Expression of a CO2-permeable aquaporin enhances mesophyll conductance in the C4 species Setaria viridis
Figures
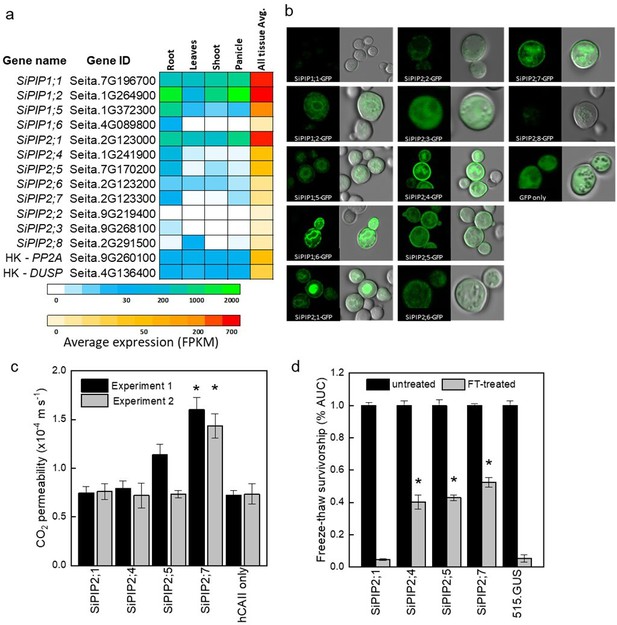
Identification of the CO2-permeable aquaporin SiPIP2;7 from Setaria italica.
(a) Expression atlas of the SiPIP genes generated from Phytomine reported as Fragments Per Kilobase of transcript per Million mapped reads (FPKM). House-keeping genes (HK) PROTEIN PHOSPHATASE 2A (PP2A) and DUAL SPECIFICITY PROTEIN (DUSP) were included for reference. (b) Localization of SiPIP-GFP fusions expressed in yeast visualised with confocal microscopy; left panels – GFP fluorescence; right panels – bright field overlaid with GFP fluorescence. Measured cell diameters are shown in Figure 1—figure supplement 2. (c) CO2 permeability assay on yeast co-expressing SiPIPs and human CARBONIC ANHYDRASE II (hCAII) analyzed by stopped-flow spectrometry (see Figure 1—figure supplement 2 for details). ‘hCAII only’ expression was used as negative control. Mean±SE, n=3 biological replicates. Two independent experiments are presented. Asterisks indicate statistically significant differences between yeast expressing SiPIPs and ‘hCAII only’ control (t-test, p<0.05). (d) Yeast water permeability was assessed in the yeast aquaporin deletion background (aqy1/2) by the cumulative growth between untreated and freeze-thawed cells and determined by the percent area under the curve (% AUC). The yeast expressing the β-glucuronidase reporter gene (515.GUS) was used as negative control. Mean±SE, n=4 biological replicates. Asterisks indicate statistically significant differences between yeast expressing SiPIPs and 515.GUS control (t-test, p<0.01).
-
Figure 1—source data 1
Gene expression and yeast assays.
- https://cdn.elifesciences.org/articles/70095/elife-70095-fig1-data1-v2.xlsx
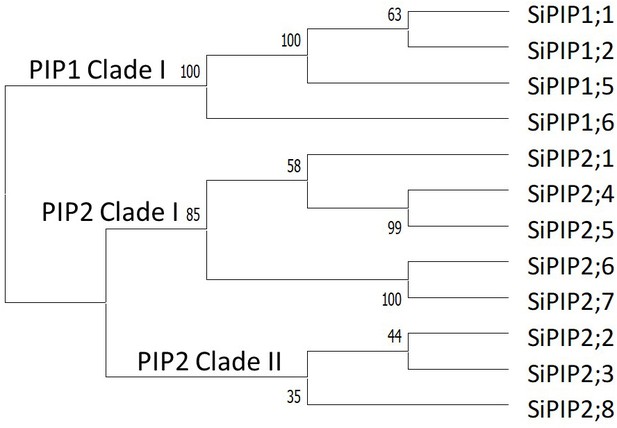
Phylogenetic analysis of Setaria italica PIP protein sequences.
Amino acid sequences for the PIP1 and PIP2 proteins from S. italica were acquired from Phytozome and numbered based on McGaughey et al., 2016. The protein sequences were aligned using MUSCLE in Geneious (v11.1.5) and the phylogenetic tree generated using the neighbor-joining method with pairwise deletions in MEGA10 (Kumar et al., 2018).
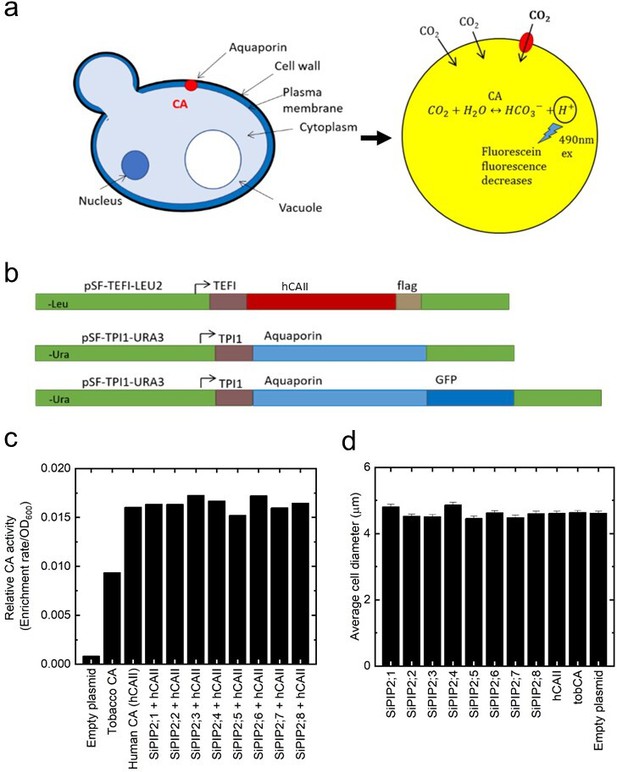
Expression of Setaria italica PIPs in yeast for functional testing of CO2 permeability.
(a) Schematic highlighting the yeast expression system and the detection of CO2 permeability by measuring the change in fluorescein fluorescence due to the acidification of the cytoplasm by producing protons from the hydration of CO2 from ectopically expressed carbonic anhydrase (CA). The decline in fluorescence is measured by stop-flow spectrophotometry at 490 nm. (b) Construct design used for expression and assay of CO2 permeability and localization in yeast. (c) CA activity measured in yeast cells expressing PIPs. (d) The cell diameter of yeast cells expressing PIPs.
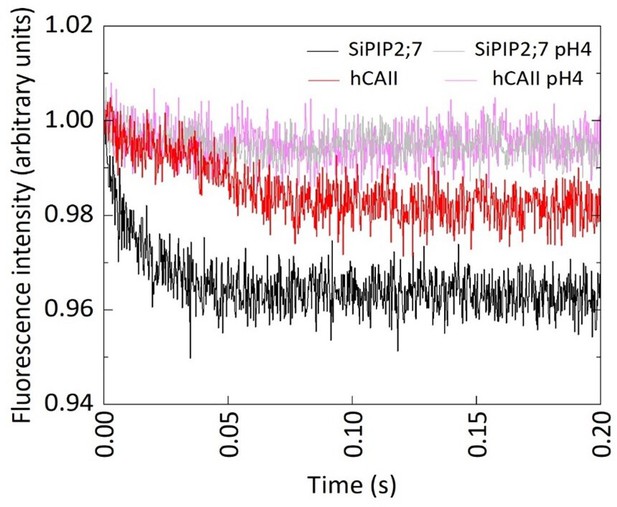
CO2 transport capacity of SiPIP2;7 is not confounded by permeability of protons.
The changes in CO2 permeability, detected on the stopped-flow spectrophotometer (Figure 1c), could be confounded by other factors such as permeability to protons also causing intracellular acidification. When SiPIP2;7+ hCAII (black) and hCAII alone (red) were injected alongside CO2 enriched buffer, a decrease in pH was observed. This decrease was more pronounced in PIP2;7+ hCAII indicating higher CO2 transport rate. When SiPIP2;7+ hCAII and hCAII were injected with a low pH (i.e., proton-rich) buffer, no decrease in fluorescence intensity was observed with either SiPIP2;7+ hCAII (gray) or hCAII alone (pink) demonstrating that the decrease in fluorescence seen with the CO2 enriched was not due to proton movement through the membrane. In fact, both traces were the same specifically pointing out that SiPIP2;7 expression did not cause excessive proton movement which could lead to a false-positive result for CO2 permeability.

SiPIP2;7 is a functional water channel when expressed in Xenopus laevis oocytes.
Oocytes were injected with either water (control) or 23 ng of SiPIP2;7 cRNA. Osmotic permeability (Pos) was determined via photometric swelling where oocytes were pre-incubated in the isotonic swelling solution before being transferred to the hypotonic swelling solution for the assay. Mean±SE, p<0.0001 (t-test).
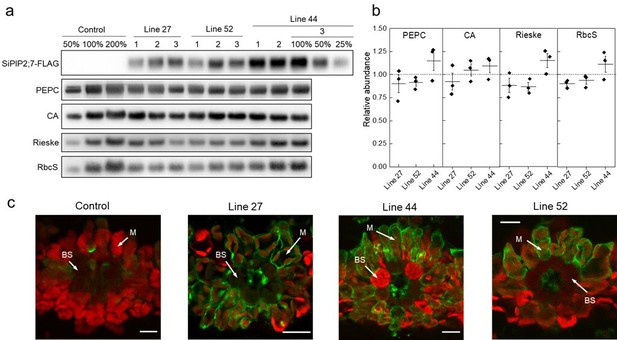
Characterization of Setaria viridis plants expressing SiPIP2;7-FLAG in mesophyll cells.
(a) Immunodetection of SiPIP2;7-FLAG and photosynthetic proteins in leaf protein samples loaded on leaf area basis. Three plants from each of the three transgenic lines were analyzed and dilution series of the control and line 44-3 samples were used for relative quantification. (b) Protein abundances calculated from the immunoblots relative to control plants. Mean±SE. No significant difference was found between the transgenic and control plants (t-test). (c) Immunolocalization of SiPIP2;7-FLAG on leaf cross-sections visualized with confocal microscopy. Fluorescence signals are pseudo-colored: green – FLAG antibodies labelled with secondary antibodies conjugated with Alexa Fluor 488; red – chlorophyll autofluorescence. BS, bundle sheath cell; M, mesophyll cell. Scale bars = 20 µm. Azygous plants of line 44 were used as control. Uncropped images of the blots are provided in Figure 2—source data 1.
-
Figure 2—source data 1
Uncropped images of western blots.
- https://cdn.elifesciences.org/articles/70095/elife-70095-fig2-data1-v2.docx

Immunodetection of SiPIP2;7-FLAG in leaves of transgenic Setaria viridis plants.
(a) T0 plants; T-DNA insertion numbers indicate the number of hpt gene copies detected by droplet digital PCR. Lines selected for further analysis are marked with asterisks. (b) Homozygous T3 plants; azygous plants of line 44 were used as control. Uncropped images of the blots from (a) are provided in Figure 2—figure supplement 1—source data 1; uncropped images of the blot from (b) are provided in Figure 2—figure supplement 1—source data 1.
-
Figure 2—figure supplement 1—source data 1
Uncropped images of western blots.
- https://cdn.elifesciences.org/articles/70095/elife-70095-fig2-figsupp1-data1-v2.zip
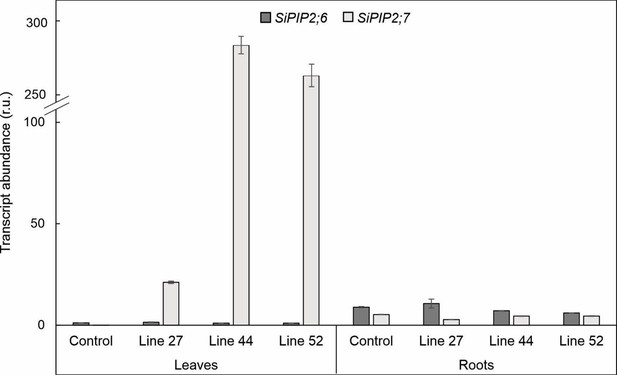
qPCR analysis of SiPIP2;6 and SiPIP2;7 expression in leaves and roots of control and transgenic Setaria viridis plants.
Azygous plants of line 44 were used as control.
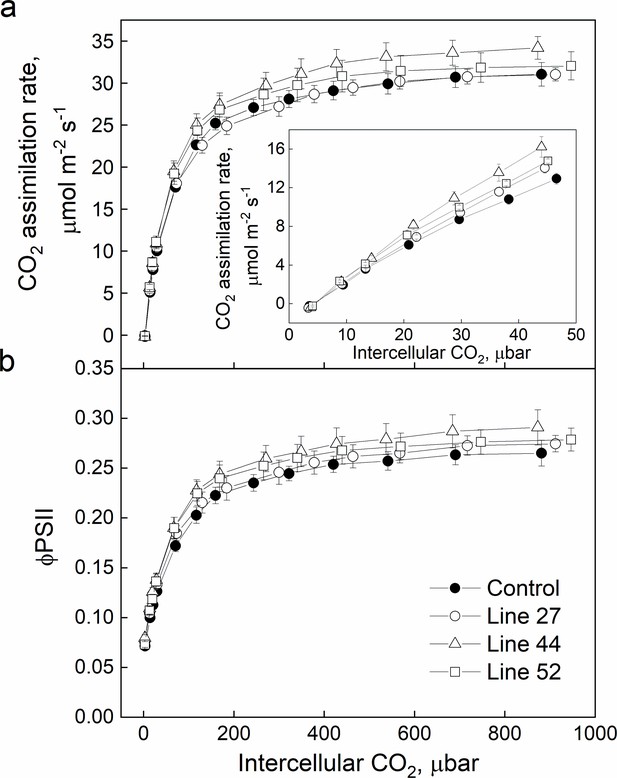
CO2 response of CO2 assimilation rate (a) and quantum yield of Photosystem II (b) in Setaria viridis plants expressing SiPIP2;7-FLAG in mesophyll cells.
Measurements were performed at the irradiance of 1500 µmol m−2 s−1; azygous plants of line 44 were used as control. Mean±SE, n=4–6 biological replicates. No significant difference was found between the transgenic and control plants (one-way ANOVA, α=0.05).
-
Figure 3—source data 1
Gas exchange and fluorescence analysis.
- https://cdn.elifesciences.org/articles/70095/elife-70095-fig3-data1-v2.xlsx
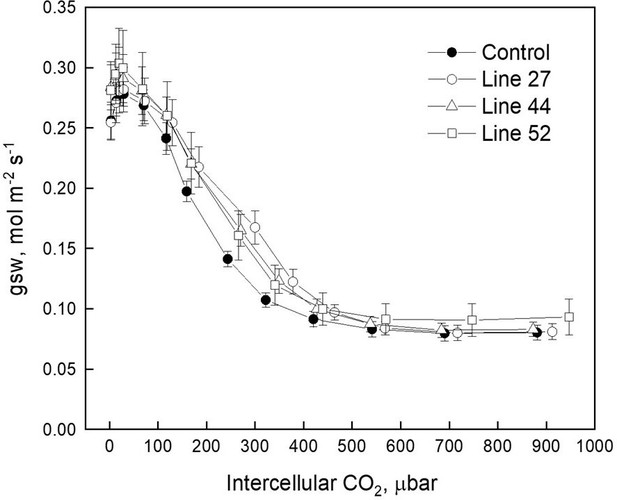
Stomatal conductance to water vapor (gsw) measured at different intercellular CO2 partial pressure.
No significant differences were detected between the transgenic and control plants (one-way ANOVA, α=0.05).
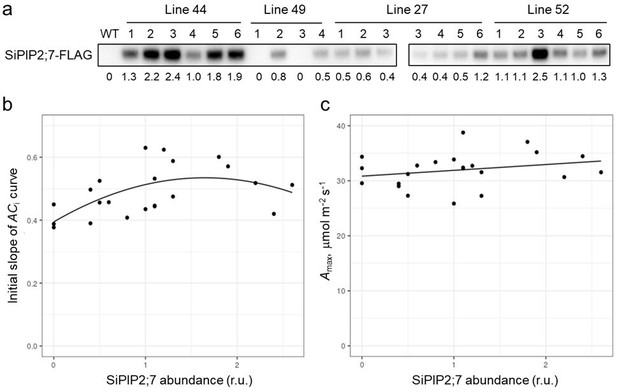
The relationship between the relative abundance of SiPIP2;7 and the initial slopes or the maximum assimilation rates (Amax) of the ACi curves in individual T1 plants of four transgenic lines.
(a) Immunodetection of the SiPIP2;7-FLAG in plants is on a leaf area basis. Quantification of immunoblot band intensities is shown underneath the bands in relative units. (b) The initial slopes compared to the relative SiPIP2;7 content. A second order polynomial was fitted using R, producing an R2 of 0.345 (p<0.05, F=5.007, df=2 and 19), which was significantly better than a linear fit when compared using ANOVA (p<0.05). No significant improvement of the model could be found adding random effects to account for transformation event or zygosity of lines. Both polynomial coefficients were significant to the model (p<0.05). (c) Amax compared to the relative SiPIP2;7 content. A linear model was fitted using R. No significant effects could be found, with R2 of the model being only 0.065; addition of random terms to this analysis were not found to be of any advantage.
-
Figure 3—figure supplement 2—source data 1
Uncropped images of western blots.
- https://cdn.elifesciences.org/articles/70095/elife-70095-fig3-figsupp2-data1-v2.zip

Effect of the mesophyll conductance, gm, on the initial slope of the CO2 assimilation response curve to the intercellular CO2 partial pressure (ACi curve) in leaves of Setaria viridis expressing SiPIP2;7-FLAG in mesophyll cells.
(a) Initial slope of the ACi curves estimated by linear fitting of curves (a subset of curves is presented in Figure 3a inset). (b) Mesophyll conductance, gm, estimated by oxygen isotope discrimination assuming full isotopic equilibrium (Osborn et al., 2017). Measurements were made at ambient CO2 and low O2. (c) Data from (a) and (b) compared to the C4 biochemical model predictions (von Caemmerer, 2000; von Caemmerer and Furbank, 1999). All graphs show Mean±SE; azygous plants of line 44 were used as control. The model relates the initial slope of the ACi curve (dA/Ci) to gm by: , where Vpmax and Kp denote the maximum PEPC activity and the Michaelis Menten constant for CO2 taken here as 250 µmol m–2 s–1 and 82 µbar (DiMario and Cousins, 2019; von Caemmerer, 2021). Asterisks indicate statistically significant differences between the plants of lines 27 (p=0.04573) and 44 (p=0.03724) and control plants (two-way ANOVA with Tukey post hoc test, α=0.05). Plants of line 52 were not significantly different from the control plants (p=0.27518).
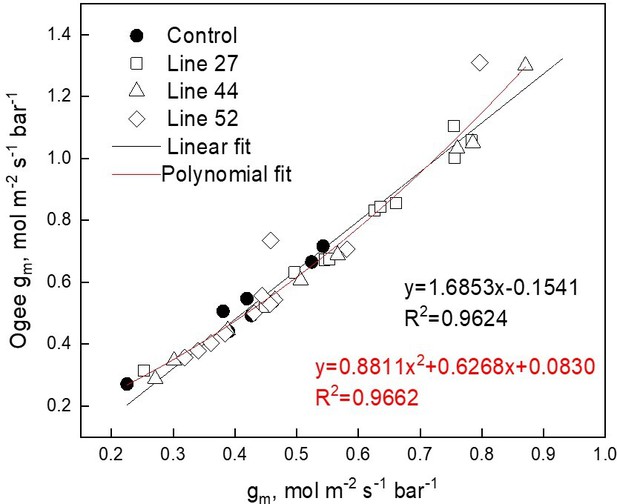
Comparison of the gm estimated by oxygen isotope discrimination assuming full isotopic equilibrium (Osborn et al., 2017) and calculations suggested by Ogée et al., 2018.
Tables
Properties of Setaria viridis plants expressing SiPIP2;7-FLAG in mesophyll cells.
PEPC, PEP carboxylase; Rubisco, ribulose bisphosphate carboxylase oxygenase; LMA, leaf mass per area. Azygous plants of line 44 were used as control. Mean±SE, n=3 except for biomass (n=8). Three-weeks old plants before flowering were used for all analyses. No significant difference was found between the transgenic and control plants (one-way ANOVA, α=0.05).
Parameter | Control | Line 27 | Line 44 | Line 52 |
---|---|---|---|---|
PEPC activity, µmol CO2 m–2 s–1 | 220.1±25.8 | 197.6±12.7 | 208.7±7.9 | 218.5±3.5 |
CA hydration rate, mol m–2 s–1 bar–1 | 6.50±0.10 | 6.32±0.22 | 5.34±0.67 | 5.35±0.56 |
Rubisco active sites, µmol m–2 | 12.17±0.63 | 12.53±0.54 | 12.84±0.13 | 12.63±0.74 |
Chlorophyll (a+b), mmol m–2 | 0.71±0.07 | 0.72±0.04 | 0.72±0.05 | 0.72±0.08 |
Chlorophyll a/b | 5.01±0.16 | 5.08±0.05 | 4.97±0.09 | 5.07±0.15 |
LMA, g (dry weight) m–2 | 23.6±1.6 | 24.0±1.5 | 25.6±1.3 | 25.4±1.3 |
Shoot biomass, g (dry weight) plant–1 | 2.06±0.36 | 2.01±0.20 | 2.23±0.31 | 2.24±0.34 |
Root biomass, g (dry weight) plant–1 | 0.27±0.07 | 0.28±0.03 | 0.34±0.06 | 0.35±0.05 |
Additional files
-
Supplementary file 1
Amino acid similarity of S. italica and S. viridis PIPs.
- https://cdn.elifesciences.org/articles/70095/elife-70095-supp1-v2.xlsx
-
Supplementary file 2
Amino acid composition of S. italica PIPs at known substrate selectivity positions.
Aquaporins have six transmembrane α-helices (H1-H6) joined by five loops (LA-LE). The monomeric channel is characterized by two NPA (Asn, Pro, Ala) motifs on loops A and E and along with the aromatic/arginine selectivity filter, these largely dictate the transport selectivity of substrates through the monomeric channels (Azad et al., 2016). Froger’s positions indicate additional residues that are predicted for substrate specificity (Froger et al., 1998).
- https://cdn.elifesciences.org/articles/70095/elife-70095-supp2-v2.xlsx
-
Supplementary file 3
Primers used for qPCR.
- https://cdn.elifesciences.org/articles/70095/elife-70095-supp3-v2.xlsx
-
Transparent reporting form
- https://cdn.elifesciences.org/articles/70095/elife-70095-transrepform1-v2.docx