Heart Attack: Working together
A healthy heart relies on a close network of organs to work properly. Indeed, its cells require high levels of energy which is provided by biomolecules transported in the blood from other organs. If the blood supply is blocked, this can result in a heart attack which damages the heart’s muscle cells leading to the organ beating irregularly or, in some cases, failing all together. Our current understanding of heart attacks is limited to examining the alterations to tissues in the heart, but less is known about what happens in other organs.
Recent advances in molecular methods have begun to shed light on this issue, showing, for instance, that gene expression in the spleen and liver of pigs is significantly modified following a heart attack (Zimmermann et al., 2017). Computer simulations can predict the molecular alterations taking place during complex diseases, and could be harnessed to examine how different tissues respond to a heart attack (Priest and Tontonoz, 2019). Now, in eLife, Adil Mardinoglu (Royal Institute of Technology), Jan Boren (Sahlgrenska University Hospital) and colleagues – including Muhammad Arif and Martina Klevstig as joint first authors – report how they used this method, and other techniques, to investigate how heart attacks alter fat, skeletal muscle and liver tissues (Arif et al., 2021).
To identify which genes are altered in these tissues, the team (who are based in Sweden, Turkey and the United Kingdom) triggered acute heart attacks in mice by surgically blocking some of the arteries that supply blood to muscle cells in the heart (Figure 1). They then collected samples from the mice’s heart, skeletal muscle, fat tissue and liver, and measured how every single protein-coding gene was expressed using a highly sensitive technique called RNA sequencing. Comparing results from the heart attack model to tissue samples from control mice revealed striking changes in a number of genes, including four that had not previously been associated with this disease (Flnc, Lgals3, Prkaca and Pprc1). Most of these molecular changes took place after 24 hours, with the most profound effects occurring in the heart’s tissues.
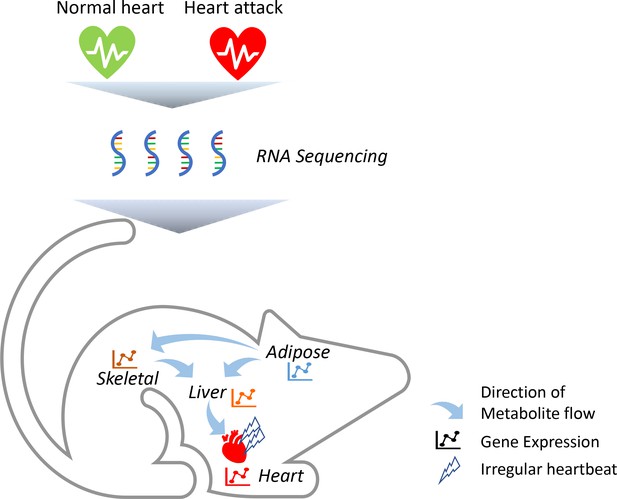
Heart attacks cause molecular changes in multiple tissues.
Acute heart attacks were induced in mice by blocking some of the arteries that supply blood to the heart, resulting in an irregular heartbeat. Samples were then collected from the heart, skeletal muscle, liver and adipose, or 'fat', tissues of these mice (red heart) and control animals that had not undergone a heart attack (green heart). RNA sequencing revealed that the heart attack altered genes expressed in all four of these tissues. Further computational simulations suggest that these genetic changes alter the production of metabolites that are then taken up by the other tissues (represented by arrows).
Image credit: Regan Odongo.
Further analysis showed that genes associated with the immune system, RNA processes and mitochondrial function were commonly altered in the heart, skeletal muscle and fat tissues. Arif et al. also found groups of genes that were only modified in specific tissues. These unique clusters had a variety of roles, ranging from reacting to the heart attack to breaking down metabolites (biomolecules derived from glucose) so muscle cells in the heart can adequately respond to the injury. These findings suggest that the molecular changes induced by a heart attack have a ripple effect that changes how genes are expressed in other organs.
Next, Arif et al. performed computational simulations to predict which of the metabolites produced by these tissues might have an important role in supplying energy to the heart. To do this, they used a technique called reporter metabolite analysis which identifies key metabolites based on the genes expressed (Patil and Nielsen, 2005). Some of the metabolites were detected in all four tissues, while others were only found in either the heart, skeletal muscle, fat or liver. These metabolites were consistent with physiological changes commonly observed in patients that have experienced a heart attack (Doenst et al., 2013).
Advanced computational simulations were then conducted on a model in which all four tissues are linked together by blood vessels. This predicted that some of the metabolites synthesised by the liver are released into the blood and taken up by the heart’s tissues (Figure 1). The model also showed that some of the metabolites frequently found in the blood samples of heart attack patients are released from fat tissues and taken up by the liver and skeletal muscles. Taken together, these findings suggest that the heart, skeletal muscles, fat and liver all have a distinct molecular response to a heart attack. However, these genetic changes allow the tissues to ‘talk to each other’ and exchange metabolites that help the heart respond adequately to the damage caused by the heart attack.
This work provides novel insights into how spatially separated tissues with different embryonic origins can still coordinate after a heart attack. It also highlights how multi-tissue computational simulations can be used to unravel complex diseases. In future studies it will be critical to determine whether a similar multi-tissue crosstalk occurs in chronic heart attacks (which are more common), and if drugs that alter these molecular processes could improve the outcome for patients.
References
-
Cardiac metabolism in heart failure: implications beyond ATP productionCirculation Research 113:709–724.https://doi.org/10.1161/CIRCRESAHA.113.300376
-
Inter-organ cross-talk in metabolic syndromeNature Metabolism 1:1177–1188.https://doi.org/10.1038/s42255-019-0145-5
Article and author information
Author details
Publication history
- Version of Record published: June 8, 2021 (version 1)
Copyright
© 2021, Odongo and Çakır
This article is distributed under the terms of the Creative Commons Attribution License, which permits unrestricted use and redistribution provided that the original author and source are credited.
Metrics
-
- 706
- views
-
- 33
- downloads
-
- 0
- citations
Views, downloads and citations are aggregated across all versions of this paper published by eLife.
Download links
Downloads (link to download the article as PDF)
Open citations (links to open the citations from this article in various online reference manager services)
Cite this article (links to download the citations from this article in formats compatible with various reference manager tools)
Further reading
-
- Computational and Systems Biology
- Developmental Biology
Organisms utilize gene regulatory networks (GRN) to make fate decisions, but the regulatory mechanisms of transcription factors (TF) in GRNs are exceedingly intricate. A longstanding question in this field is how these tangled interactions synergistically contribute to decision-making procedures. To comprehensively understand the role of regulatory logic in cell fate decisions, we constructed a logic-incorporated GRN model and examined its behavior under two distinct driving forces (noise-driven and signal-driven). Under the noise-driven mode, we distilled the relationship among fate bias, regulatory logic, and noise profile. Under the signal-driven mode, we bridged regulatory logic and progression-accuracy trade-off, and uncovered distinctive trajectories of reprogramming influenced by logic motifs. In differentiation, we characterized a special logic-dependent priming stage by the solution landscape. Finally, we applied our findings to decipher three biological instances: hematopoiesis, embryogenesis, and trans-differentiation. Orthogonal to the classical analysis of expression profile, we harnessed noise patterns to construct the GRN corresponding to fate transition. Our work presents a generalizable framework for top-down fate-decision studies and a practical approach to the taxonomy of cell fate decisions.
-
- Computational and Systems Biology
- Genetics and Genomics
We propose a new framework for human genetic association studies: at each locus, a deep learning model (in this study, Sei) is used to calculate the functional genomic activity score for two haplotypes per individual. This score, defined as the Haplotype Function Score (HFS), replaces the original genotype in association studies. Applying the HFS framework to 14 complex traits in the UK Biobank, we identified 3619 independent HFS–trait associations with a significance of p < 5 × 10−8. Fine-mapping revealed 2699 causal associations, corresponding to a median increase of 63 causal findings per trait compared with single-nucleotide polymorphism (SNP)-based analysis. HFS-based enrichment analysis uncovered 727 pathway–trait associations and 153 tissue–trait associations with strong biological interpretability, including ‘circadian pathway-chronotype’ and ‘arachidonic acid-intelligence’. Lastly, we applied least absolute shrinkage and selection operator (LASSO) regression to integrate HFS prediction score with SNP-based polygenic risk scores, which showed an improvement of 16.1–39.8% in cross-ancestry polygenic prediction. We concluded that HFS is a promising strategy for understanding the genetic basis of human complex traits.