Inhibition of SARS-CoV-2 polymerase by nucleotide analogs from a single-molecule perspective
Figures
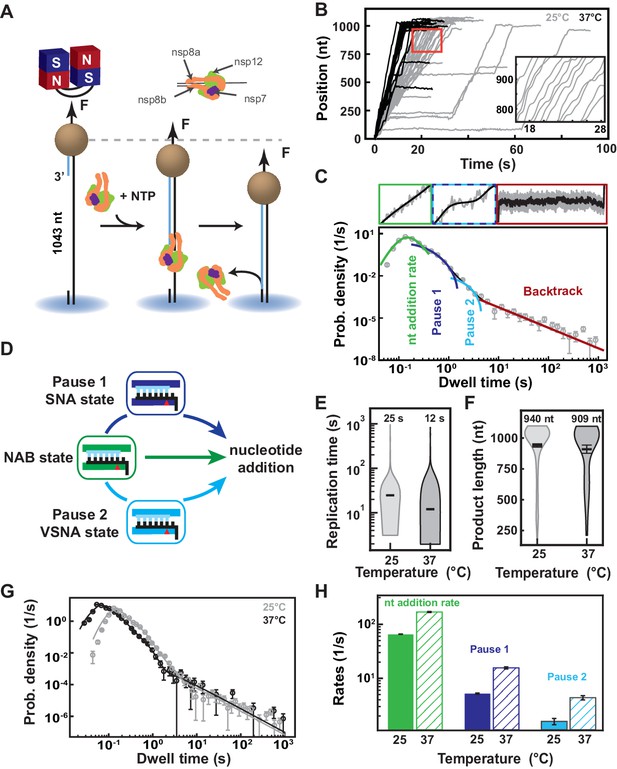
SARS-CoV-2 polymerase is a fast and processive RNA polymerase complex.
(A) Schematic of the magnetic tweezers assay to monitor RNA synthesis by the SARS-CoV-2 polymerase complex. A magnetic bead is attached to a glass coverslip surface by a 1043 long ssRNA construct that experiences a constant force F (35 pN if not mentioned otherwise). The polymerase, formed by nsp7, nsp8, and nsp12, assembles at the 3′-end of the RNA strand annealed to the template. The subsequent conversion of the ssRNA template into dsRNA reduces the end-to-end extension of the tether, signaling replication activity. (B) SARS-CoV-2 polymerase activity traces acquired at either 25°C (gray) or 37°C (black), showing bursts of nucleotide addition interrupted by pauses. The inset is a zoom-in of the traces captured in the red square. (C) The dwell times collected from (B) are assembled into a distribution that is fitted using a stochastic pausing model (see Materials and methods; solid lines). The model includes four different probability distribution functions that describe the event that kinetically dominates the dwell time: uninterrupted 10 nucleotide additions (green), exponentially distributed Pause 1 and Pause 2 (blue and cyan, respectively), and the power-law distributed backtrack (red). (D) The dwell time distribution in (C) is described by the viral RdRp kinetic model (adapted from Dulin et al., 2017). Fast nucleotide addition is achieved by the nucleotide addition burst (NAB) pathway with the nucleotide addition rate extracted from (C). Pause 1 and Pause 2 are the kinetic signatures of the slow and very slow nucleotide addition (SNA and VSNA, respectively) pathways, the latter being likely related to nucleotide mismatch incorporation. (E) Total replication time and (F) product length of SARS-CoV-2 polymerase activity traces at either 25°C or 37°C. The median total replication time and the mean product length are indicated above the violin plots, and represented as thick horizontal lines. The error bars represent one standard deviation extracted from 1000 bootstraps. (G) Dwell time distributions of SARS-CoV-2 polymerase activity traces at 25°C (gray circles) and 37°C (black circles) extracted from (B), and their respective fit to the stochastic-pausing model (corresponding solid lines). (H) Nucleotide addition rate (green), Pause 1 (dark blue), and Pause 2 (cyan) exit rates at either 25°C or 37°C (solid and hatched bars, respectively) extracted from (G). The error bars in (C and G) represent one standard deviation extracted from 1000 bootstraps. The error bars in (H) are one standard deviation extracted from 100 bootstraps.

Experimental conditions of SARS-CoV-2 polymerase high throughput magnetic tweezers experiments.
(A) SDS-PAGE analysis of SARS CoV-2 replicase proteins. Shown is a 15% polyacrylamide gel with 2 µg of purified nsp7 (9 kDa), nsp8 (22 kDa), and nsp12 (110 kDa). Broad-range molecular weight markers (Mr) and corresponding molecular weights are indicated. Gel was stained with Coomassie. (B) Schematic of the RNA hairpin construct assembled from hybridizing and ligating single-stranded RNAs. (C) Force as a function of the extension of the RNA hairpin is presented in (A). Increasing and decreasing force ramp represented in gray and black, respectively. The red arrow indicates the extension at 35 pN, the force applied in the measurements unless specified. (D) Force as a function of the extension of the RNA hairpin after conversion into dsRNA. Increasing and decreasing force ramp represented in gray and black, respectively. (E) Typical field of view containing ~450 hairpin tethered magnetic beads in a high throughput magnetic tweezers assay.
-
Figure 1—figure supplement 1—source data 1
Source image for the SDS-PAGE gel in Figure 1—figure supplement 2A.
- https://cdn.elifesciences.org/articles/70968/elife-70968-fig1-figsupp1-data1-v1.zip
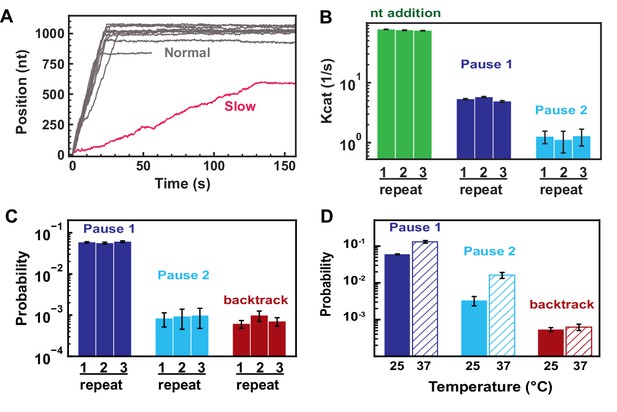
Selection of SARS-CoV-2 polymerase elongation traces and reproducibility.
(A) SARS-CoV-2 polymerase activity traces acquired at 35 pN and 500 µM NTPs. Slow traces (<0.5% of the total number of events) are discarded from subsequent analysis. (B) Nucleotide addition rate (green), Pause 1 (dark blue), and Pause 2 (cyan) exit rates for triplicate experiment acquired at 25 pN and 500 µM NTP. (C) Probabilities to enter Pause 1 (dark blue), Pause 2 (cyan), and the backtrack (red) states for triplicate experiment acquired as in (B). (D) Probabilities to enter Pause 1 (dark blue), Pause 2 (cyan), and the backtrack (red) states at either 25°C (solid bars) or 37°C (hatched bars). Error bars in (E, F, D) are one standard deviation extracted from 100 bootstraps.
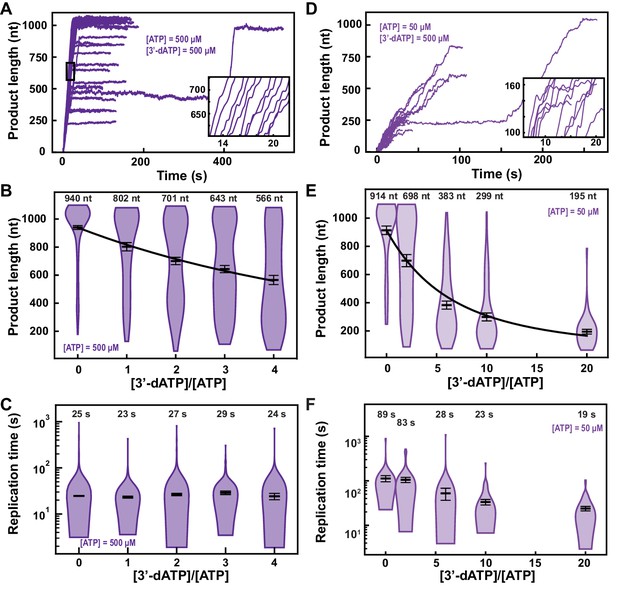
3′-dATP is an effective chain terminator for the SARS-CoV-2 polymerase.
(A) SARS-CoV-2 replication traces for 500 µM NTPs and 500 µM 3′-dATP; and (D), for 50 µM ATP, 500 µM all other NTPs and 500 µM 3′-dATP. (B, E) SARS-CoV-2 polymerase product length for the 1043 nt long template using the indicated concentration of ATP, 500 µM of other NTPs, as a function of [3′-dATP]/[ATP]. The mean values are indicated above the violin plots, and represented by horizontal black thick lines flanked by one standard deviation error bars extracted from 1000 bootstraps. (C, F) Replication time for the reaction conditions described in (B, E). The medians are indicated above the violin plots, and represented by horizontal black thick lines flanked by one standard deviation error bars extracted from 1000 bootstraps. In (B, E), the solid lines are the fits of the terminator effective incorporation rate (see Materials and methods). In (A, D), the insets are zoom-in of the replication traces captured in the black square.

SARS-CoV-2 polymerase activity traces kinetics in presence of 3′-dATP.
(A) Dwell time distributions of SARS-CoV-2 polymerase activity traces acquired in the presence of 500 µM NTP either without (circles) or with 0.5 mM (triangles), 1 mM (squares), 1.5 mM (diamonds), and 2 mM (pentagons) 3′-dATP. The color code from light to dark gray further highlights the increasing concentration of 3′-dATP. The solid lines represent the fit to the pause-stochastic model. (B) Nucleotide addition rate (green), Pause 1 (dark blue), and Pause 2 (cyan) exit rates for the conditions described in (A). (C) Probabilities to enter Pause 1 (dark blue), Pause 2 (cyan), and the backtrack (red) states for the conditions described in (A). (D) Dwell time distributions of SARS-CoV-2 polymerase activity traces acquired in the presence of 50 µM ATP, 500 µM of other NTPs, and either without (circles), or with 0.1 mM (triangles), 0.3 mM (squares), 0.5 mM (diamonds), and 1 mM (pentagons) 3′-dATP. The color code from light to dark gray further highlights the increasing concentration of 3′-dATP. The solid lines represent the fit of the pause-stochastic model. (E) Nucleotide addition rate (green), Pause 1 (dark blue), and Pause 2 (cyan) exit rates for the conditions described in (D). (F) Probabilities to enter Pause 1 (dark blue), Pause 2 (cyan), and the backtrack (red) states for the conditions described in (D). The error bars in (A, D) represent one standard deviation extracted from 1000 bootstraps and the error bars in (B, C, E, F) represent one standard deviation extracted from 100 bootstraps.

Probabilistic model describing the competition for incorporation of a nucleic acid chain terminator NA and natural nucleotide.
From the empty active site state (E), either a terminator (T) or a natural nucleotide (N) can bind through direct competition with the first order binding rates and (solid arrows represent rates), respectively. From the bound states (Tb/Nb), there can be many sub-steps before either incorporating the base with probability or ejecting it from the active site with probability and (dashed arrows represent probabilities).
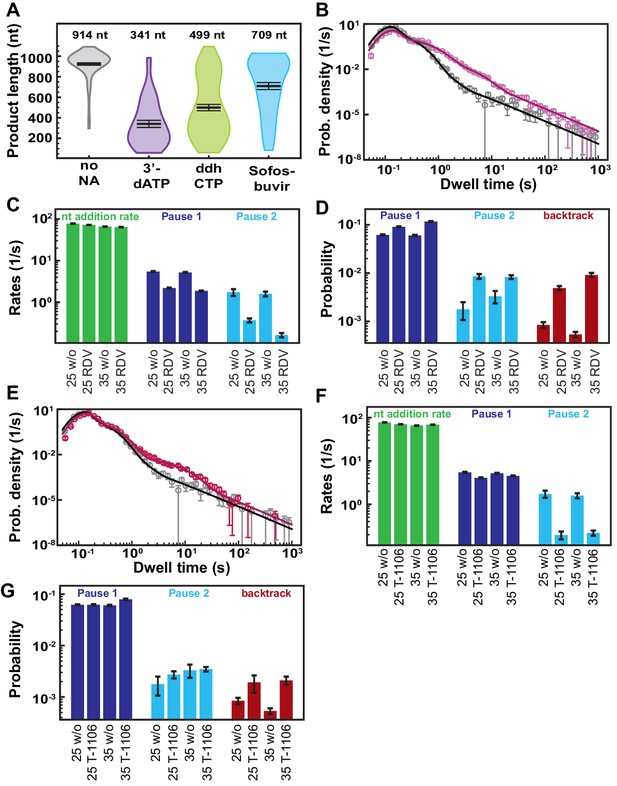
Decreasing the applied tension does not change the effect of nucleotide analogs (NAs) on the SARS-CoV-2 polymerase elongation.
All measurements are done at 25°C. (A) Product length of SARS-CoV-2 polymerase at 50 µM ATP and 500 µM all other NTPs (gray) or 300 µM NA and 50 µM of the competing NTP (purple: 3′-dATP, green: ddhCTP, and blue: Sofosbuvir-TP). The mean values are indicated above the violin plots, and represented by horizontal black thick lines flanked by one standard deviation error bars extracted from 1000 bootstraps. (B) Dwell time distributions of SARS-CoV-2 polymerase activity traces for 500 µM all NTPs without (gray) or with 100 µM RDV-TP (pink) at 25 pN. The corresponding solid lines are the fit to the pause-stochastic model. (C) Nucleotide addition rate (green), Pause 1 (dark blue), and Pause 2 (cyan) exit rates for the conditions described in (B). (D) Probabilities to enter Pause 1 (dark blue), Pause 2 (cyan), and the backtrack (red) states for the conditions described in (B) and Figure 3D. (E) Dwell time distributions of SARS-CoV-2 polymerase activity traces for 500 µM all other NTPs without (gray) or with 300 µM T1106-TP (red). The corresponding solid lines are the fit to the pause-stochastic model. (F) Nucleotide addition rate (green), Pause 1 (dark blue), and Pause 2 (cyan) exit rates for the conditions described in (E). (G) Probabilities to enter Pause 1 (dark blue), Pause 2 (cyan), and the backtrack (red) states for the conditions described in (E) and Figure 4D. The error bars in (B, E) represent one standard deviation extracted from 1000 bootstraps and the error bars in (C, E, F, G) represent one standard deviation extracted from 100 bootstraps.
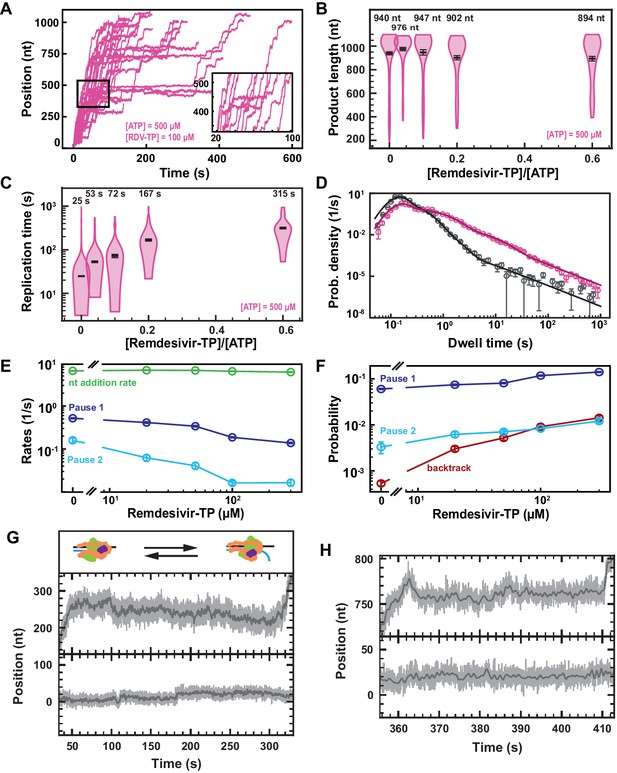
Remdesivir-TP (RDV-TP) is not a chain terminator but induces long-lived SARS-CoV-2 polymerase backtrack.
(A) SARS-CoV-2 polymerase activity traces for 500 µM NTPs and 100 µM RDV-TP. The inset is a zoom-in of the polymerase activity traces captured in the black square. (B) SARS-CoV-2 polymerase product length for the 1043 nt long template using the indicated concentration of ATP, 500 µM of other NTPs, as a function of [RDV-TP]/[ATP]. The mean values are indicated above the violin plots, and represented by horizontal black thick lines flanked by one standard deviation error bars extracted from 1000 bootstraps. (C) Replication time for the reaction conditions described in (B). The median values are indicated above the violin plots, and represented by horizontal black thick lines flanked by one standard deviation error bars extracted from 1000 bootstraps. (D) Dwell time distributions of SARS-CoV-2 polymerase activity traces for 500 µM NTPs in the absence (gray) or presence of 100 µM RDV-TP (pink). The corresponding solid lines are the fit of the stochastic-pausing model. (E) Nucleotide addition rate (green), Pause 1 (dark blue), and Pause 2 (cyan) exit rates for [NTPs]=500 µM and several RDV-TP concentrations. (F) Probabilities to enter Pause 1 (dark blue), Pause 2 (cyan), and the backtrack (red) states for the conditions described in (E). The error bars in (D) represent one standard deviation extracted from 1000 bootstraps and the error bars in (E, F) represent one standard deviation extracted from 100 bootstrap procedures. (G, H) Examples of deep SARS-CoV-2 backtracks induced by RDV-TP incorporation (top) and traces showing no polymerase activity (bottom). Traces acquired using ultra-stable magnetic tweezers as described in Bera et al., 2021 at 35 pN, 58 Hz acquisition frequency (gray), low-pass filtered at 1 Hz (dark gray), and using a SARS-CoV-2 polymerase reaction buffer containing 10 µM RDV-TP, 50 µM ATP, and 500 µM all other NTPs. The insert in (G) shows a schematic of backtracking polymerase.

SARS-CoV-2 polymerase elongation traces in presence of RDV-TP at 25°C.
(A) Examples of deep SARS-CoV-2 backtracks induced by RDV-TP incorporation (top) and traces showing no polymerase activity (bottom). Traces acquired using ultra-stable magnetic tweezers as described in Bera et al., 2021 at 35 pN, 58 Hz acquisition frequency (gray), low-pass filtered at 1 Hz (dark gray), and using a reaction buffer containing 10 µM RDV-TP, 50 µM ATP, and 500 µM all other NTPs. (B) Dwell time distributions of SARS-CoV-2 polymerase activity traces acquired in the presence of 500 µM NTP at 25°C, either without (circles), or with 20 µM (triangles), 50 µM (squares), 100 µM (diamonds), and 300 µM (pentagons) RDV-TP. The color code from light to dark gray further highlights the increasing concentration of RDV-TP. The solid lines represent the fit of the stochastic-pausing model. The error bars represent one standard deviation extracted from 1000 bootstraps.
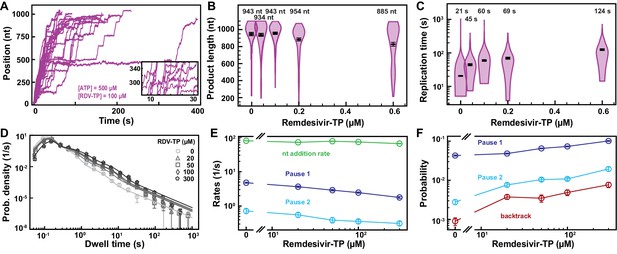
SARS-CoV-1 polymerase activity traces kinetics in presence of RDV-TP.
(A) SARS-CoV-1 polymerase activity traces for 500 µM NTPs and 100 µM RDV-TP. (B) SARS-CoV-1 polymerase product length for the 1043 nt long template using 500 µM NTPs, as a function of [RDV-TP]/[ATP]. The mean values are indicated above the violin plots, and represented by horizontal black thick lines flanked by one standard deviation error bars extracted from 1000 bootstraps. (C) Replication time for the reaction conditions described in (B). The median values are indicated above the violin plots, and represented by horizontal black thick lines flanked by one standard deviation error bars extracted from 1000 bootstraps. (D) Dwell time distributions of SARS-CoV-1 polymerase activity traces acquired in the presence of 500 µM NTP, either without (circles), or with 20 µM (triangles), 50 µM (squares), 100 µM (diamonds), and 300 µM (pentagons) RDV-TP. The color code from light to dark gray further highlights the increasing concentration of RDV-TP. The solid lines represent the fit of the stochastic-pausing model. (E) Nucleotide addition rate (green), Pause 1 (dark blue), and Pause 2 (cyan) exit rates from the fit of the dwell time distributions in (B). (F) Probabilities to enter Pause 1 (dark blue), Pause 2 (cyan), and the backtrack (red) states for the conditions described in (B). The error bars in (D) represent one standard deviation extracted from 1000 bootstraps and the error bars in (E, F) represent one standard deviation extracted from 100 bootstraps.
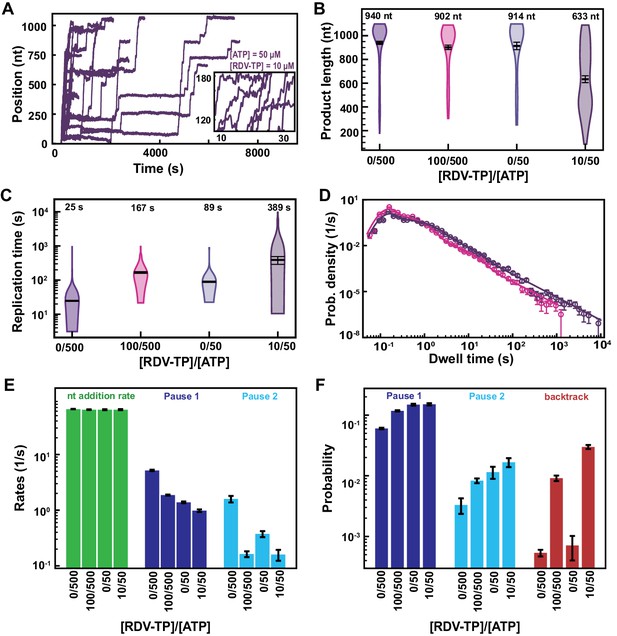
Lower ATP concentration at constant RDV-TP:ATP stoichiometry increases the effects of RDV-TP on SARS-CoV-2 polymerase elongation kinetics.
(A) SARS-CoV-2 polymerase activity traces for 10 µM RDV-TP with 50 µM ATP and 500 µM of the other NTPs. (B) Product length of SARS-CoV-2 polymerase at RDV-TP:ATP stoichiometry of 0/500, 100/500, 0/50, and 10/50. The mean values are indicated above the violin plots, and represented by horizontal black thick lines flanked by one standard deviation error bars extracted from 1000 bootstraps. (C) The replication times for the conditions described in (B). The median values are indicated above the violin plots, and represented by horizontal black thick lines flanked by one standard deviation extracted from 1000 bootstraps. (D) Dwell time distributions of SARS-CoV-2 polymerase activity traces for either 50 µM ATP, 500 µM all other NTPs and 10 µM RDV-TP (purple), or at 500 µM all NTPs and 100 µM RDV-TP (pink). The corresponding solid lines are the fit to the stochastic-pausing model. (E) Nucleotide addition rate (green), Pause 1 (dark blue), and Pause 2 (cyan) exit rates for the conditions described in (B). (F) Probabilities to enter Pause 1 (dark blue), Pause 2 (cyan), and the backtrack (red) states for the conditions described in (B). The error bars in (D) represent one standard deviation extracted from 1000 bootstraps and the error bars in (E, F) represent one standard deviation extracted from 100 bootstraps.

SARS-CoV-2 polymerase activity traces kinetics in presence of RDV-TP at 37°C.
(A) Dwell time distributions of SARS-CoV-2 replication activity acquired in the presence of 500 µM NTP at 37°C without (gray) and with 100 µM RDV-TP (pink). The solid lines represent the fit of the stochastic-pausing model. (B) Nucleotide addition rate (green), Pause 1 (dark blue), and Pause 2 (cyan) exit rates from the fit of the dwell time distributions in (A). (C) Probabilities to enter Pause 1 (dark blue), Pause 2 (cyan), and the backtrack (red) states for the conditions described in (A). The error bars in (A) represent one standard deviation extracted from 1000 bootstraps and the error bars in (B, C) represent one standard deviation extracted from 100 bootstraps.
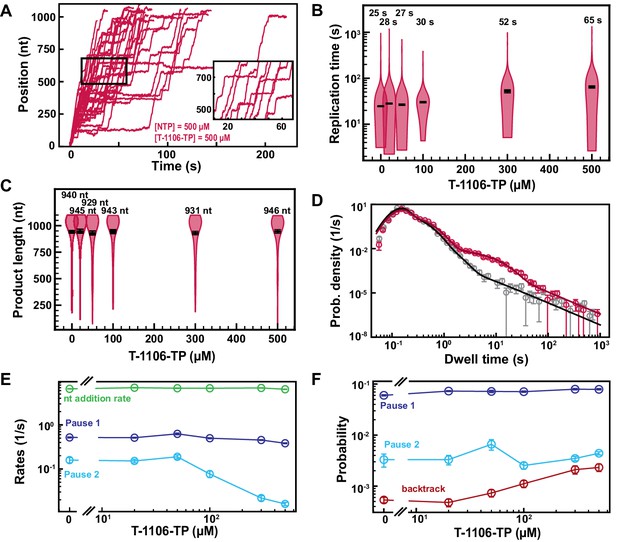
T-1106-TP incorporation induces pauses of intermediate duration and backtrack.
(A) SARS-CoV-2 polymerase activity traces in the presence of 500 µM NTPs, in the presence of 500 µM T-1106-TP. The inset is a zoom-in of the polymerase activity traces captured in the black square. (B) SARS-CoV-2 replication time for the 1043 nt long template using 500 µM of all NTPs, and the indicated concentration of T-1106-TP. The median values are indicated above the violin plots, and represented by horizontal black thick lines flanked by one standard deviation error bars extracted from 1000 bootstraps. (C) SARS-CoV-2 polymerase product length using 500 µM NTPs and the indicated concentration of T-1106-TP. The mean values are indicated above the violin plots, and represented by horizontal black thick lines flanked by one standard deviation error bars extracted from 1000 bootstraps. (D) Dwell time distributions of SARS-CoV-2 polymerase activity traces for 500 µM NTP either without (gray) or with 500 µM (red) T-1106-TP. The corresponding solid lines are the fit to the stochastic-pausing model. (E) Nucleotide addition rate (green), Pause 1 (dark blue), and Pause 2 (cyan) exit rates for [NTPs]=500 µM and several T-1106-TP concentrations. (F) Probabilities to enter Pause 1 (dark blue), Pause 2 (cyan), and the backtrack (red) states for the conditions described in (E). The error bars denote one standard deviation from 1000 bootstraps in (D) and the error bars in (E, F) denote one standard deviation extracted from 100 bootstrap procedures.
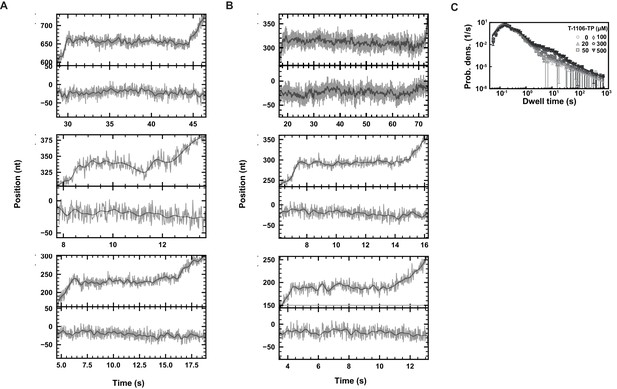
SARS-CoV-2 polymerase elongation in presence of T-1106-TP.
Using an ultra-stable magnetic tweezers configuration, we monitored pauses in SARS-CoV-2 polymerase activity traces at 58 Hz camera acquisition frequency (gray; 1 Hz low-pass filtered: dark gray), applying 35 pN force, and in the presence of 500 µM T-1106-TP and 500 µM all NTPs. Top: zoom-in the polymerase activity traces; bottom inactive tether followed simultaneously. (A) Some pauses demonstrate shallow backtracks, (B) while in other pause cases, polymerase backtrack is difficult to confirm given the spatiotemporal resolution of the assay. (C) Dwell time distributions of SARS-CoV-2 polymerase activity traces acquired in the presence of 500 µM NTP, either without (circles), or with 20 µM (triangles), 50 µM (squares), 100 µM (diamonds), 300 µM (pentagons), and 500 µM (upside down triangle) T-1106-TP. The color code from light to dark gray further highlights the increasing concentration of T-1106-TP. The solid lines represent the fit of the stochastic-pausing model. The error bars represent one standard deviation extracted from 1000 bootstraps.
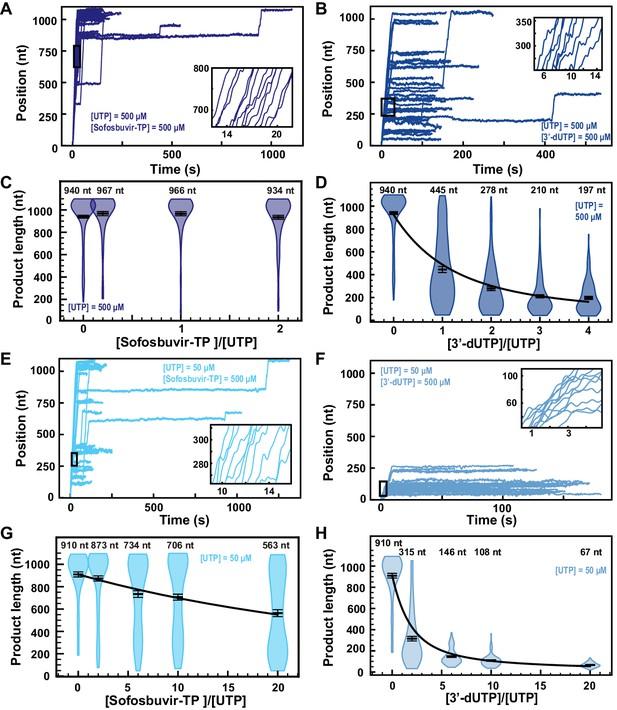
Sofosbuvir-TP is a poor SARS-CoV-2 polymerase inhibitor in contrast with 3′-dUTP.
(A, B) SARS-CoV-2 polymerase activity traces for 500 µM NTPs and 500 µM of either (A) Sofosbuvir-TP or (B) 3′-dUTP. (C, D) SARS-CoV-2 polymerase product length using the indicated concentration of UTP, 500 µM of other NTPs, as a function of either (C) [Sofosbuvir-TP]/[UTP] or (D) [3′-dUTP]/[UTP]. The mean values are indicated above the violin plots, and represented by horizontal black thick lines flanked by one standard deviation error bars extracted from 1000 bootstraps. (E, F) SARS-CoV-2 polymerase activity traces in the presence of 50 µM of UTP, 500 µM of all other NTPs, and 500 µM of either (E) Sofosbuvir-TP or (F) 3′-dUTP. (G, H) SARS-CoV-2 polymerase product length using 50 µM UTP, 500 µM of other NTPs, as a function of either (G) [Sofosbuvir-TP]/[UTP] or (H) [3′-dUTP]/[UTP]. The mean values are indicated above the violin plots, and represented by horizontal black thick lines flanked by one standard deviation error bars extracted from 1000 bootstraps. In (D, G, H), the solid line is the fit of the terminator effective incorporation rate (see Materials and methods). In (A, B, E, F), the insets are a zoom-in of the replication traces captured in the black square.
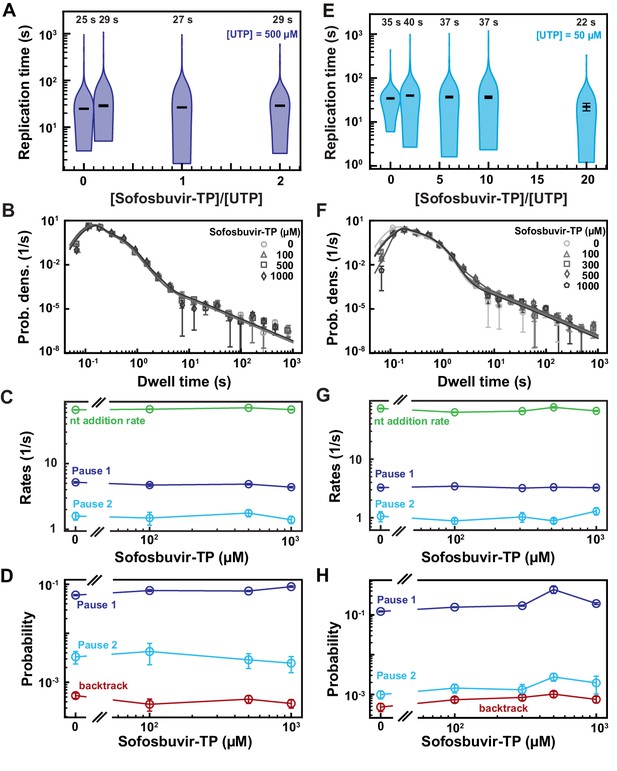
SARS-CoV-2 polymerase activity traces kinetics in presence of Sofosbuvir-TP.
(A, E) SARS-CoV-2 replication time for the 1043 nt long template using the indicated concentration of UTP, 500 µM of other NTPs as a function of the stoichiometry of [Sofosbuvir-TP]/[UTP]. The median values are indicated above the violin plots, and represented by horizontal black thick lines flanked by one standard deviation extracted from 1000 bootstraps. (B) Dwell time distributions of SARS-CoV-2 polymerase activity traces acquired in the presence of 500 µM NTP either without (circles) or with 0.1 mM (triangles), 0.5 mM (squares), and 1 mM (diamonds) Sofosbuvir-TP. The color code from light to dark gray further highlights the increasing concentration of Sofosbuvir-TP. The solid lines represent the fit to the stochastic-pausing model. (C) Nucleotide addition rate (green), Pause 1 (dark blue), and Pause 2 (cyan) exit rates for the conditions described in (B). (D) Probabilities to enter Pause 1 (dark blue), Pause 2 (cyan), and the backtrack (red) states for the conditions described in (B). (F) Dwell time distributions of SARS-CoV-2 polymerase activity traces acquired in the presence of 50 µM UTP, 500 µM of other NTPs, and either without (circles), or with 0.1 mM (triangles), 0.3 mM (squares), 0.5 mM (diamonds), and 1 mM (pentagons) Sofosbuvir-TP. The color code from light to dark gray further highlights the increasing concentration of Sofosbuvir-TP. The solid lines represent the fit to the stochastic-pausing model. (G) Nucleotide addition rate (green), Pause 1 (dark blue), and Pause 2 (cyan) exit rates for the conditions described in (F). (H) Probabilities to enter Pause 1 (dark blue), Pause 2 (cyan), and the backtrack (red) states for the conditions described in (F). The error bars in (B, F) represent one standard deviation extracted from 1000 bootstraps and the error bars in (C, D, G, H) represent one standard deviation extracted from 100 bootstraps.
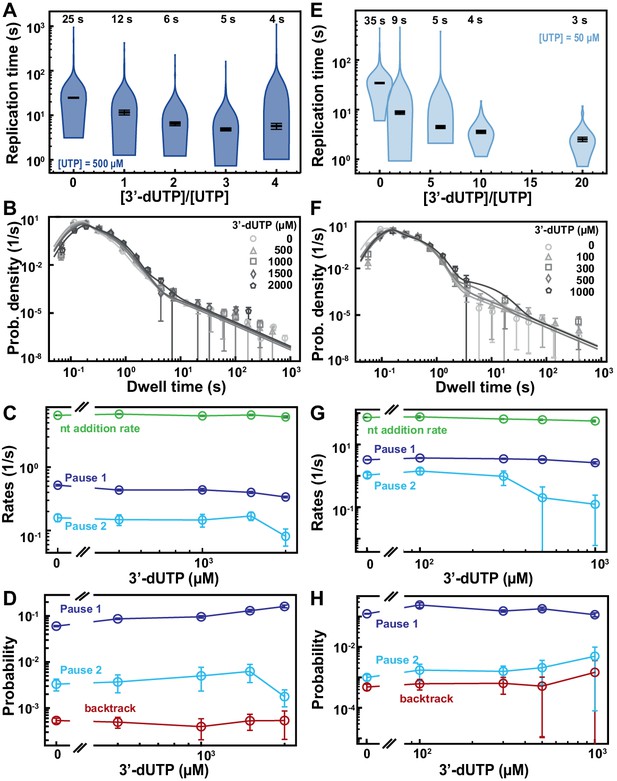
SARS-CoV-2 polymerase activity traces kinetics in presence of 3′-dUTP.
(A, E) SARS-CoV-2 replication time for the 1043 nt long template using the indicated concentration of UTP, 500 µM of other NTPs as a function of the stoichiometry of [3′-dUTP]/[UTP]. The median values are indicated above the violin plots, and represented by horizontal black thick lines flanked by one standard deviation extracted from 1000 bootstraps. (B) Dwell time distributions of SARS-CoV-2 polymerase activity traces acquired in the presence of 500 µM NTP either without (circles) or with 0.5 mM (triangles), 1 mM (squares), 1.5 mM (diamonds), and 2 mM (pentagon) 3′-dUTP. The color code from light to dark gray further highlights the increasing concentration of 3′-dUTP. The solid lines represent the fit to the stochastic-pausing model. (C) Nucleotide addition rate (green), Pause 1 (dark blue), and Pause 2 (cyan) exit rates for the conditions described in (B). (D) Probabilities to enter Pause 1 (dark blue), Pause 2 (cyan), and the backtrack (red) states for the conditions described in (B). (F) Dwell time distributions of SARS-CoV-2 polymerase activity traces acquired in the presence of 50 µM UTP, 500 µM of other NTPs, and either without (circles), or with 0.1 mM (triangles), 0.3 mM (squares), 0.5 mM (diamonds), and 1 mM (pentagons) 3′-dUTP. The color code from light to dark gray further highlights the increasing concentration of 3′-dUTP. The solid lines represent the fit to the stochastic-pausing model. (G) Nucleotide addition rate (green), Pause 1 (dark blue), and Pause 2 (cyan) exit rates for the conditions described in (F). (H) Probabilities to enter Pause 1 (dark blue), Pause 2 (cyan), and the backtrack (red) states for the conditions described in (F). The error bars in (B, F) represent one standard deviation extracted from 1000 bootstraps and the error bars in (C, D, G, H) represent one standard deviation extracted from 100 bootstraps.
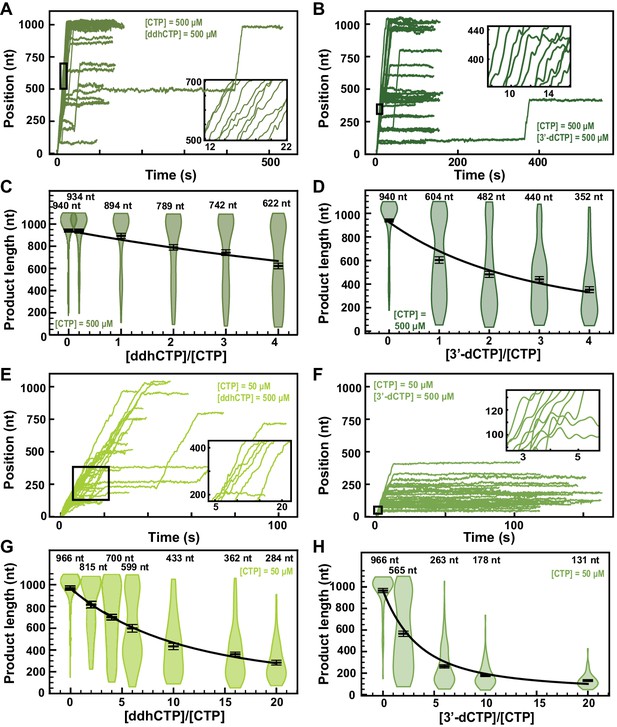
ddhCTP and 3ʹ-dCTP inhibit efficiently the SARS-CoV-2 polymerase.
(A, B) SARS-CoV-2 polymerase activity traces for 500 µM NTPs and 500 µM of either (A) ddhCTP or (B) 3ʹ-dCTP. (C, D) SARS-CoV-2 polymerase product length using the indicated concentration of CTP, 500 µM of other NTPs, as a function of either (C) [ddhCTP]/[CTP] or (D) [3ʹ-dCTP]/[CTP]. The mean values are indicated above the violin plots, and represented by horizontal black thick lines flanked by one standard deviation error bars extracted from 1000 bootstraps. (E, F) SARS-CoV-2 polymerase activity traces in the presence of 50 µM of CTP, 500 µM of all other NTPs, and 500 µM of either (E) ddhCTP or (F) 3ʹ-dCTP. (G, H) SARS-CoV-2 polymerase activity traces product length using 50 µM CTP, 500 µM of other NTPs, as a function of the stoichiometry of either (G) [ddhCTP]/[CTP] or (H) [3ʹ-dCTP]/[CTP]. The mean values are indicated above the violin plots, and represented by horizontal black thick lines flanked by one standard deviation error bars extracted from 1000 bootstraps. In (C, D, G, H), the solid lines are the fits of the terminator effective incorporation rate (see Materials and methods). In (A, B, E, F), the insets are a zoom-in of the replication traces captured in the black square.
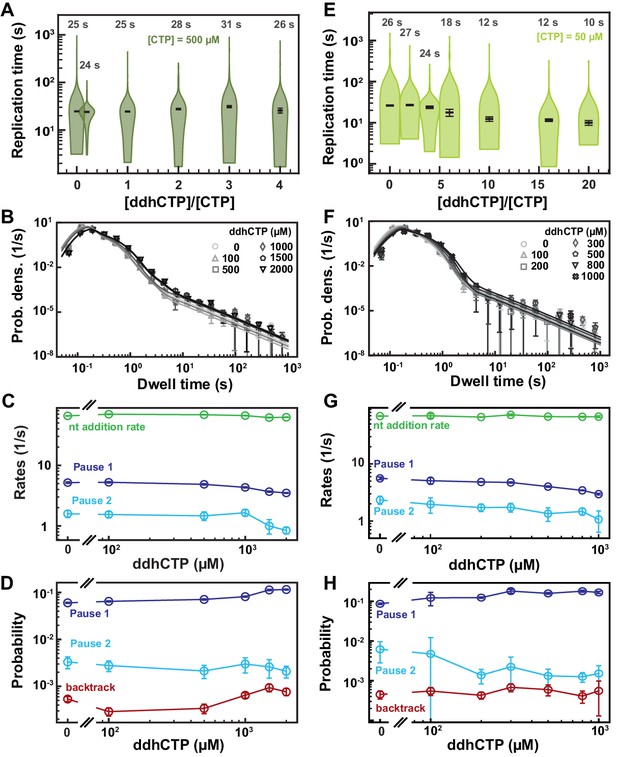
SARS-CoV-2 polymerase activity traces in presence of ddhCTP.
(A, E) SARS-CoV-2 replication time for the 1043 nt long template using the indicated concentration of CTP, 500 µM of other NTPs, and the indicated stoichiometry of [ddhCTP]/[CTP]. The median values are indicated above the violin plots, and represented by horizontal black thick lines flanked by one standard deviation extracted from 1000 bootstraps. (B) Dwell time distributions of SARS-CoV-2 polymerase activity traces acquired in the presence of 500 µM NTP either without (circles) or with 0.1 mM (triangles), 0.5 mM (squares), and 1 mM (diamonds) ddhCTP. The color code from light to dark gray further highlights the increasing concentration of ddhCTP. The solid lines represent the fit to the stochastic-pausing model. (C) Nucleotide addition rate (green), Pause 1 (dark blue), and Pause 2 (cyan) exit rates for the conditions described in (B). (D) Probabilities to enter Pause 1 (dark blue), Pause 2 (cyan), and the backtrack (red) states for the conditions described in (B). (F) Dwell time distributions of SARS-CoV-2 polymerase activity traces acquired in the presence of 50 µM CTP, 500 µM of other NTPs, and either without (circles), or with 0.1 mM (triangles), 0.2 mM (squares), 0.3 mM (diamonds), 0.5 mM (pentagons), 0.8 mM (upside down triangle), and 1 mM (x) ddhCTP. The color code from light to dark gray further highlights the increasing concentration of ddhCTP. The solid lines represent the fit to the stochastic-pausing model. (G) Nucleotide addition rate (green), Pause 1 (dark blue), and Pause 2 (cyan) exit rates for the conditions described in (F). (H) Probabilities to enter Pause 1 (dark blue), Pause 2 (cyan), and the backtrack (red) states for the conditions described in (F). The error bars in (B, F) represent one standard deviation extracted from 1000 bootstraps and the error bars in (C, D, G, H) represent one standard deviation extracted from 100 bootstraps.

SARS-CoV-2 polymerase activity traces kinetics in presence of 3′-dCTP.
(A, E) SARS-CoV-2 replication time for the 1043 nt long template using the indicated concentration of CTP, 500 µM of other NTPs as a function of the stoichiometry of [3′-dCTP]/[CTP]. The median values are indicated above the violin plots, and represented by horizontal black thick lines flanked by one standard deviation extracted from 1000 bootstraps. (B) Dwell time distributions of SARS-CoV-2 polymerase activity traces acquired in the presence of 500 µM NTP either without (circles) or with 0.5 mM (triangles), 1 mM (squares), 1.5 mM (diamonds), and 2 mM (pentagon) 3′-dCTP. The color code from light to dark gray further highlights the increasing concentration of 3′-dCTP. The solid lines represent the fit to the stochastic-pausing model. (C) Nucleotide addition rate (green), Pause 1 (dark blue), and Pause 2 (cyan) exit rates for the conditions described in (B). (D) Probabilities to enter Pause 1 (dark blue), Pause 2 (cyan), and the backtrack (red) states for the conditions described in (B). (F) Dwell time distributions of SARS-CoV-2 polymerase activity traces acquired in the presence of 50 µM CTP, 500 µM of other NTPs, and either without (circles), or with 0.1 mM (triangles), 0.3 mM (squares), 0.5 mM (diamonds), and 1 mM (pentagons) 3′-dCTP. The color code from light to dark gray further highlights the increasing concentration of 3′-dCTP. The solid lines represent the fit to the stochastic-pausingmodel. (G) Nucleotide addition rate (green), Pause 1 (dark blue), and Pause 2 (cyan) exit rates for the conditions described in (F). (H) Probabilities to enter Pause 1 (dark blue), Pause 2 (cyan), and the backtrack (red) states for the conditions described in (F). The error bars in (B, F) represent one standard deviation extracted from 1000 bootstraps and the error bars in (C, D, G, H) represent one standard deviation extracted from 100 bootstraps.

ddhC does not inhibit SARS-CoV-2 replication in huh7-hACE2 cells.
Huh7-hACE2 cells in 96-well were incubated with the indicated concentrations of the tested compounds for 1.5 hr before SARS-CoV-2 (USA-WA1/2020 isolate) was added at MOI of 0.05. At ~24 hpi, the percentage of infected cells was assessed by immunofluorescence assay using a rabbit monoclonal antibody against the SARS-CoV-2 N protein. ‘sofo’ is Sofosbuvir. Results in (A and B) represent two separate experiments.
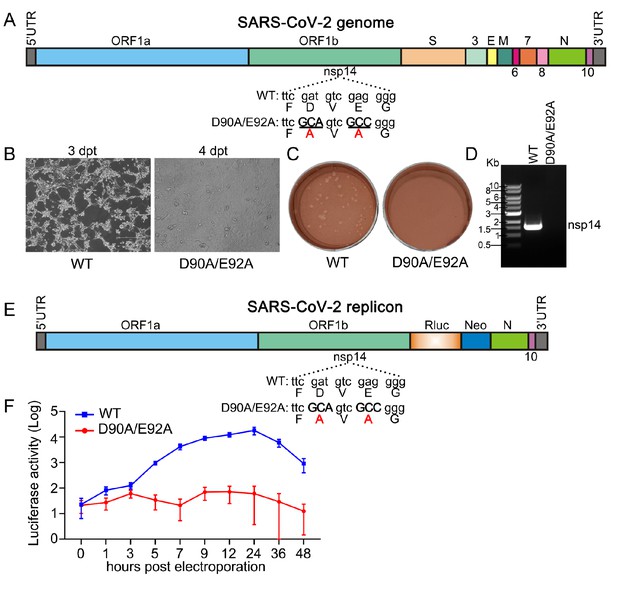
SARS-CoV-2 nsp14 exoribonuclease knockout is not replicative.
(A) SARS-CoV-2 genome. The SARS-CoV-2 nsp14 exoribonuclease nucleotides and amino acid mutations (D90A/E92A) are indicated. (B) Phase-contrast images of electroporated cells. Vero E6 cells were electroporated with SARS-CoV-2 WT or nsp14 exoribonuclease knockout mutant RNA. (C) Plaque morphology of SARS-CoV-2 WT and nsp14 exoribonuclease knockout mutant viruses. Supernatants were harvested on day 3 post-electroporation (WT) and day 4 post-electroporation (mutant) from (B). Plaque assay was performed in Vero E6 cells and staining with neutral red solution after 48 hr infection. (D) RT-PCR analysis. Extracellular RNA from (B) were harvested on day 3 (WT) and day 4 (mutant). The nsp14 region of SARS-CoV-2 was amplified by RT-PCR to confirm viral production. Figure 6—figure supplement 4—source data 1 is the original of the agarose gel. (E) Transient replicon of SARS-CoV-2. A Renilla luciferase gene is inserted as a reporter and the nsp14 exoribonuclease knockout is shown as indicated. (F) Replicon luciferase assay. Huh-7 cells were electroporated with WT or mutant replicon RNA, cells were harvested and assayed for luciferase activities at indicated timepoints.
-
Figure 6—figure supplement 4—source data 1
Source image for the agarose gel in Figure 6—figure supplement 4D.
- https://cdn.elifesciences.org/articles/70968/elife-70968-fig6-figsupp4-data1-v1.zip
Tables
Summary table for the investigated NAs.
Modification | Incorporation pathway | Mechanism of action | Main conclusions | ||
---|---|---|---|---|---|
In vitro incorporation efficiency | In vivo efficacy | ||||
3′-dATP | Ribose, 3′ | NAB | Chain terminator | Medium | Unreported |
3′-dCTP | Ribose, 3′ | NAB | Chain terminator | Medium | Unreported |
3′-dUTP | Ribose, 3′ | NAB | Chain terminator | Medium | Unreported |
Remdesivir-TP | Ribose, 1′ | SNA, VSNA | Polymerase backtrack | Very high | Very high |
T-1106-TP | Base | VSNA | Induces pauses (mutagenic) | Medium | Unreported |
Sofosbuvir-TP | Ribose, 2′ | NAB | Chain terminator | Very low | None |
ddhCTP | Ribose, 3′ | NAB | Chain terminator | low | None |
Additional files
-
Supplementary file 1
Summary of statistics, rates, and probabilities for each experimental condition presented in this study.
- https://cdn.elifesciences.org/articles/70968/elife-70968-supp1-v1.xls
-
Supplementary file 2
Summary of statistics, rates, and probabilities for each experimental condition presented in this study.
- https://cdn.elifesciences.org/articles/70968/elife-70968-supp2-v1.xls
-
Transparent reporting form
- https://cdn.elifesciences.org/articles/70968/elife-70968-transrepform-v1.docx