Loss of N1-methylation of G37 in tRNA induces ribosome stalling and reprograms gene expression
Figures
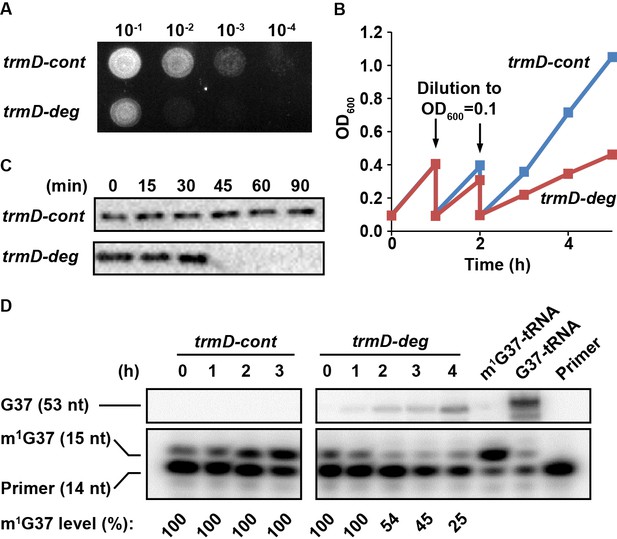
Conditional m1G37 deficiency of the E. coli trmD-deg strain.
(A) Growth of trmD-deg and trmD-cont G78 cells on an LB plate. An overnight culture of trmD-deg and trmD-cont cells in LB + Glc was serially diluted, spotted on an LB + Ara plate to turn on clpXP expression, and incubated at 37°C overnight. (B) Representative growth of trmD-deg and trmD-cont G78 cells in a liquid LB culture. An overnight culture of trmD-deg and trmD-cont G78 cells in LB + Glc was grown in LB for 1–2 hr, then diluted into LB + Ara at OD600 of 0.1 and grown to OD600 of 0.3 at 37°C. The cycle of dilution and re-growth was repeated three times to observe a growth defect of the trmD-deg strain. (C) Western blot analysis of TrmD protein. An overnight culture of trmD-deg and trmD-cont cells in LB + Glc was diluted to LB + Ara at T = 0, and was sampled at the indicated time points. Cell lysates were separated on a 12% SDS-PAGE and TrmD protein was detected by primary antibody against E. coli TrmD and a secondary antibody against rabbit IgG. (D) Primer-extension inhibition analysis. An overnight culture of trmD-deg and trmD-cont cells in LB + Glc was diluted to LB + Ara at T = 0 and the fresh culture was taken through three cycles of dilution and re-growth. Total RNA was extracted over the time course, probed with a 5'-[32P]-labeled DNA primer targeting E. coli tRNALeu/CAG, and analyzed by a 12% PAGE/7 M urea gel and phosphorimaging. Primer extension would terminate in the control at 1 nt downstream of m1G37, generating a 15 nt fragment, whereas primer extension would continue to the 5'-end in TrmD deficiency, generating a 53 nt fragment. The fraction of m1G37 in each sample is calculated based on all primer-extension products as shown in the source file, not including the primer. Because the same amount of cell culture was used for extraction of RNA, an increased primer-extension stop at the 15 nt band relative to the primer position was observed in trmD-cont cells, reflecting an increased cell density 0–3 hr and increased synthesis of m1G37-tRNA. In all samples collected for trmD-cont cells, no synthesis of the read-through 53 nt band was observed, indicating 100% methylation. At the time of cell harvest, the m1G37 level was 100% for trmD-cont cells at T = 3 hr, but was below 25% for trmD-deg cells at T = 4 hr.
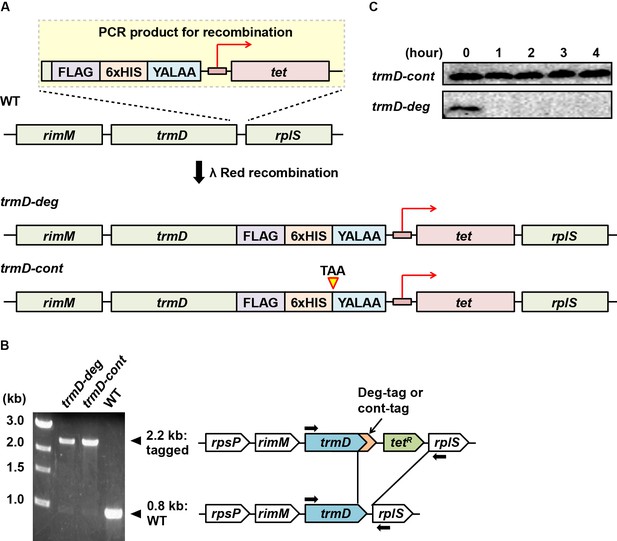
Genetic construction and validation ofE. coli trmD-degandtrmD-contstrains.
(A) Construction of E. coli trmD-deg and trmD-cont strains based on the genomic context. A PCR product was amplified to contain nucleotide sequences for a FLAG tag, a 6xHis tag, the degron peptide YALAA, and a tetracycline resistance marker with its promoter. This PCR product contained terminal extensions that were homologous to the 3'-end and the 3'-flanking region of E. coli chromosomal trmD and it was integrated into the chromosome trmD locus by λRed recombination, generating the trmD-deg strain. To generate the trmD-cont strain, the same PCR product was used except that a stop codon TAA was added before the sequence for the YALAA tag. (B) Genotype confirmation by PCR for trmD-deg and trmD-cont strains. Colony PCR using primers targeting the 5'-terminal and 3'-flanking regions of trmD (shown by arrows) confirmed clones of trmD-deg and trmD-cont strains. The deg-tag adds to the C-terminus of TrmD with the YALAA peptide, which is targeted by the ClpXP degradation system, whereas the cont-tag does not. The clones were streak-purified until the WT band was completely removed. The mutant genomic trmD locus was introduced by P1 transduction to G78 cells, which lack the chromosomal clpX gene. (C) Western blot analysis of TrmD protein over an extended time course. E. coli trmD-deg and trmD-cont strains were inoculated to LB + Ara (0.2%) grown at 37°C for 4 hr. Each culture was diluted to OD = 0.1 at the indicated time to maintain cells in the log phase and TrmD was probed by Western blot as in Figure 1C.
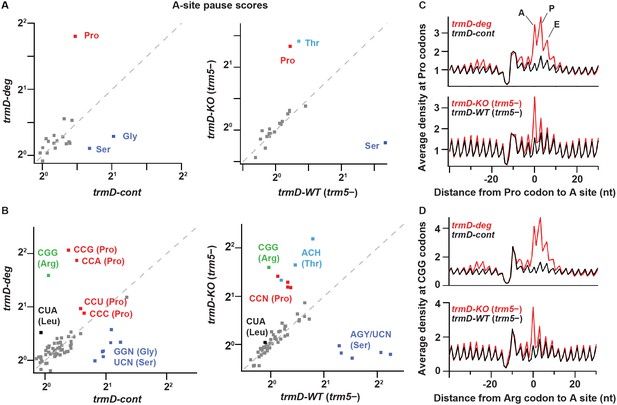
TrmD depletion leads to ribosome pausing at CCN (Pro) and CGG (Arg) codons.
(A) Pause scores for codons positioned in the ribosomal A site. The codons are grouped by amino acid. Left panel: data from biological replicates of trmD-deg and trmD-cont strains upon ClpXP induction. Each biological replicate is an independent culture of cells. Right panel: data from trmD-KO (trm5–) and trmD-WT (trm5–) strains upon turning off trm5. (B) The same as above but showing the 61 sense codons individually. (C) Plots of average ribosome density aligned at Pro codons for trmD-deg strain (top) and trmD-KO (trm5–) strain (bottom) in red with their respective control strains in black. The peaks corresponding to the ribosomal A, P, and E sites are labeled. (D) Plots of average ribosome density aligned at CGG codons as above. Note that trmD-deg samples were obtained with the traditional ribosome profiling lysis buffer, whereas trmD-KO (trm5–) samples were obtained with a high Mg2+ lysis buffer that more effectively arrests translation after cell lysis, revealing these pauses at higher resolution.
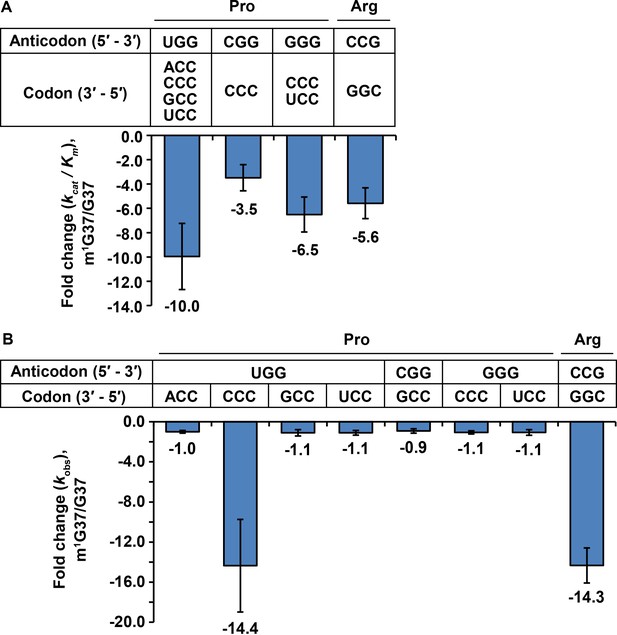
Kinetic parameters showing the loss of tRNA aminoacylation and peptide-bond formation at the A site in m1G37 deficiency.
(A) Fold-change in the loss of kcat/Km of tRNA aminoacylation from the m1G37-state to the G37-state. Bar graphs are shown for the UGG, the CGG, and the GGG isoacceptors of E. coli tRNAPro, and for the CCG isoacceptor of E. coli tRNAArg, with the fold-change value displayed at the bottom of each bar. The bars are SD of three independent replicates (n = 3), and the data are presented as the mean ± SD for each sample. (B) Fold-change in the loss of kobs from the m1G37-state to the G37-state in overall reaction at the A site leading to peptide-bond formation. Bar graphs are shown for the UGG, CGG, and GGG isoacceptors of E. coli tRNAPro, and for the CCG isoacceptor of E. coli tRNAArg. Each tRNA is paired to the cognate codon, and the fold-change value is displayed at the bottom of each bar as the mean ± SD of three independent replicates (n = 3).
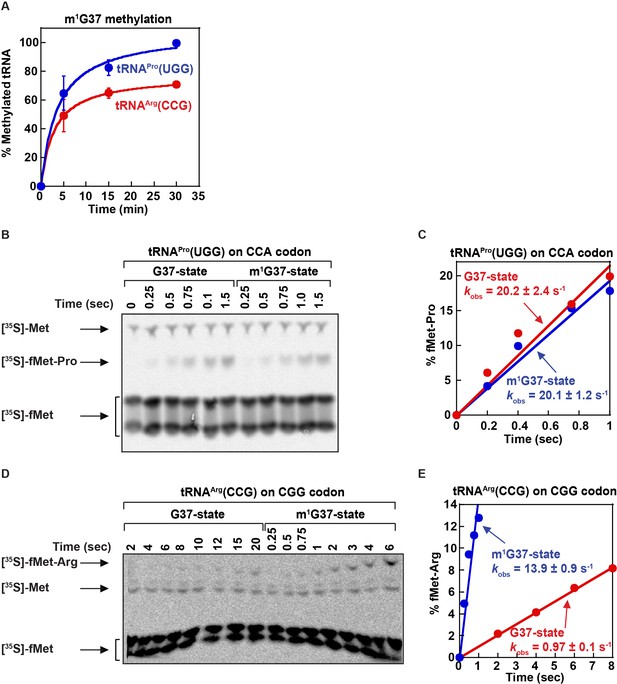
The in vitro reactions for tRNA methylation and peptide-bond formation.
(A) Efficiency of m1G37 methylation by TrmD in vitro to generate the m1G37-state tRNA substrates for biochemical assays performed in Figure 3. Reactions were performed with 6 μM of each tRNA and 1 μM purified recombinant E. coli TrmD over time. The results showed nearly 100% methylation of the transcript of E. coli tRNAPro(UGG) and nearly 70% methylation of the transcript of E. coli tRNAArg(CCG). (B) An example of a time course of the overall peptide-bond formation at the A site for E. coli tRNAPro(UGG) at the CCA codon assayed at the G37-state and at the m1G37-state. The reaction was performed under substrate-limiting conditions in an in vitro reconstituted translation system that encompasses all intermediate steps leading to peptidyl transfer from [35S]-fMet at the P site to the prolyl moiety at the A site. The synthesized [35S]-fMet-Pro was separated from [35S]-fMet by electrophoretic thin layer chromatography (eTLC). The migration positions of [35S]-fMet, [35S]-fMet-Pro, and the unformylated [35S]-Met were indicated. Consistent with all of our peptide-bond formation assays, [35S]-fMet migrated at two positions. The % of peptide-bond formation was calculated by the band intensity of the dipeptide over the total intensity of the dipeptide and both species of fMet. (C) A linear time course of synthesis of [35S]-fMet-Pro from [35S]-fMet based on the raw data in (B). The kinetic parameter kobs is shown for both the G37-state and the m1G37-state, with no major difference between the two at this anticodon-codon pair. (D) An example of a time course of the overall peptide-bond formation at the A site for E. coli tRNAArg(CCG) at the CGG codon assayed at the G37-state and at the m1G37-state. The reaction was performed under substrate-limiting conditions as in (B). The synthesized [35S]-fMet-Arg was separated from [35S]-fMet by eTLC. The migration positions of [35S]-fMet, [35S]-fMet-Arg, and the unformylated [35S]-Met were indicated. The % of peptide-bond formation was calculated by the band intensity of the dipeptide over the total intensity of the dipeptide and both species of fMet. (E) A linear time course of synthesis of [35S]-fMet-Arg from [35S]-fMet based on the raw data in (D). The kinetic parameter kobs is shown for both the G37-state and the m1G37-state, with a clear difference between the two at this anticodon-codon pair.
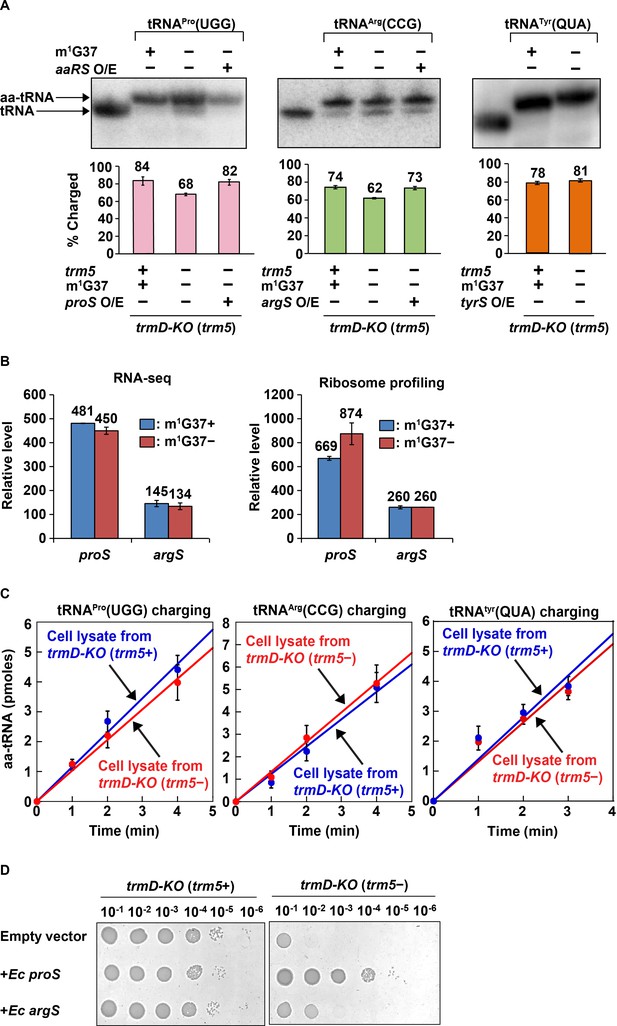
Acid-urea gel analysis of loss of tRNA aminoacylation in m1G37-deficient E. coli cells.
(A) Northern blots of acid-urea gels showing the fractional distribution of charged (aa-tRNA) vs. uncharged (tRNA) in total RNA prepared from trmD-KO cells complemented by the Ara-controlled trm5. Three E. coli tRNAs were probed: tRNAPro(UGG) (left panel), tRNAArg(CCG) (middle panel), and tRNATyr(QUA) (right panel), each under m1G37-abundant (+) and m1G37-deficient (–) conditions. Of the three, the m1G37-dependent tRNAPro(UGG) and tRNAArg(CCG) were further probed in m1G37-deficient cells with over-expression (O/E) of proS and argS, respectively. In contrast, the m1G37-independent tRNATyr(QUA) served as a control and was not probed for O/E of tyrS. The % of aa-tRNA in each case is calculated from the sum of the charged aminoacyl-tRNA (aa-tRNA) and the uncharged tRNA and is shown in the bar graph below for three biological replicates (n = 3). (B) Relative levels of expression of E. coli proS and argS in RNA-seq and ribosome-profiling analysis in m1G37+ and m1G37– conditions of two biological replicates. (C) Aminoacylation activity in E. coli cell lysates prepared in m1G37+ and m1G37– conditions. Three enzymes, encoded by proS (left panel), argS (middle panel), and tryS (right panel), were each tested against the cognate tRNA (i.e., m1G37-state tRNAPro(UGG), m1G37-state tRNAArg(CCG), and A37-state tRNATyr(QUA), respectively). Data are the average of three biological replicates (n = 3). Aminoacylation was performed under substrate-limiting conditions to obtain a linear line of product formation over time. (D) Viability of E. coli trmD-KO (trm5+) and trmD-KO (trm5–) cells harboring an empty vector, or the vector over-expressing E. coli proS or argS, was evaluated by spotting a serial dilution of cells onto an M9 plate with or without Ara for creating trmD-KO (trm5+) and trmD-KO (trm5–) conditions.
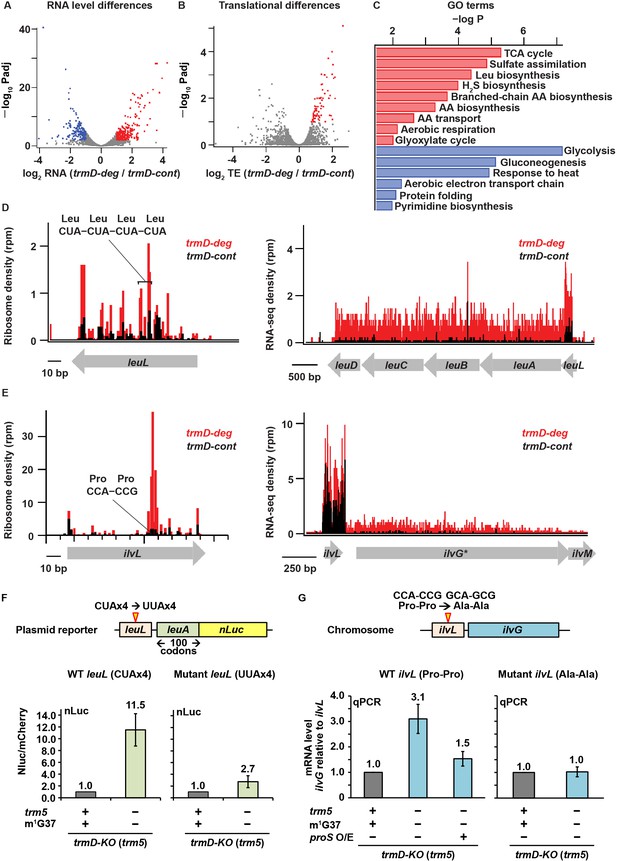
Changes in gene expression arising from m1G37 deficiency.
(A) Volcano plot showing differences in steady-state RNA levels (from RNA-seq data analyzed by DESeq) using samples of two replicates of trmD-deg and trmD-cont strains upon ClpXP induction. Genes that are more than 2-fold higher in the mutant with p < 0.01 are colored red (n = 220); those that are more than 2-fold lower with p < 0.01 are colored blue (n = 166). (B) Volcano plot showing differences in ribosome occupancy (Ribo-seq/RNA-seq analyzed by Xtail) using samples from two replicates of trmD-deg and trmD-cont strains upon ClpXP induction. Of these, 95 genes that are up-regulated (p < 0.1) are colored red. (C) Gene ontology categories for genes enriched in the up-regulated genes (red) and down-regulated genes (blue) from (A). (D) Gene model showing ribosome reads (in rpm) and RNA-seq density (in rpm, reads per million mapped reads) at the leuL leader sequence upstream of the leuABCD operon. (E) Gene model showing ribosome reads (in rpm) and RNA-seq density (in rpm) at the ilvL leader sequence upstream of the ilvGMEDA operon. (F) A plasmid reporter construct that demonstrates the codon-specific effect in the leuL leader sequence on expression of the downstream leuA gene. The plasmid reporter encodes the genomic sequence of leuL, followed by the first 100 codons of leuA, in the native sequence or with substitution of the four consecutive CUA codons with four UUA codons. The 100 codons of leuA are then fused to the nano-luciferase (nLuc) gene in-frame. Expression of nLuc, normalized by co-expression of mCherry in a separate plasmid within the same cell, is shown for m1G37+ and m1G37– conditions for the average of three biological replicates (n = 3). (G) Analysis of the CCA-CCG (Pro-Pro) codons in the genomic locus of ilvL as the determinant of regulation of gene expression of the downstream ilvG gene. A variant Escherichia coli strain was created that changed the CCA-CCG (Pro-Pro) codons to GCA-GCG (Ala-Ala) codons at the natural genomic locus, using CRISPR/Cas editing. Expression of ilvG was monitored by qPCR analysis in m1G37+ and m1G37– conditions and in m1G37– condition with over-expression of proS as shown as the average of three biological replicates (n = 3).
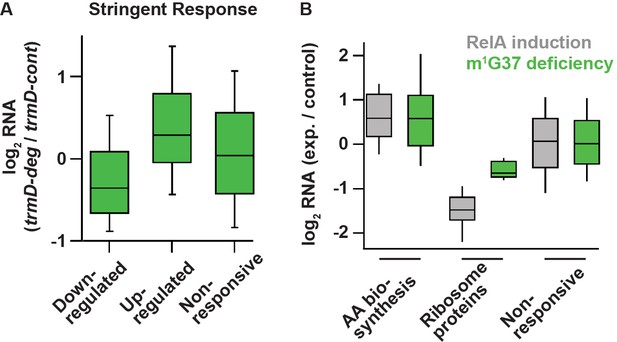
Deficiency of m1G37 induces gene expression similar to that of the bacterial stringent response.
(A) A set of genes published previously by Gourse et al. (Sanchez-Vazquez et al., 2019), whose steady-state RNA levels changed more than 2-fold upon induction of the stringent response by RelA over-expression (down n = 401 genes, up n = 321 genes, other = 3036 genes). Using these same sets of genes, the ratio of RNA levels in trmD-deg and trmD-cont strains upon ClpXP induction are shown. (B) Changes in expression in genes involved in amino acid biosynthesis (n = 89), genes encoding ribosomal proteins (n = 47), and other genes.
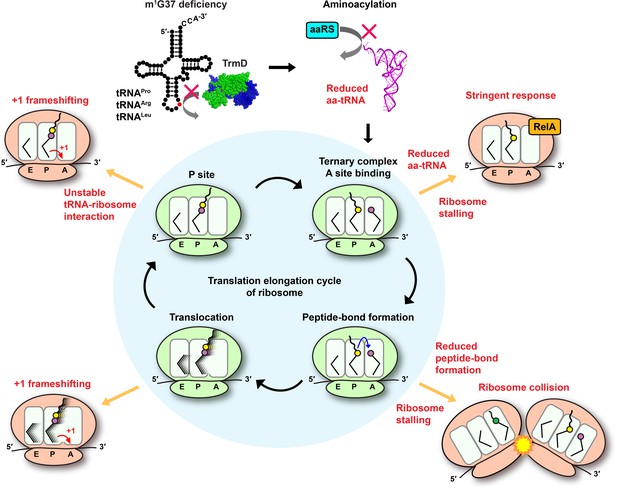
Deficiency of m1G37 impairs the elongation cycle of protein synthesis in bacteria.
A cloverleaf structure of tRNA, showing the position of m1G37 in red. Loss of m1G37 reduces aminoacylation, lowering levels of aa-tRNAs at the ribosome A site, stalling the ribosome, and activating programmatic changes in gene expression similar to those induced by the bacterial stringent response upon RelA sensing of uncharged tRNA and synthesis of ppGpp. Ribosome stalling at the A site is prolonged if the m1G37-deficient aa-tRNA is delayed in peptide-bond formation, leading to ribosome collision. Even if the m1G37-deficient tRNA manages to enter the A site and participates in peptide-bond formation, it would promote +1 frameshifting during translocation from the A site to the P site and during occupancy within the P site, while also destabilizing the P site structure and possibly falling off from the P site to prematurely terminate protein synthesis. Accumulation of these defects ultimately collapses the entire elongation cycle and leads to cell death.
Tables
Reagent type (species) or resource | Designation | Source or reference | Identifiers | Additional information |
---|---|---|---|---|
Strain, strain background (Escherichia coli) | G78 trmD-deg | This paper | TrmD is fused with a C-terminal YALAA degron tag | |
Strain, strain background (Escherichia coli) | G78 trmD-cont | This paper | Control strain without a degron tag | |
Strain, strain background (Escherichia coli) | MG1655 trmD-KO | Masuda et al., 2019 | ||
Strain, strain background (Escherichia coli) | MG1655 trmD-KO ilvL Ala-Ala mutant | This paper | CRISPR mutant possessing GCA-GCG codons replacing CCA-CCG codons on ilvL gene | |
Strain, strain background (Escherichia coli) | JM109 trmD-KO | Demo et al., 2021 | ||
Strain, strain background (Escherichia coli) | BL21(DE3) trmD-KO | Gamper et al., 2015a | ||
Strain, strain background (Escherichia coli) | BL21(DE3) | CGSC | CGSC#: 12504 | |
Antibody | anti-TrmD (Rabbit polyclonal) | Li and Björk, 1999 doi: 10.1017/S1355838299980834 | WB (1:10000) | |
Antibody | anti-rabbit IgG (Goat polyclonal) | Sigma-Aldrich | Cat#: A0545 | WB(1:160000) |
Peptide, recombinant protein | Proteinase K | Promega | Cat#: V302B | |
Peptide, recombinant protein | Micrococcal nuclease | Roche | Cat#: 10107921001 | |
Peptide, recombinant protein | Superscript III reverse transcriptase | Invitrogen | Cat#: 18080–044 | |
Peptide, recombinant protein | RQ1 RNase-free DNase | Promega | Cat#: M6101 | |
Commercial assay or kit | TruSeq Stranded Total RNA kit | Illumina | Cat#: 20020598 | |
Commercial assay or kit | SuperSignal West Pico Chemiluminescent Substrate | Thermo Fisher Scientific | Cat#: 34080 | |
Commercial assay or kit | NEBuilder HiFi DNA Assembly Cloning Kit | New England Biolabs | Cat#: E5520S | |
Commercial assay or kit | Nano-Glo Luciferase Assay System | Promega | Cat#: N1110 | |
Commercial assay or kit | TRIzol | Invitrogen | Cat#: 15596026 | |
Commercial assay or kit | Direct-zol RNA MiniPrep Kits | Zymo Research | Cat#: R2051 | |
Commercial assay or kit | RevertAid First Strand cDNA synthesis Kit | Thermo Fisher Scientific | Cat#: K1622 | |
Commercial assay or kit | SYBR Green I Master | Roche | Cat#: 04707516001 | |
Software, algorithm | Skewer | Jiang et al., 2014 | ||
Software, algorithm | bowtie | Langmead et al., 2009 | ||
Software, algorithm | DESeq | Love et al., 2014 | ||
Software, algorithm | Xtail | Xiao et al., 2016 | ||
Software, algorithm | DAVID | Huang et al., 2007 | ||
Software, algorithm | Pathway Tools | Karp et al., 2021 | ||
Software, algorithm | ImageQuant | GE Healthcare | ||
Software, algorithm | Kaleidagraph | Synergy software | ||
Software, algorithm | ImageJ | NIH | ||
Other | MOPS EZ Rich Defined | Teknova | Cat#: M2105 | Growth medium |
Additional files
-
Source data 1
Original image files and kinetic parameters.
- https://cdn.elifesciences.org/articles/70619/elife-70619-data1-v2.zip
-
Transparent reporting form
- https://cdn.elifesciences.org/articles/70619/elife-70619-transrepform-v2.docx