
Figure 6—source data 1
Real-time qPCR data as shown in Figure 6A, B, D, in Figure 6—figure supplement 1A-B and in Figure 6—figure supplement 4B; polysome profile data as shown in Figure 6C.
- https://cdn.elifesciences.org/articles/69269/elife-69269-fig6-data1-v1.xlsx
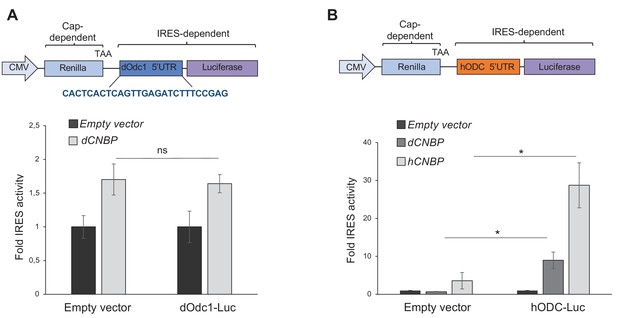
dCNBP does not control polyamine metabolism through dOdc1 internal ribosome entry site (IRES)-dependent translation.
(A) Schematic representation of the bicistronic CMV-renilla-TAA/dOdc1-IRES-firefly luciferase (dOdc1-luc) vector (top). The renilla ORF is translated via conventional cap-dependent mechanism, whereas translation of the luciferase ORF is controlled by the dOdc1 5’UTR sequence. IRES activity of dOdc1-luc which is not significantly modulated by dCNBP overexpression compared to the empty vector (bottom). Columns represent the fold changes of luciferase activity, normalized to the renilla expression. Error bars represent SEM; ns: no significant with Student’s t-test, of three independent experiments. (B) Schematic representation of a bicistronic CMV-renilla-TAA/hODC-IRES-firefly luciferase (hODC-luc) vector (top). IRES activity of hODC-luc which is significantly modulated by both dCNBP or hCNBP overexpression, compared to the empty vector (bottom). Columns represent the fold changes of luciferase activity, normalized to the renilla expression. Error bars represent SEM; p<0.05 with Student’s t-test, of three independent experiments. All full data in Figure 6—source data 1.
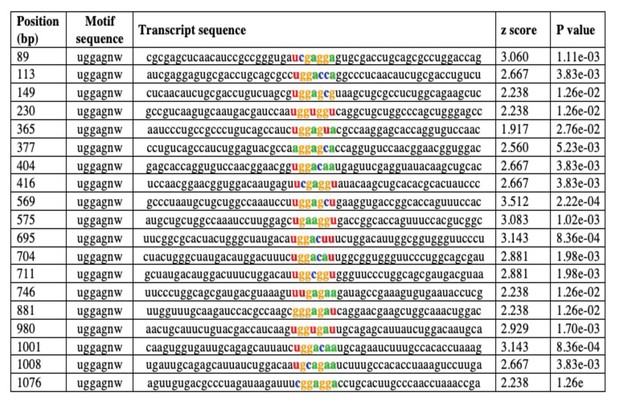
In silico prediction of putative cellular nucleic acid-binding protein (CNBP) binding sites on the dOdc1 mRNA by RBPmap.
The dOdc1 transcript (FBtr0088863) was uploaded to the RBPmap web server for mapping binding sites. As criteria Drosophila genome, UGGAGNW consensus motif and high stringency level were used (Paz et al., 2014; http://rbpmap.technion.ac.il).
-
Figure 6—figure supplement 2—source data 1
- https://cdn.elifesciences.org/articles/69269/elife-69269-fig6-figsupp2-data1-v1.docx
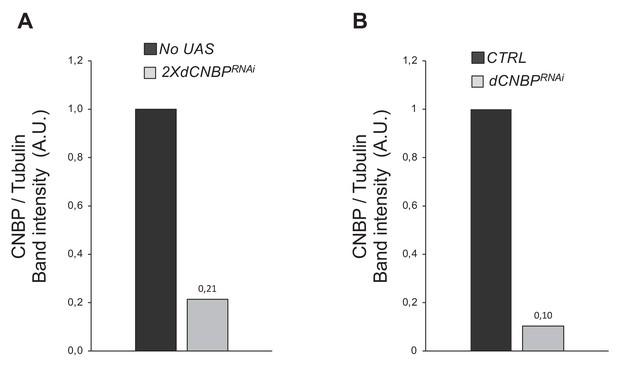
Efficiency of dCNBP silencing.
Quantification by IMAGE J 1.50i of the levels of dCNBP in extracts (A) obtained from control larvae (no UAS) or from larvae where the UAS-dCNBPRNAi-16283; UAS-dCNBPRNAi-16284 constructs (2XdCNBPRNAi) are driven by the c179GAL4 driver (from the immunoblot shown in Figure 6B); or in extracts (B) obtained from untreated S2 cells (CTRL) or from S2 cells CNBP-interfered (dCNBPRNAi; from the immunoblot shown in Figure 6D). A.U., arbitrary unit. Quantification data in source data for western blot [WB] quantification.
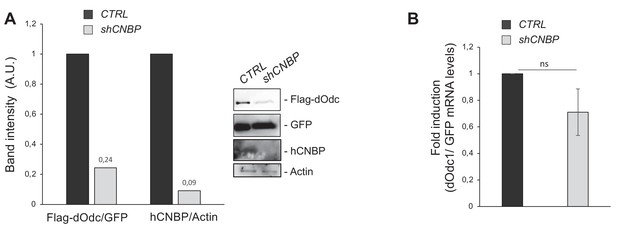
Cellular nucleic acid-binding protein (CNBP) promotes translation of dOdc mRNA.
Effect of human CNBP (hCNBP) depletion on both dOdc protein expression and mRNA level in 293T human cells. (A) Immunoblot showing a direct correlation of hCNBP depletion with a strong reduction of dOdc protein levels, with the corresponding band quantification normalized on the loading control (IMAGE J 1.50i; quantification data in source data for western blot [WB] quantification). Actin, loading control. A.U., arbitrary unit. (B) dOdc1 mRNA levels (qPCR), normalized with the GFP mRNA levels. Error bars represent SEM of three independent experiments; ns, not significant in unpaired t-test. Full data in Figure 6—source data 1.
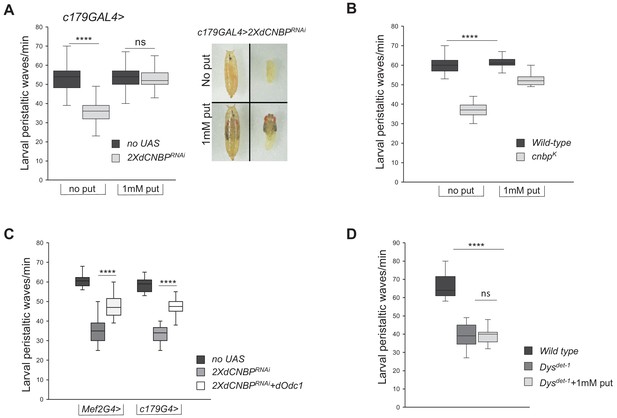
Odc and polyamine are responsible for the CNBP-dependent locomotor phenotype.
(A–B) Rescue of locomotor phenotype in both dCNBP-depleted larvae (A) and dCNBP mutant larvae (B) by 1 mM putrescine feeding at 29°C. Box plot representation of the distribution of peristaltic contraction rates performed by the following genotypes: (A) c179GAL4 driving no UAS or UAS-dCNBPRNAi-16283; UAS-dCNBPRNAi-16284 (2XdCNBPRNAi) with (1 mM put) or without (no put) putrescine. Note how putrescine feeding of interfered individuals results also in a higher stage of pupal development with respect to individuals not treated (photo in A). (B) Control (wild-type) or cnbpk larvae with (1 mM put) or without (no put) putrescine. (****p<0.0001; ns, not significant, Kruskal-Wallis with post hoc Dunn’s test). (C) Rescue of locomotor defects in dCNBP-depleted larvae by dOdc1 overexpression under the control of either Mef2 or c179GAL4 driver. Box plot representation of the distribution of peristaltic contraction rates performed by the following genotypes: Mef2GAL4 or c179GAL4 (no UAS), Mef2GAL4 or c179GAL4>2XdCNBPRNAi, Mef2GAL4 or c179GAL4>2XdCNBPRNAi + dOdc1 (UASdCNBPRNAi-16283; Mef2GAL4/UASdCNBPRNAi-16284; UASdOdc1/TM6B or UASdCNBPRNAi-16283; c179GAL4/UASdCNBPRNAi-16284; UAS dOdc1/+). (A–B–C) The line inside the box indicates the median for each genotype and box boundaries represent the first and third quartiles; whiskers are min and max in the 1.5 interquartile range (****p<0.0001; ns, not significant, Kruskal-Wallis with post hoc Dunn’s test); ≥10 larvae tested for each genotype in at least three independent experiments. (D) Mutants for dystrophin (Dysdet-1) present larval locomotor abnormalities that cannot be rescued by feeding larvae with 1 mM putrescine. Box plot representation of the distribution of peristaltic contraction rates performed by Dysdel-1 mutant larvae fed with or without putrescine (+1 mM put) with respect to wild-type control. The line inside the box indicates the median for each genotype and box boundaries represent the first and third quartiles; whiskers are min and max in the 1.5 interquartile range (ns, not significant, ****p<0.0001, Kruskal-Wallis with post hoc Dunn’s test); ≥10 larvae tested for each genotype in at least two independent experiments. All full data in Figure 7—source data 1.
-
Figure 7—source data 1
Larval and adult movement measurements as shown in Figure 7A-D, Figure 7—figure supplement 1B and Figure 7—figure supplement 3. Putrescine content measurment as shown in Figure 7—figure supplement 1A.
- https://cdn.elifesciences.org/articles/69269/elife-69269-fig7-data1-v1.xlsx
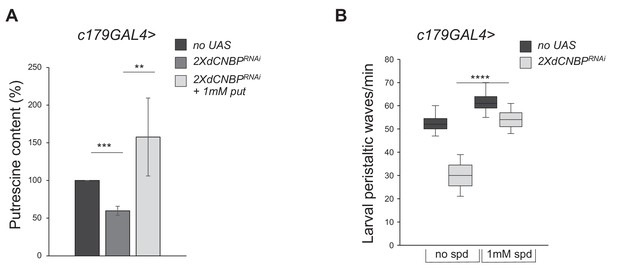
Effects of other polyamines on the cellular nucleic acid-binding protein (CNBP)-dependent locomotor phenotype.
(A) Columns represent the fold difference of putrescine content in dCNBP-depleted larvae grown in absence or presence of 1 mM putrescine. c179GAL4 without putrescine (no UAS), c179GAL4>UASdCNBPRNAi-16283; UASdCNBPRNAi-16284 (2XdCNBPRNAi) with (1 mM put) or without putrescine. **p<0.01, ***p<0.001 with t-test. (B) Box plot representation of the distribution of peristaltic contraction rates performed by third instar larvae of c179GAL4 (no UAS) or by c179GAL4>UASdCNBPRNAi-16283; UASdCNBPRNAi-16284 (2XdCNBPRNAi) with (1 mM spd) or without (no spd) spermidine. The line inside the box indicates the median for each genotype and box boundaries represent the first and third quartiles; whiskers are min and max in the 1.5 interquartile range (****p<0.0001, ordinary one-way ANOVA post hoc Tukey’s test); ≥10 larvae tested for each genotype in at least two independent experiments. Error bars represent SEM. All full data in Figure 7—source data 1.
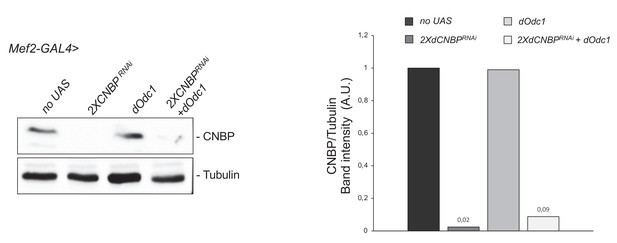
dOdc1 overexpression does not affect dCNBP downregulation.
Immunoblot showing the levels of dCNBP in control larvae (no UAS), compared to larvae interfered for dCNBP (UASdCNBPRNAi-16283; UASdCNBPRNAi-16284; named 2XdCNBPRNAi), or overexpressing dOdc1 (UAS-dOdc1), or to larvae overexpressing dOdc1 and simultaneously interfered for dCNBP (UASdCNBPRNAi-16283; Mef2-GAL4/UASdCNBPRNAi-16284; UAS Odc1/TM6B; named 2XdCNBPRNAi+dOdc1) under the control of the Mef2-GAL4 driver. On the right, the corresponding band quantification normalized on the loading control (IMAGE J 1.50i; quantification data in source data for western blot [WB] quantification) is shown. Tubulin, loading control. A.U., arbitrary unit.
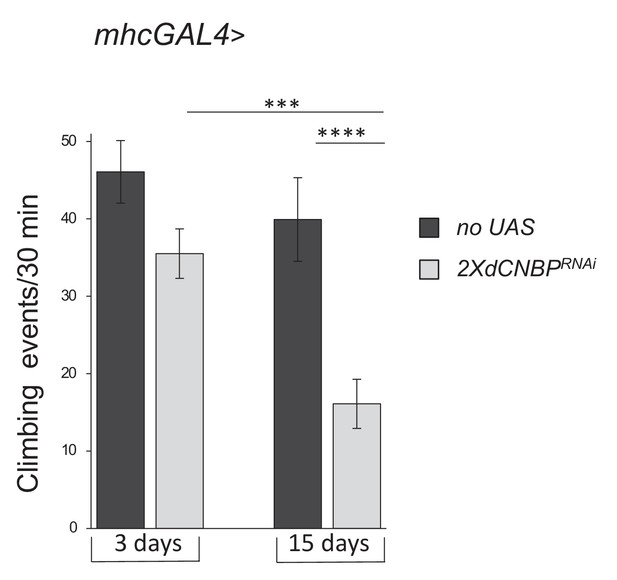
dCNBP-depleted flies exhibited an ageing-dependent locomotor dysfunction.
mhcGAL4 (no UAS) or mhcGAL4 > UASdCNBPRNAi-16283; UASdCNBPRNAi-16284 (2XUASdCNBPRNAi) reared at 29°C, male flies were assayed for negative geotaxis measured by the Drosophila activity monitoring (DAM) system at the indicated ages (error bars represent SEM; ***p<0.001; ****p<0.0001, Kruskal-Wallis with post hoc Dunn’s test); ≥15 males tested for each genotype in at least two independent experiments. Full data in Figure 7—source data 1.
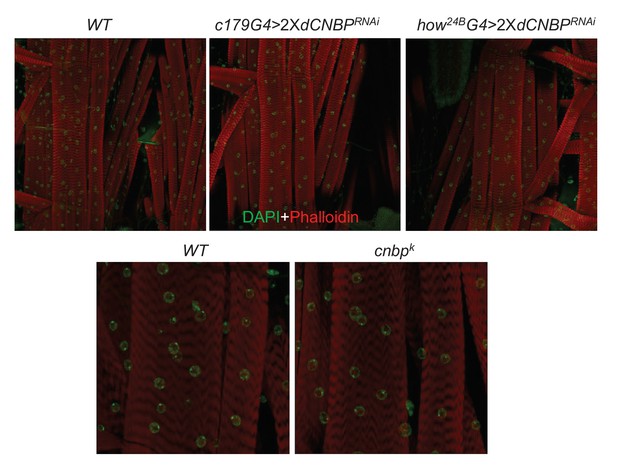
dCNBP depletion does not cause morphological changes of fly larval muscle tissues.
Muscle morphology and sarcomeric organization of the L2/L3 larval body wall muscles. Confocal images of larval muscles (segment A2; muscle 6/7) form wild-type (WT) controls, UASdCNBPRNAi-16283; UASdCNBPRNAi-16284 (2XdCNBPRNAi) driven by either how24BGAL4 or c179GAL4 as indicated (third instar larvae) or from cnbpk mutant (second instar larvae), stained with DAPI (green) and FITC phalloidin (red); ≥3 larvae have been tested for each genotype in at least two independent experiments.
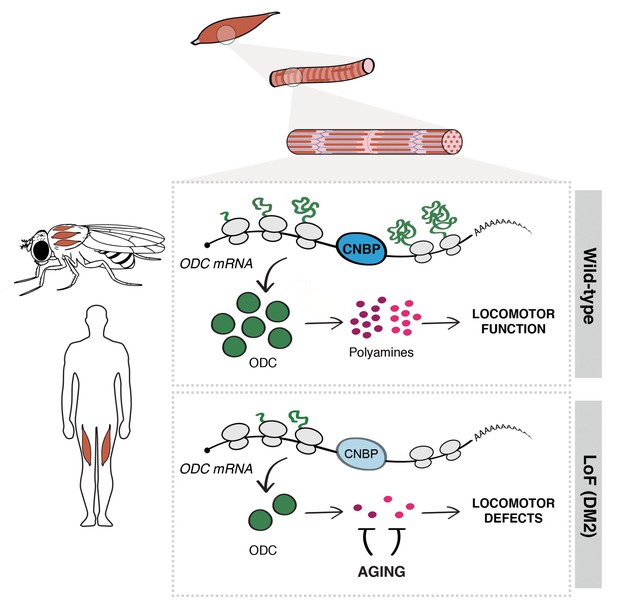
Cellular nucleic acid-binding protein (CNBP) controls muscle function by regulating polyamine metabolism.
Schematic representation of the mechanism of action of CNBP on muscle function in both Drosophila and humans. CNBP binds ornithine decarboxylase (ODC) mRNA and regulates its translation, leading to increased polyamine content. Lack of CNBP impairs locomotor function through ODC-polyamine downregulation.
Tables
Effects of dCNBP silencing using different tissue-specific GAL4 drivers.
Driver line | Expression pattern | 2XdCNBPRNAi at 29°C | 2XdCNBPRNAi at 25°C |
---|---|---|---|
tubulin-GAL4 | Constitutive-ubiquitous | Embryonic lethal | Larval lethal (third instar) |
actin-GAL4 | Constitutive-ubiquitous | Embryonic lethal | Larval lethal (third instar) |
elav-GAL4 | Pan-neuronal | No locom. phenotype | NT |
nrv-GAL4 | Pan-neuronal specific in CNS and PNS | No locom. phenotype | NT |
D42-GAL4 | Motor neurons | No locom. phenotype | NT |
n-syb-GAL4 | Pan-neuronal | No locom. phenotype | NT |
repo-GAL4 | Glia | No locom. phenotype | NT |
69B-GAL4 | Embryonic epiderm, CNS, and imaginal discs | Larval lethal (first instar) | Larval lethal. Escapers with locom. defects at 18°C |
mhc-GAL4 | Myosin heavy chain promoter | Reduced climbing activity | NT |
Mef2-GAL4 | Somatic muscle cells, embryonic mesoderm (stages 10–17), embryonic cardioblast | Embryonic lethal | Semi-lethal (pupal stage) Escapers with locom. defects |
c179-GAL4 | Embryonic mesoderm and larval muscles | Reduced larval activity | Pupal lethal |
how24B-GAL4 | Embryonic mesoderm. Precursors of the somatic muscles, visceral muscles, and cardiac cells. Larval nuclei of muscle fibers. | Reduced larval activity | Pupal lethal |
GMR-GAL4 | Eye imaginal disc | Retinal degeneration | NT |
nub-GAL4 | Wing imaginal disc | Wing size reduction and lost of patterning elements | Wing size reduction |
5053 GAL4 | Embryonic longitudinal visceral muscle founder cells | Vital and no adult locomotor phenotype | NT |
srmd710-GAL4 | Embryonic and larval tendon cells. No expression in muscle or muscle precursors | Vital and no adult locomotor phenotype | NT |
Reagent type (species) or resource | Designation | Source or reference | Identifiers | Additional information |
---|---|---|---|---|
Genetic reagent (Drosophila melanogaster) | actin-GAL4 | Bloomington | 25374 | y[1] w[*]; P{Act5C-GAL4-w}E1/CyO |
Genetic reagent (Drosophila melanogaster) | tubulin-GAL4 | Bloomington | 5138 | y[1] w[*]; P{w[+mC]=tubP-GAL4}LL7/TM3, Sb[1] Ser[1] |
Genetic reagent (Drosophila melanogaster) | elav-GAL4 | Bloomington | 77894 | y[1] w[*] P{w[+mC]=elav(FRT.ewg)GAL4.eFeG}1 |
Genetic reagent (Drosophila melanogaster) | nrv-GAL4 | Bloomington | 6799 | w[*]; P{w[+mC]=nrv2-GAL4.S}8 |
Genetic reagent (Drosophila melanogaster) | D42-GAL4 | Bloomington | 8816 | w[*]; P{w[+mW.hs]=GawB}D42 |
Genetic reagent (Drosophila melanogaster) | n-syb-GAL4 | Bloomington | 51635 | y[1] w[*]; P{w[+m*]=nSyb-GAL4.S}3 |
Genetic reagent (Drosophila melanogaster) | repo-GAL4 | Bloomington | 7415 | w[1118]; P{w[+m*]=GAL4} repo/TM3, Sb[1] |
Genetic reagent (Drosophila melanogaster) | 69B-GAL4 | Bloomington | 1744 | w[*]; P{w[+mW.hs]=GawB}69B |
Genetic reagent (Drosophila melanogaster) | Mhc-GAL4 | Bloomington | 38464 | ; w[*]; P{w[+mC]=Mhc-RFP.F3-580} |
Genetic reagent (Drosophila melanogaster) | Mef2-GAL4 | Bloomington | 26882 | w[*]; Kr[If-1]/CyO, P{w[+mC]=GAL4-Mef2.R}2, P{w[+mC]=UAS-mCD8.mRFP} |
Genetic reagent (Drosophila melanogaster) | c179-GAL4 | Bloomington | 6450 | w[*]; P{w[+mW.hs]=GawB}c179 |
Genetic reagent (Drosophila melanogaster) | how24B-GAL4 | Bloomington | 1767 | w[*]; P{w[+mW.hs]=GawB}how[24B] |
Genetic reagent (Drosophila melanogaster) | GMR-GAL4 | Bloomington | 9146 | w[1118]; P{GMR-GAL4.w[-]}2/CyO |
Genetic reagent (Drosophila melanogaster) | nub-GAL4 | Bloomington | 86108 | w[*]; P{w[nub.PK]=nub-GAL4.K}2 |
Genetic reagent (Drosophila melanogaster) | 5053 GAL4 | Bloomington | 2702 | w[*]; P{w[+mW.hs]=GawB}tey[5053A]/TM6B, Tb[+] |
Genetic reagent (Drosophila melanogaster) | srmd710-GAL4 | Bloomington | 26663 | w[*]; P{w[+mW.hs]=GawB}sr[md710]/TM6B, Tb[1] |
Genetic reagent (Drosophila melanogaster) | UAS dCNBPRNAi | VDRC | GD16283 | CNBP long hairpin on chromosome X |
Genetic reagent (Drosophila melanogaster) | UAS dCNBPRNAi | VDRC | GD16284 | CNBP long hairpin on chromosome 2 |
Genetic reagent (Drosophila melanogaster) | 2XUAS dCNBPRNAi | Antonucci et al., 2014 | GD16283+ GD16284 | - |
Genetic reagent (Drosophila melanogaster) | dCNBP k | Kyoto | 203535 | y[1] w[67c23]; P{w[+mC]=GSV6}GS11716 / SM1 |
Genetic reagent (Drosophila melanogaster) | UAS dCNBP-HA RNAi resistant | This study | – | Injection stock #BL 8622 |
Genetic reagent (Drosophila melanogaster) | UAS hCNBP-FLAG | This study | – | Injection stock #BL 8622 |
Genetic reagent (Drosophila melanogaster) | UAS Odc1RNAi | VDRC | GD30039 | Odc1 long hairpin on chromosome 3 |
Genetic reagent (Drosophila melanogaster) | UAS Odc1RNAi | VDRC | GD30038 | Odc1 long hairpin on chromosome 2 |
Genetic reagent (Drosophila melanogaster) | UAS Odc2RNAi | VDRC | KK104597 | Odc2 long hairpin on chromosome 2 |
Genetic reagent (Drosophila melanogaster) | UAS Odc1+Odc2RNAi | This study | GD30038+ KK104597 | Long hairpin for Odc2 on chromosome 2 and for Odc1 on chromosome 3 |
Genetic reagent (Drosophila melanogaster) | dOdc1 mutant | Bloomington | 56103 | y[1] w[*]; Mi{y[+mDint2]=MIC}Odc1[MI10996] |
Genetic reagent (Drosophila melanogaster) | UAS dOdc1 | Gupta et al., 2013 | - | - |
Antibody | anti-CNBP (goat) | Abcam | ab48027, RRID:AB870003 | WB 1:1000 |
Antibody | anti-ODC (rabbit) | ENZO | BML-PW8880-0100 RRID:AB_2156495 | WB 1:500 |
Antibody | anti-Actin goat | Santa Cruz | sc-1616, RRID:AB630836 | WB 1:3000 |
Antibody | anti-GFP (mouse) | Santa Cruz | sc-9996, RRID:AB_627695 | WB 1:3000 |
Antibody | anti-Vinculin (mouse) | Santa Cruz | sc-73614, RRID:AB_1131294 | WB 1:3000 |
Antibody | anti-FLAG-HRP | Sigma | A8592, RRID:AB_439702 | WB 1:500 |
Antibody | anti-CNBP (mouse) | Agrobio (this study) | – | WB 1:1000 |
Antibody | anti-HA-HRP (mouse) | Santa Cruz | sc-7392, RRID:AB_627809 | WB 1:2000 |
Antibody | anti-vibrator (rabbit) | Giansanti et al., 2006 | - | WB 1:3000 |
Sequence-based reagent | T7 CNBP FW Drosophila melanogaster | This study | dsRNA primer | TAATACGACTCACTATAGGGAG GTCCGGGCGGCGTTGG |
Sequence-based reagent | T7 CNBP RV Drosophila melanogaster | This study | dsRNA primer | TAATACGACTCACTATAGGGAG ATGTGTCCGGTGCGG |
Sequence-based reagent | dOdc1 Fw Drosophila melanogaster | This study | PCR primer | TGGCAGCGATGACGTAAAGTT |
Sequence-based reagent | dOdc1 Rv Drosophila melanogaster | This study | PCR primer | TGGTTCGGCGATTATGTGAA |
Sequence-based reagent | GAPDH Fw Drosophila melanogaster | This study | PCR primer | CCTGGCCAAGGTCATCAATG |
Sequence-based reagent | GAPDH RvDrosophila melanogaster | This study | PCR primer | ATGACCTTGCCCACAGCCTT |
Sequence-based reagent | dOdc1-IRES FW | This study | PCR primer | TAAGAATTCCTCGGAAAGATCTCAAC |
Sequence-based reagent | dOdc1-IRES RW | This study | PCR primer | TTAGAATTCACAAGTCGT TGACTGATAAC |
Chemical compound, drug | DFMO | Sigma | #D193 | |
Commercial assay or kit | RevertAid H Minus First Strand cDNA Synthesis kit | Thermo Fisher Scientific | K1632 | |
Chemical compound, drug | Putrescine | Sigma | #51799 | |
Chemical compound, drug | Spermidine | Sigma | #S2626 | |
Plasmids | plko SCR, plkoSh_cnbp | D'Amico et al., 2015 | ||
Plasmids | GFP | Coni et al., 2020 | ||
Plasmids | hODC-LUC | D'Amico et al., 2015 | ||
Plasmids | dOdc1-LUC | This study | ||
Plasmids | HA-dCNBP | Antonucci et al., 2014 | ||
Plasmids | FLAG-hCNBP | D'Amico et al., 2015 | ||
Cell line | S2 | DGRC | Cat# 181, RRID:CVCL_Z992 | |
Cell line | HEK-293T | ATCC | CRL-3216, RRID:CVCL_0063 |
Additional files
-
Source data 1
Original Blots for all Western Blot experiments.
- https://cdn.elifesciences.org/articles/69269/elife-69269-data1-v1.pptx
-
Source data 2
Single Original Blots for all Western Blot experiments in tiff format.
- https://cdn.elifesciences.org/articles/69269/elife-69269-data2-v1.zip
-
Source data 3
Western Blot quantifications as shown in Figure 1E, Figure 1—figure supplement 1, Figure 2B, Figure 3A, Figure 3—figure supplement 1A, Figure 5A, Figure 5—figure supplement 1, Figure 6—figure supplement 3, Figure 1—figure supplement 4A, Figure 7—figure supplement 2.
- https://cdn.elifesciences.org/articles/69269/elife-69269-data3-v1.xlsx
-
Transparent reporting form
- https://cdn.elifesciences.org/articles/69269/elife-69269-transrepform-v1.pdf