V-ATPase V0a1 promotes Weibel–Palade body biogenesis through the regulation of membrane fission
Figures
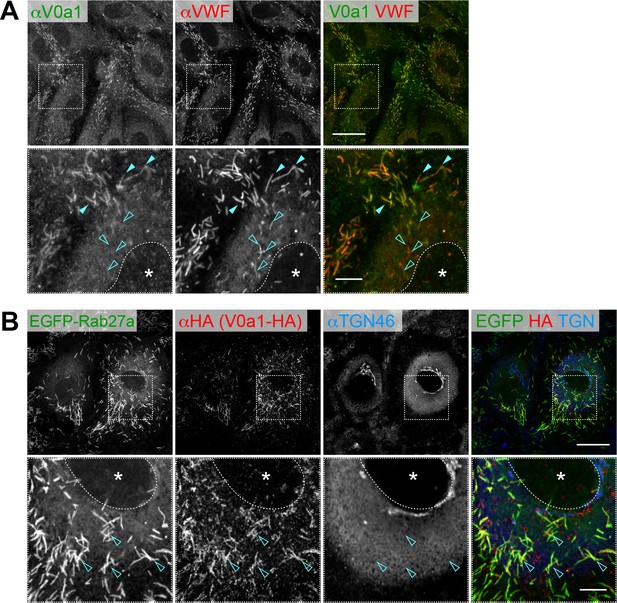
V0a1 is localized on peripheral mature Weibel–Palade bodies (WPBs) in human umbilical vein endothelial cells (HUVECs).
(A) Endogenous V0a1 protein was detected on the peripheral WPBs (closed arrowheads); however, it was not detected on the perinuclear WPBs (open arrowheads). (B) V0a1 was well-colocalized with EGFP-Rab27a, a mature WPB marker. Scale bars, 20 μm (wide field) and 5 μm (expanded). Asterisks, nucleus.
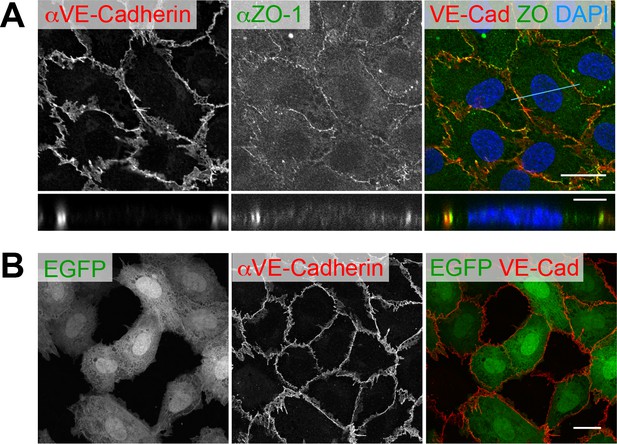
Lentivirus-mediated transduction does not show any visible alterations in human umbilical vein endothelial cells (HUVECs).
(A) HUVECs cultured on fibronectin for five days formed tight and adherens junctions. ZO-1 and VE-cadherin (tight and adherens junctional proteins, respectively) were localized along the cell boundary. Top panels, horizontal section; bottom panels, transverse sections. Scale bars, 20 μm (horizontal section) and 5 μm (transverse section). The line in the top panel marks the region where the transverse image was taken. (B) Lentivirus-mediated expression of EGFP did not disturb cellular junctions in HUVECs. Scale bar, 20 μm.
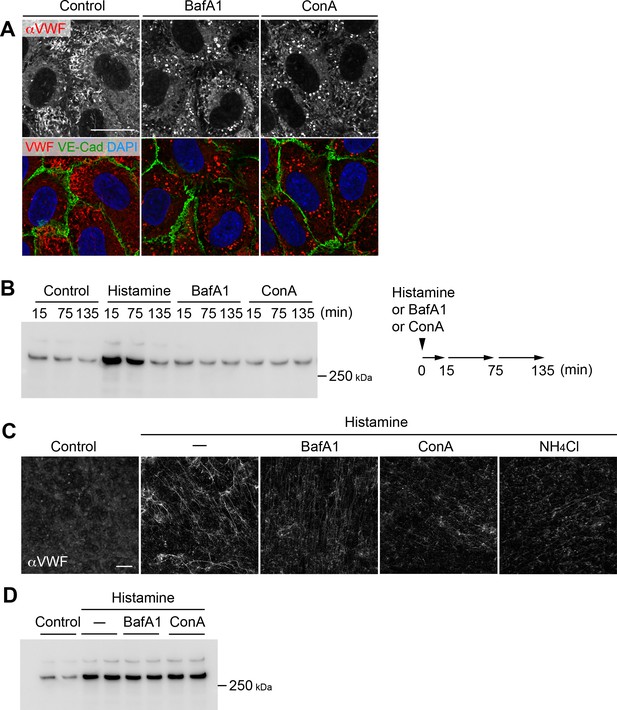
V-ATPase blockage and acidic compartment neutralization cause rounding of Weibel–Palade bodies (WPBs) and impair the efficient dispersion of von Willebrand factor (VWF) multimers.
(A) V-ATPase inhibitor exposure caused rounding of Weibel–Palade bodies. Human umbilical vein endothelial cells (HUVECs) cultured for 5 days were exposed to 100 nM bafilomycin A1 (BafA1) and concanamycin A (ConA) for 4 hr. Scale bar, 20 μm. (B) V-ATPase blockage did not alter the basal/constitutive secretion of VWF. HUVECs rested in the basal medium were exposed to histamine (100 μM), BafA1 (100 nM), or ConA (100 nM). Conditioned media was collected and refreshed at the indicated time points and immunoblotted using anti-VWF antibody. A schematic illustration of the experiments is shown on the right. (C) V-ATPase blockage and neutralization impaired the efficient dispersion of VWF multimers. HUVECs were pretreated with BafA1 (100 nM), ConA (100 nM), or NH4Cl (5 mM) for 4 hr and exposed to histamine (100 μM) for 15 min. Exocytosised VWF multimers were visualized using an anti-VWF antibody in the absence of detergent. Scale bar, 100 μm. (D) V-ATPase blockage did not alter histamine-evoked VWF secretion. HUVECs exposed to the V-ATPase inhibitors (100 nM for 4 hr) were stimulated using histamine (100 μM) for 15 min. Conditioned media was immunoblotted with an anti-VWF antibody. Samples were run in duplicates. Source files of all blots used in this figure are available in Figure 1—figure supplement 2—source data 1.
-
Figure 1—figure supplement 2—source data 1
Uncropped immunoblot images for Figure 1—figure supplement 2B and D.
- https://cdn.elifesciences.org/articles/71526/elife-71526-fig1-figsupp2-data1-v2.zip
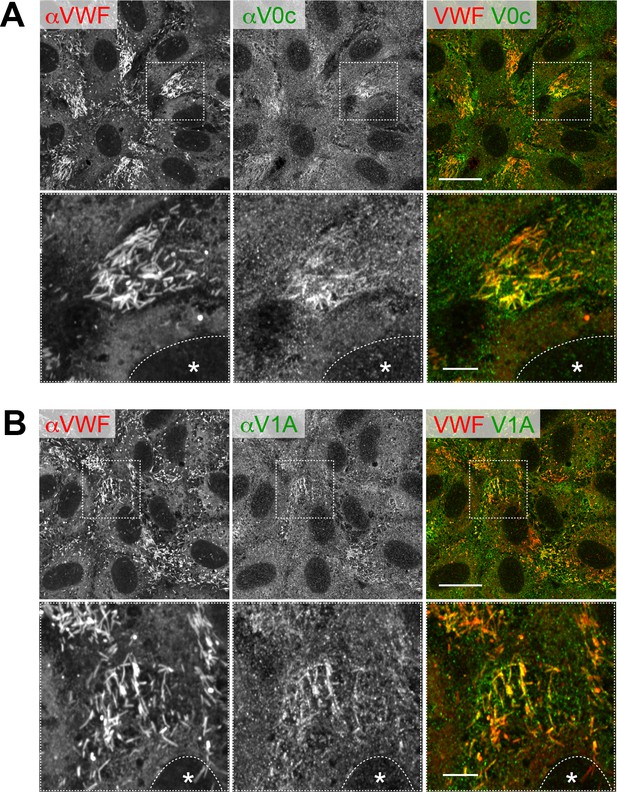
Two common V-ATPase subunits are detected in WPBs in HUVECs.
Endogenous V0c (A) and V1A (B) were visualized using immunofluorescence. Scale bars: 20 μm (wide field) and 5 μm (expanded).
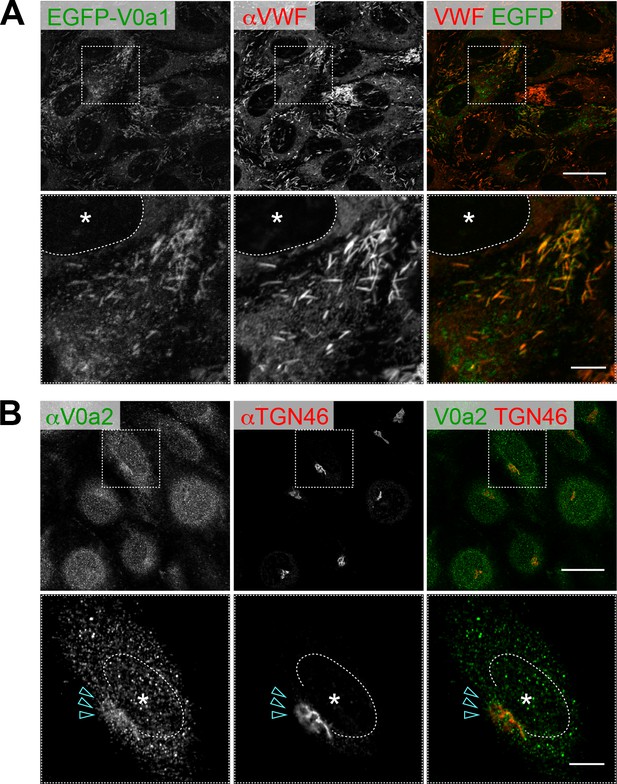
Two V0a isoforms are localized in distinct populations of Weibel–Palade bodies (WPBs) in human umbilical vein endothelial cells.
(A) EGFP-V0a1 was localized in peripheral WPBs. C-terminal EGFP-tagged V0a1 (V0a1-EGFP) showed the same subcellular localization. (B) Endogenous V0a2 was found on the trans-Golgi network and bud structure (arrowheads). Scale bars: 20 μm (wide field) and 5 μm (expanded). Asterisks, nucleus.
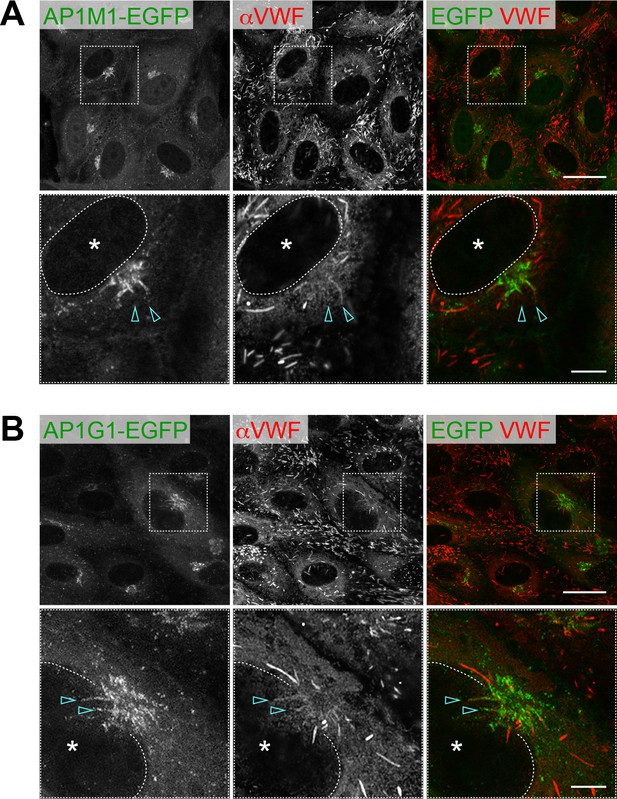
Two AP-1 components, AP1M1-EGFP (A) and AP1G1-EGFP (B), are localized around the perinuclear WPBs (arrowheads).
Scale bars: 20 μm (wide field) and 5 μm (expanded). Asterisks, nucleus.
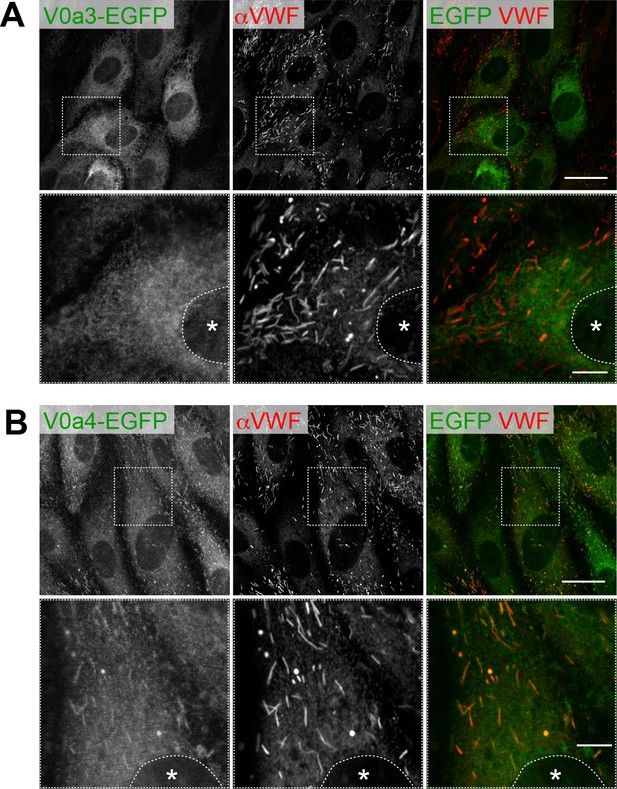
Subcellular localization of V0a3-EGFP (A) and V0a4-EGFP (B).
Although low levels of V0a4-EGFP are observed in WPBs, the majority was found elsewhere in the cells. Scale bars: 20 μm (wide field) and 5 μm (expanded). Asterisks, nucleus.
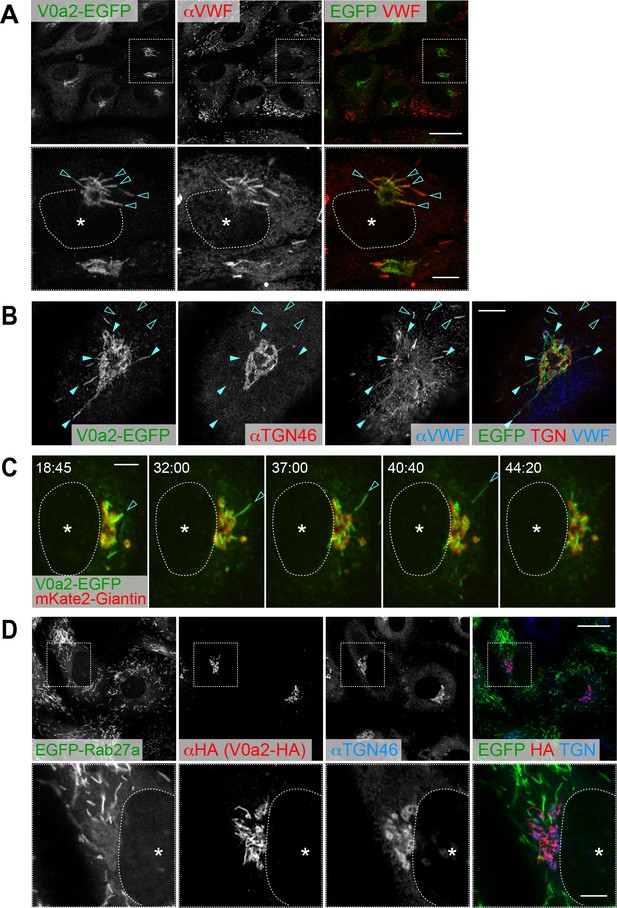
V0a2 is localized on the perinuclear newly forming Weibel–Palade bodies (WPBs).
(A) V0a2-EGFP was found on perinuclear WPBs (arrowheads); however, it was not found in the peripheral WPBs. N-terminal EGFP-tagged V0a2 (EGFP-V0a2) exhibited the same subcellular localization. Scale bars: 20 μm (wide field) and 5 μm (expanded). (B) Super resolution image around trans-Golgi network (TGN). V0a2-EGFP was observed on buds originating from the TGN (closed arrowheads). The von Willebrand factor signal observed in the V0a2 buds was faint compared to the strong signal in the post-budded WPBs (open arrowheads). Scale bar, 5 μm. (C) V0a2-EGFP (green) was localized on the newly forming buds in the Golgi apparatus (arrowhead) and disappeared as soon as the bud separates from the Golgi apparatus (mKate2-Giantin, red). Images were captured from the Video 1. Scale bar, 5 μm. (D) V0a2 buds were absent in Rab27a, a mature WPB marker. Scale bars: 20 μm (wide field) and 5 μm (expanded). Asterisks, nucleus.
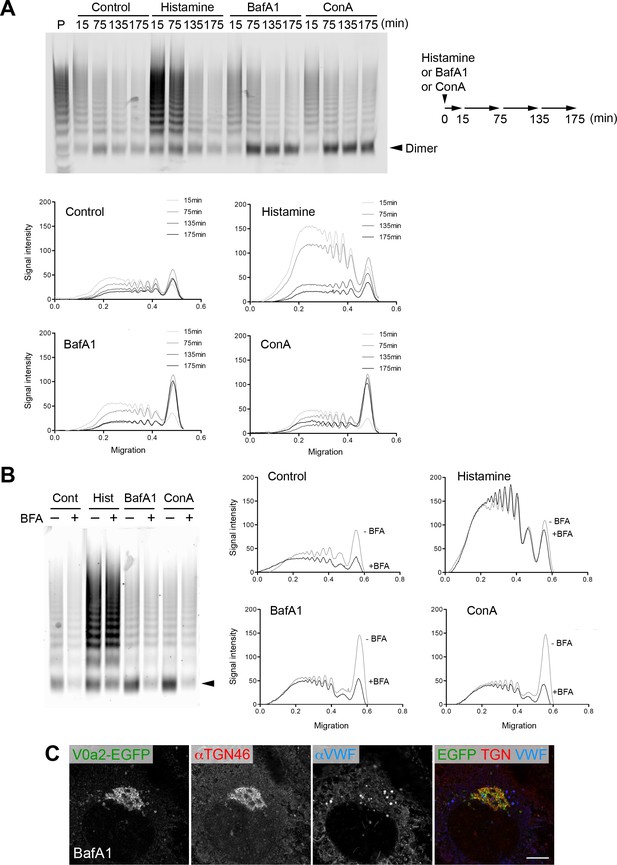
Cellular V-ATPase activity is required for von Willebrand factor (VWF) multimerization.
(A) Multimer profiles of basally/constitutively secreted VWF in the presence of a V-ATPase inhibitor. The samples used in the multimer assay are identical to the samples used in Figure 1—figure supplement 2B; Human umbilical vein endothelial cells (HUVECs) rested in basal medium were exposed to histamine (100 μM), bafilomycin A1 (BafA1, 100 nM), or concanamycin A (ConA, 100 nM). Conditioned media was collected at the indicated time points and evaluated using VWF multimer analysis (top). A schematic illustration of the experiments is shown on the right. Densitometric analysis was performed using Fiji software (bottom). Histamine exposure induced rapid exocytosis of large VWF multimers, whereas BafA1 and ConA treatment caused predominant secretion of the VWF dimer (arrowhead) without affecting the amount of secreted VWF (Figure 1—figure supplement 2B). P, human plasma as a VWF multimer control. (B) The dimer secretion induced by V-ATPase blockage occurred in the trans-Golgi network. After one hour preincubation with brefeldin A (BFA 100 ng/ml), HUVECs were exposed to BafA1 or ConA (100 nM) in the presence or absence of BFA (100 ng/ml). The collected media were evaluated using VWF multimer analysis. The dimer (arrowhead) secretion induced by V-ATPase inhibition was blocked by BFA. (C) V-ATPase activity was required for bud formation of nascent Weibel–Palade bodies. HUVECs were exposed to BafA1 (100 nM) for 175 min. V0a2 buds were no longer observed in these cells (compare to those in Figure 2B). Exposure to ConA (100 nM) and NH4Cl (5 mM) showed similar results. Scale bar, 5 μm. Source files of all blots used in this figure are available in Figure 2—figure supplement 1—source data 1.
-
Figure 2—figure supplement 1—source data 1
Uncropped immunoblot images for Figure 2—figure supplement 1A and B.
- https://cdn.elifesciences.org/articles/71526/elife-71526-fig2-figsupp1-data1-v2.zip
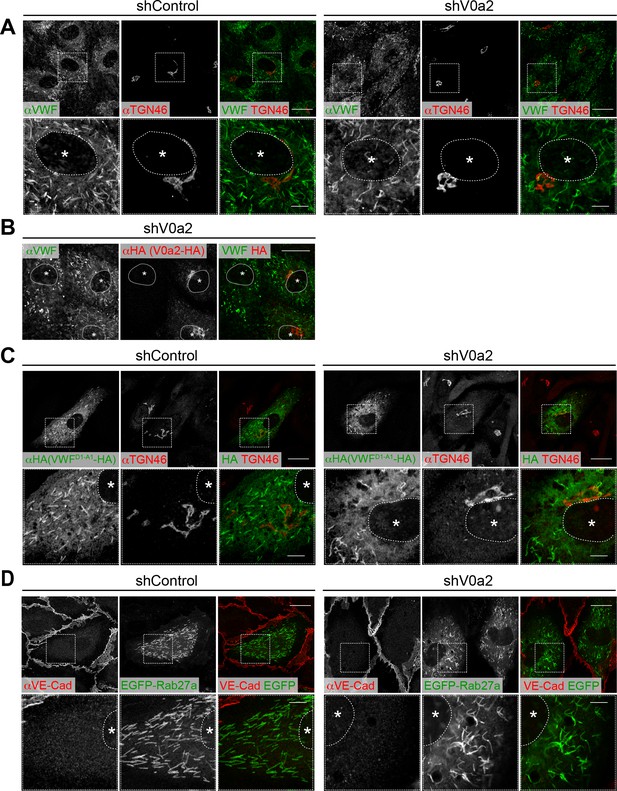
Depletion of V0a2 causes the formation of irregular, twisted Weibel–Palade bodies (WPBs).
(A) Irregular WPBs were generated in the V0a2-depleted cells. WPBs were visualized using immunofluorescence with anti-von Willebrand factor (VWF) antibody. Scale bars, 20 μm (wide field) and 5 μm (expanded). (B) The irregular WPBs were rescued by the induction of shRNA-resistant V0a2 (V0a2-HA). Scale bar, 20 μm. (C, D) WPBs separated from the trans-Golgi network and maturated even in the absence of V0a2. VWFD1-A1-HA (C) and EGFP-Rab27a (D) were introduced in the V0a2-depleted human umbilical vein endothelial cells. Due to limitations in the size for packaging into a lentivirus particle, we used VWFD1-A1 instead of full-length VWF. VWFD1-A1 is shown to function in the same manner as full-length VWF in cells (Michaux et al., 2006). Scale bars, 20 μm (wide field) and 5 μm (expanded). Asterisks, nucleus.
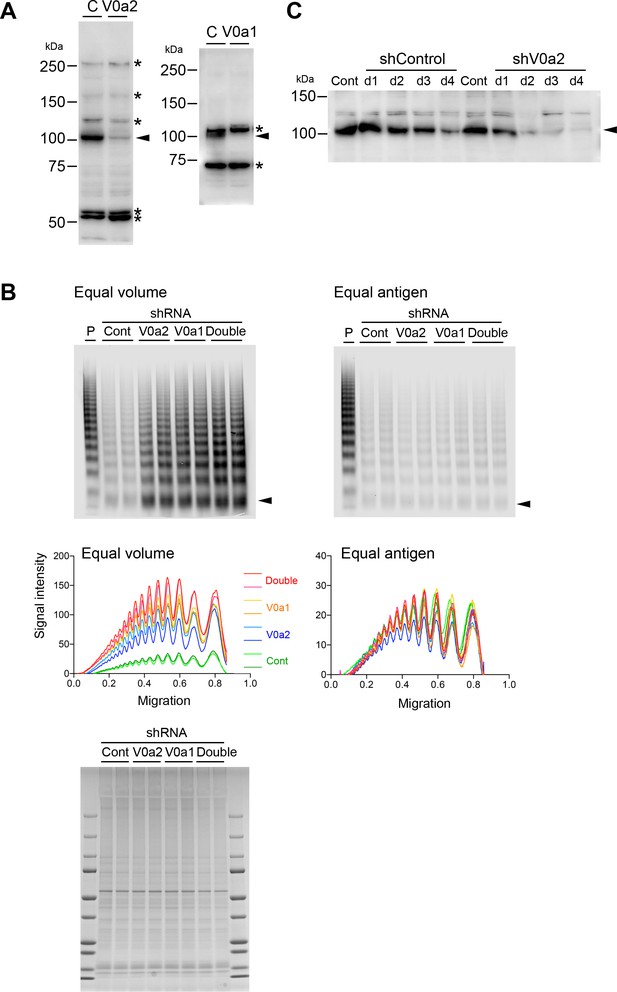
Depletion of the V0a isoforms does not alter von Willebrand factor (VWF) multimerization.
(A) Induction of shRNA against V0a2 (left) and V0a1 (right) depletes endogenous proteins in human umbilical vein endothelial cells (HUVECs) (arrowheads). The relative molecular mass (kDa) is shown on the right. Asterisks on the right indicate non-specific bands. (B) V0a2 and V0a1 were dispensable for VWF multimerization. Conditioned media from the V0a isoform-depleted cells were evaluated using VWF multimer assay (left: equal volume loaded, right: equal antigen loaded). CBB staining of cell lysate was performed to confirm the equal loading of the conditioned media (bottom). Samples were run in duplicates. Arrowheads indicate VWF dimer. (C) Sufficient depletion by shRNA was achieved on day 2 after transduction. HUVECs were transduced with lentiviruses expressing shRNA to V0a2. After the indicated time points (days 1–4), the residual amount of endogenous V0a2 protein was evaluated using immunoblotting (arrowhead). The relative molecular mass (kDa) is shown on the left. Source files of all blots used in this figure are available in Figure 3—figure supplement 1—source data 1.
-
Figure 3—figure supplement 1—source data 1
Uncropped immunoblot images for Figure 3—figure supplement 1A, B and C.
- https://cdn.elifesciences.org/articles/71526/elife-71526-fig3-figsupp1-data1-v2.zip
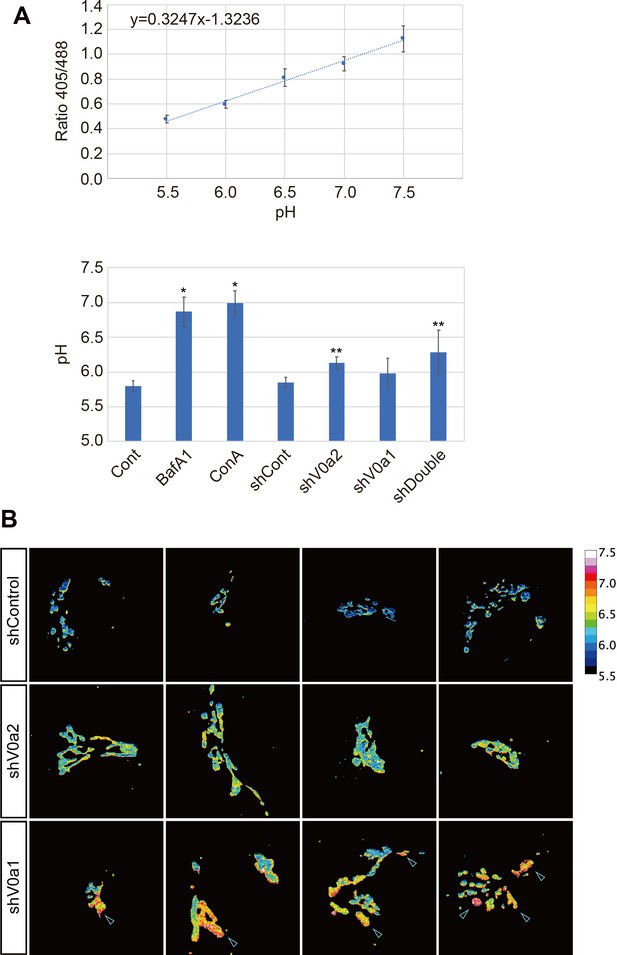
V-ATPase blockage causes almost total neutralization of the luminal TGN pH, while the depletion of the V0a isoforms results in a modest pH increase.
(A) Intraluminal pH of the TGN was measured by using GalT-pHluorin that allows to estimate cellular pH in a range of 5.5–7.5 (top). Depletion of the V0a isoforms caused a modest increase in pH compared with V-ATPase blockage (bottom). *p < 0.05 to Control, **p < 0.05 to shControl (n = 12, average± SD). (B) V0a2 depletion resulted in a slight and homogenous increase of the luminal TGN pH, whereas V0a1 depletion caused relatively large pH increase in the some TGN subdomains (arrowheads). Luminal TGN pH was calculated from ratiometric images generated from GalT-pHluorin images (displayed in a pseudocolor). Four representative images are shown for each group. Source file of all graph data shown in this figure is available in Figure 3—figure supplement 2—source data 1.
-
Figure 3—figure supplement 2—source data 1
Graph data for Figure 3—figure supplement 2A.
- https://cdn.elifesciences.org/articles/71526/elife-71526-fig3-figsupp2-data1-v2.zip
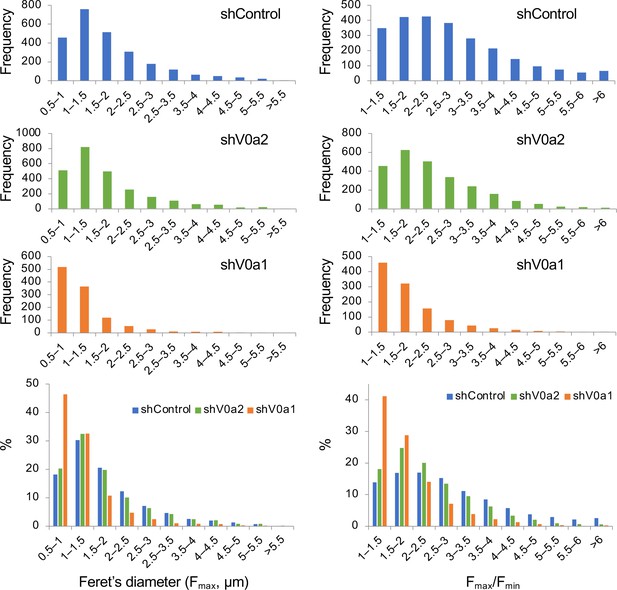
Frequency distribution of the longest Feret’s diameter (Fmax) and the aspect ratio of the longest and shortest distance (Fmax/Fmin) of individual WPBs in the V0a-depleted cells.
The longest (Fmax) and shortest (Fmin) distances between any two points along individual WPBs were measured by Fiji. Frequency distribution of Feret’s diameter (Fmax, left panels) and Fmax/Fmin ratio (right panels) of WPB particles were shown as histograms. n = 2509 (shControl), n = 2520 (shV0a2), and n = 1118 (shV0a1). For easier comparison, proportional distributions of the values were shown in the bottom. Source file of all graph data shown in this figure is available in Figure 3—figure supplement 3—source data 1.
-
Figure 3—figure supplement 3—source data 1
Graph data for Figure 3—figure supplement 3.
- https://cdn.elifesciences.org/articles/71526/elife-71526-fig3-figsupp3-data1-v2.zip
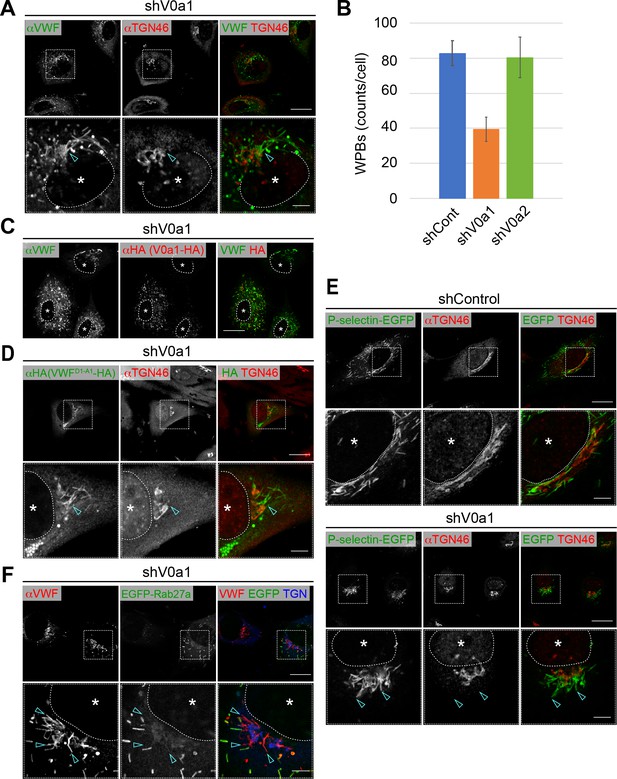
Weibel–Palade bodies (WPBs) cannot be separated from the trans-Golgi network in the absence of V0a1.
(A) V0a1 depletion caused the perinuclear accumulation of WPBs (arrowheads, compared to that in the shControl in Figure 3A). Scale bars, 20 μm (wide field) and 5 μm (expanded). (B) The depletion of V0a1 resulted in the significant decrease in the number of WPBs (counts per cell, average± SD). We confirmed that there are no obvious difference in cell size between the V0a isoform depleted cells and the control. n = 30 (shControl), n = 29 (shV0a1), and n = 31 (shV0a2). Eight independent fields were collected from each imaging sample. The images were analyzed using Fiji. *p < 0.05 to shControl. (C) The phenotype mediated by V0a1 depletion was rescued by the induction of a shRNA-resistant V0a1 (HA-V0a1). Scale bar, 20 μm. (D, E) WPB contents were trapped in the perinuclear WPBs that accumulated in the absence of V0a1. VWFD1-A1-HA (D) and P-selectin-EGFP (E) were transfected in V0a1-depleted cells. Both proteins were observed in the accumulated WPBs (arrowheads, compared to that in the shControl in Figure 3C for VWFD1-A1-HA). (F) EGFP-Rab27a was absent in perinuclear WPBs that accumulated in V0a1-depleted cells (arrowheads). Scale bars, 20 μm (wide field) and 5 μm (expanded). Asterisks, nucleus. Source files of all graph data shown in this figure are available in Figure 4—source data 1.
-
Figure 4—source data 1
Graph data for Figure 4B.
- https://cdn.elifesciences.org/articles/71526/elife-71526-fig4-data1-v2.zip
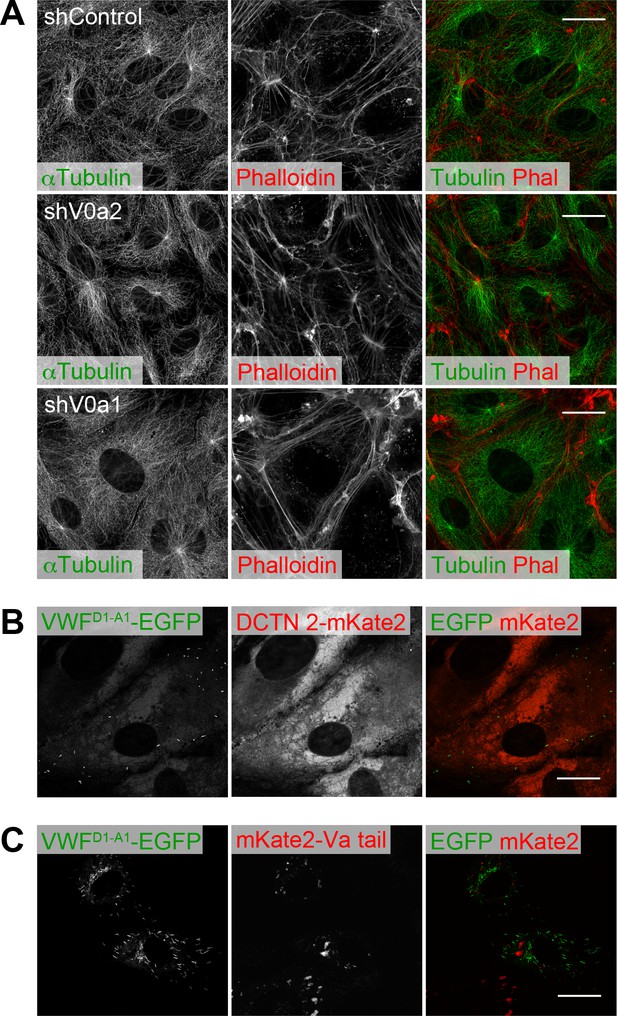
Microtubule and actin networks are unaffected by depletion of V0a isoforms.
(A) Microtubule and actin networks in V0a isoform-depleted cells. Microtubule and actin networks were visualized using an anti-α-tubulin antibody and phalloidin, respectively. (B, C) The induction of dominant-negative forms that block microtubule and actin network mediating transport did not cause the perinuclear accumulation of WPBs, as observed in the V0a1-depleted cells. VWFD1-A1-EGFP was introduced into dynactin 2-mKate2 (B) or mKate2-Va tail (C)-expressing human umbilical vein endothelial cells. WPBs were almost totally absent in the dynactin 2-mKate2-expressing cells. Scale bars, 20 μm.
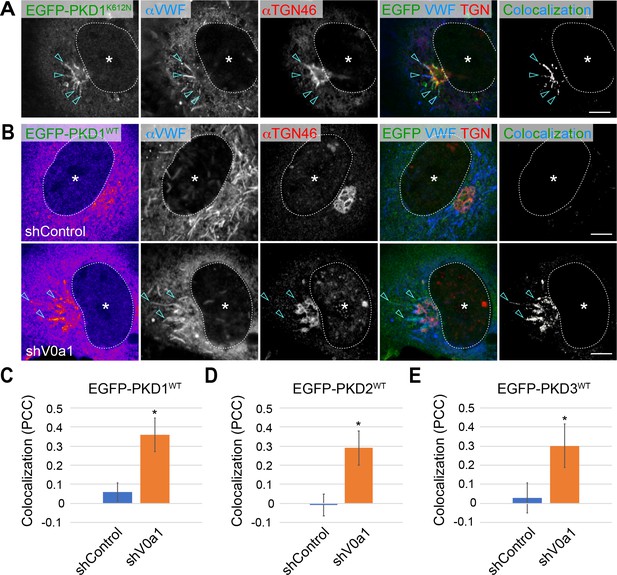
Weibel–Palade bodies (WPB) biogenesis requires protein kinase D1 (PKD1).
(A) The von Willebrand factor (VWF) was trapped in the tubules (arrowheads) formed by the induction of a dominant-negative form of PKD1 (EGFP-PKD1K612N) because of the blockage of PKD1-mediating membrane fission at the TGN (arrowheads). (B) EGFP-PKD1WT was accumulated on the perinuclear WPBs that accumulated in the absence of V0a1. The channel overlap of EGFP-PKD1WT and VWF are shown as ‘colocalization’ on the right. Scale bars, 5 μm. C–E. The localization of EGFP-PKDs on WPBs was significantly increased in the V0a1-depleted cells. The colocalization of VWF and EGFP-PKD1WT (C), EGFP-PKD2WT (Figure 5—figure supplement 3D), and EGFP-PKD3WT (Figure 5—figure supplement 3E) is shown as Pearson’s correlation coefficient (PCC, average± SD). n = 14 (VWF and EGFP-PKD1WT), n = 12 (VWF and EGFP-PKD2WT), and n = 13 (VWF and EGFP-PKD3WT). *p < 0.05 to shControl. Asterisks, nucleus. Source file of the graph data shown in C–E is available in Figure 5—source data 1.
-
Figure 5—source data 1
Graph data for Figure 5C–E.
- https://cdn.elifesciences.org/articles/71526/elife-71526-fig5-data1-v2.zip
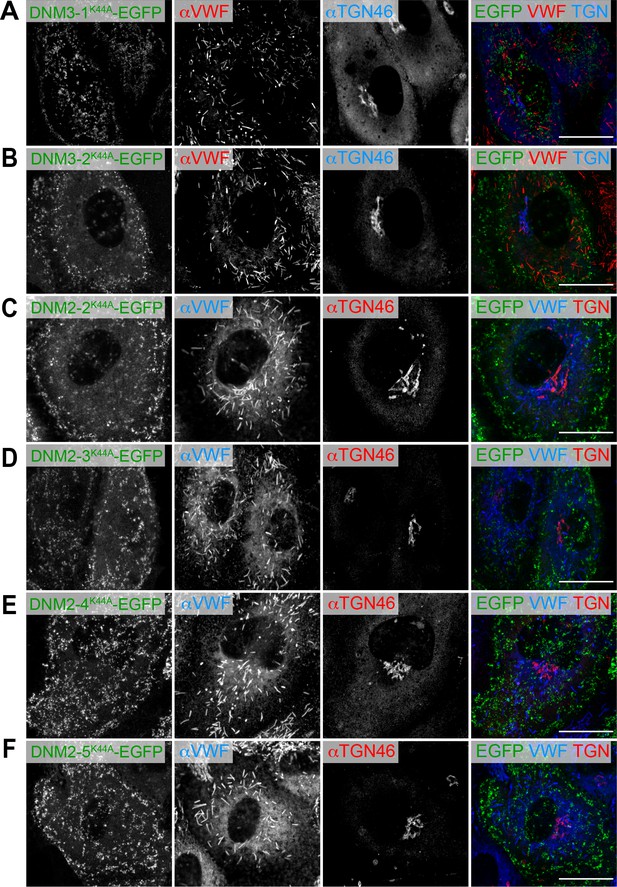
Induction of dominant-negative forms of dynamins does not alter the biogenesis of Weibel–Palade bodies.
(A–F) Human umbilical vein endothelial cells were introduced with EGFP-tagged dominant-negative forms of dynamin 3 (isoforms 1 and 2) and dynamin 2 (isoforms 2, 3, 4, and 5). Scale bars, 20 μm.
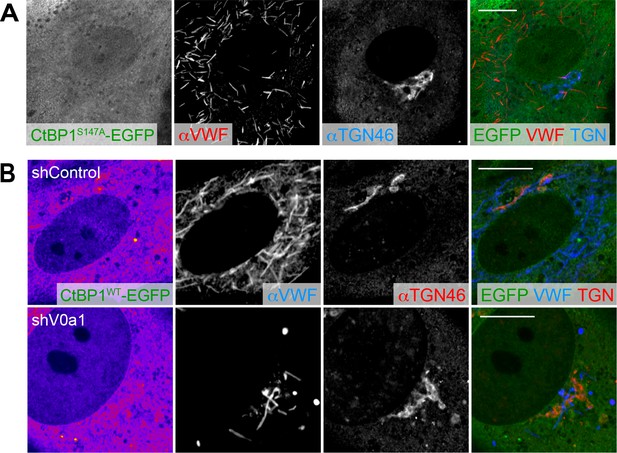
Induction of dominant-negative forms of CtBP1/BARS does not alter the biogenesis of Weibel–Palade bodies.
(A) Human umbilical vein endothelial cells were introduced with EGFP-tagged dominant-negative forms of CtBP1/BARS. (B) EGFP-tagged wild-type CtBP1/BARS was introduced into V0a1-depleted cells. Although faint signal of EGFP-CtBP1/BARSWT was observed around the trans-Golgi network, no noticeable difference was found. Scale bars, 10 μm.
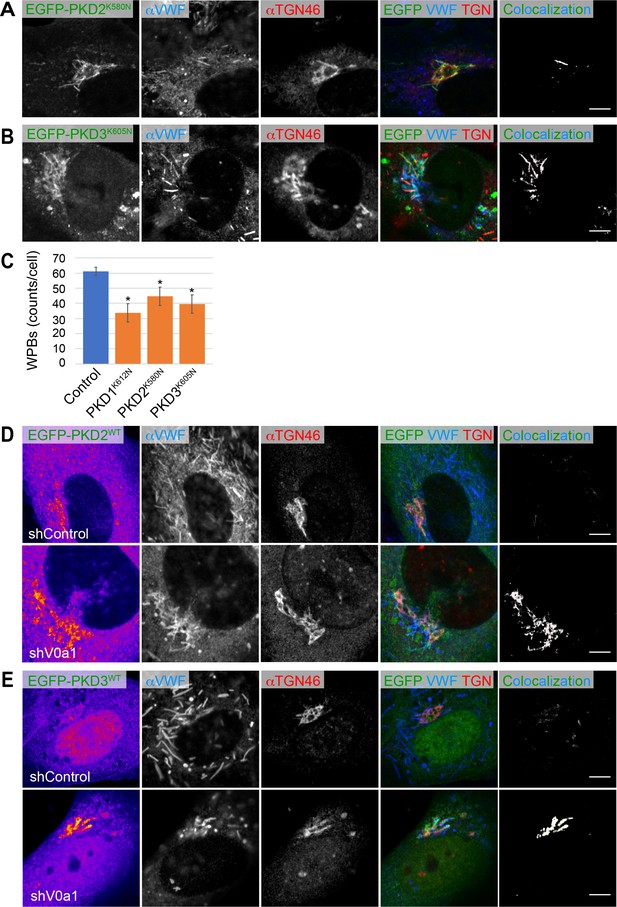
Biogenesis of Weibel–Palade bodies (WPBs) requires protein kinase D (PKD).
(A, B) The von Willebrand factor (VWF) was trapped in the tubules formed by the induction of dominant-negative forms of PKD2 (EGFP-PKD2K580N) and PKD3 (EGFP-PKD3K605N). (C) The induction of dominant-negative forms of PKDs (EGFP-PKD1K612N, EGFP-PKD2K580N, EGFP-PKD3K605N) resulted in the substantial decrease in the number of WPBs (counts per cell, average± SD). EGFP-introduced cells were used as the control. Eight independent fields were collected from each imaging sample. n = 96 (EGFP), n = 84 (EGFP-PKD1K612N), n = 78 (EGFP-PKD2K580N), and n = 67 (EGFP-PKD3K605N). *p < 0.05 to the control. (D, E) EGFP-PKD2WT (D), and EGFP-PKD3WT (E) accumulated in the perinuclear WPBs in the absence of V0a1. The channel overlap of PKD and VWF is shown as ‘colocalization’ on the right. Scale bars, 5 μm. Source file of the graph data shown in this figure is available in Figure 5—figure supplement 3—source data 1.
-
Figure 5—figure supplement 3—source data 1
Graph data for Figure 5—figure supplement 3C.
- https://cdn.elifesciences.org/articles/71526/elife-71526-fig5-figsupp3-data1-v2.zip
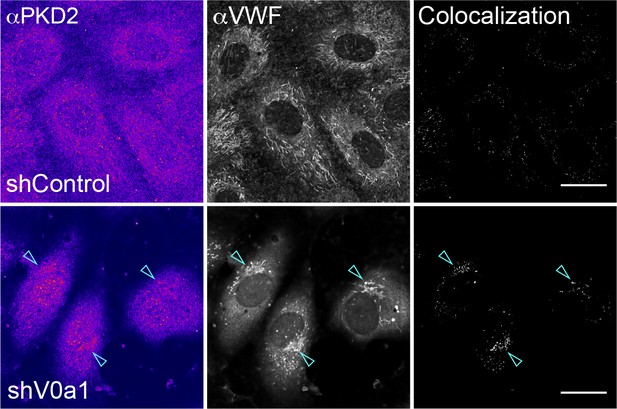
Endogenous protein kinase D2 (PKD2) protein accumulates in the trans-Golgi network (TGN) in the absence of V0a1.
Endogenous PKD2 was found in Weibel–Palade bodies accumulated in the TGN of V0a1-depleted cells (arrowheads). The channel overlap of PKD2 and von Willebrand factor is shown as colocalization on the right. We observed similar perinuclear staining with other anti-PKD2 antibody. Scale bars, 20 μm.
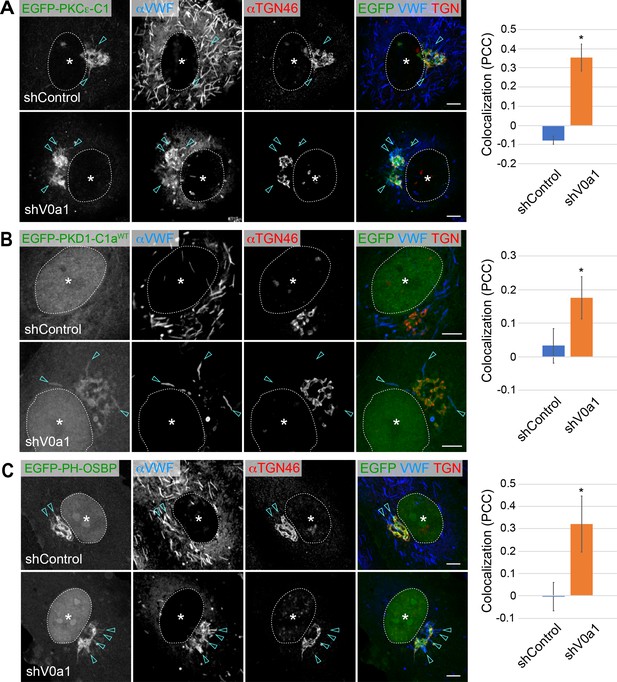
Diacylglycerol (DAG) and phosphatidylinositol-4-phosphate (PI4P) are present on newly forming Weibel–Palade bodies (WPBs).
(A, B) DAG was present on newly forming perinuclear WPBs. Two specific DAG reporters, EGFP-PKCε-C1 (A) and EGFP-PKD1-C1a (B), were introduced into human umbilical vein endothelial cells. EGFP-PKCε-C1 (A) is found in the perinuclear WPBs of the control (arrowheads). The signal is more evident in the accumulated WPBs in V0a1-depleted cells (arrowheads). EGFP-PKD1-C1a (B) is found in the accumulated WPBs in V0a1-depleted cells (arrowheads). Since PKD1-C1a is not a high-affinity DAG reporter as PKCε-C1, it may explain why the signal is not evident in the control. The colocalization of von Willebrand factor (VWF) and EGFP-PKCε-C1 (A) and EGFP-PKD1-C1a (B) is shown as Pearson’s correlation coefficient (PCC, average± SD) on the right. n = 19 (VWF and EGFP-PKCε-C1), and n = 14 (VWF and EGFP-PKD1-C1a). *p < 0.05 to shControl. (C) PI4P was present in perinuclear WPBs. EGFP-PH-OSBP, a PI4P reporter, was found in few perinuclear WPBs in the control (arrowheads). The signal is more evident in V0a1-depleted cells (arrowheads). The colocalization of VWF and EGFP-PH-OSBP is shown as Pearson’s correlation coefficient (PCC, average ± SD) on the right. n = 18 (VWF and EGFP-PH-OSBP). *p < 0.05 to shControl. Scale bars, 5 μm. Asterisks, nucleus. Source file of the graph data shown in 6 A–C is available in Figure 6—source data 1.
-
Figure 6—source data 1
Graph data for Figure 6A–C.
- https://cdn.elifesciences.org/articles/71526/elife-71526-fig6-data1-v2.zip
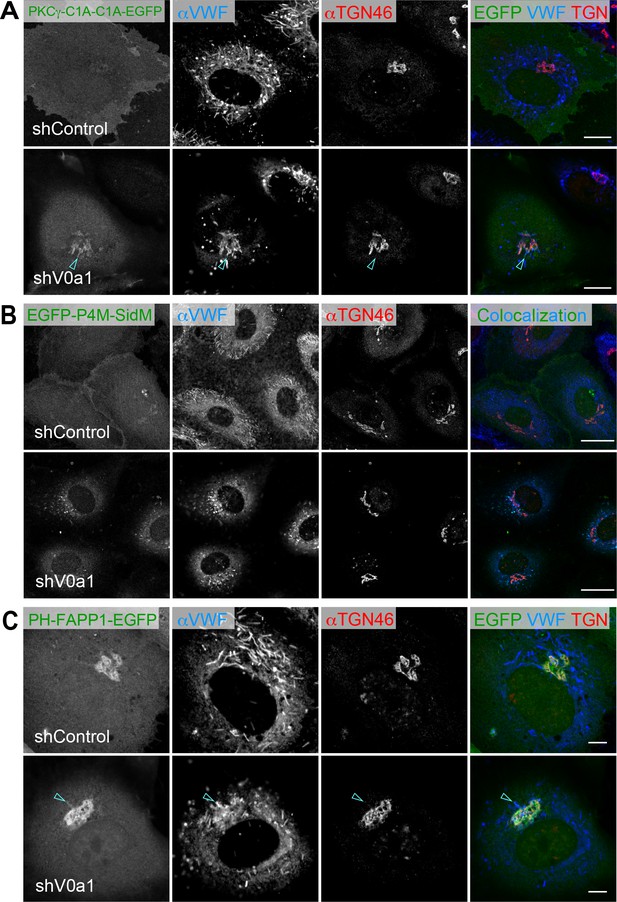
Diacylglycerol (DAG) and phosphatidylinositol-4-phosphate (PI4P) are present on newly forming Weibel–Palade bodies (WPBs).
(A) PKCγ-C1A-C1A-EGFP, a DAG reporter, was detected in perinuclear WPBs in V0a1-depleted cells (arrowheads). Scale bar, 10 μm. (B, C) PI4P was present in perinuclear WPBs. Two PI4P reporters, EGFP-P4M-SidM (B) and PH-FAPP1-EGFP (C), were introduced into human umbilical vein endothelial cells. Both were detected in WPBs accumulated upon V0a1-depletion. Scale bars: 20 μm (B) and 5 μm (C).
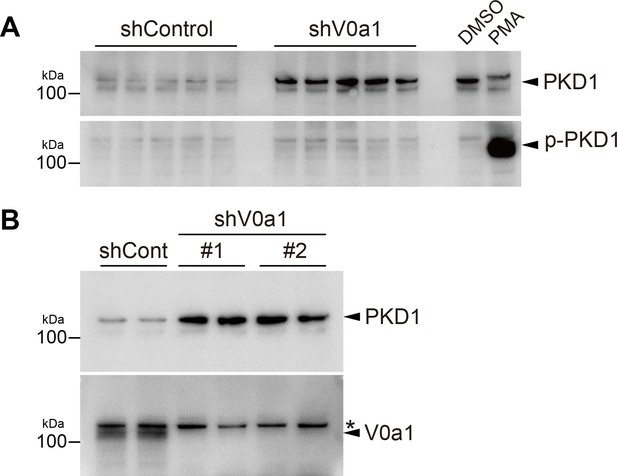
Active/phosphorylated PKD1 is unchanged independent from the absence or presence of V0a1, whereas V0a1-depletion causes an increase of total PKD1 protein.
(A) HUVEC lysate (five independent samples for each) was immunoblotted with total PKD1 (top) and phospho-PKD1(pSer744/748) antibodies (bottom). PMA exposure (100 nM, 10 min) massively induced PKD1 phosphorylation on the activation loop. (B) Transfection of two independent shRNAs against V0a1 shows a similar increase of endogenous PKD1 protein. HUVEC lysate (two independent samples for each) was immunoblotted with total PKD1 (top) and V0a1 antibodies (bottom). shV0a1#1, TRCN0000380234, targeting the 3′-UTR region and shV0a1#2, TRCN0000333635, targeting the coding region around Tyr620-Met627. Asterisk on the right indicates non-specific bands. The relative molecular mass (kDa) is shown on the left. Source files of all blots used in this figure are available in Figure 6—figure supplement 2—source data 1.
-
Figure 6—figure supplement 2—source data 1
Uncropped immunoblot images for Figure 6—figure supplement 2.
- https://cdn.elifesciences.org/articles/71526/elife-71526-fig6-figsupp2-data1-v2.zip
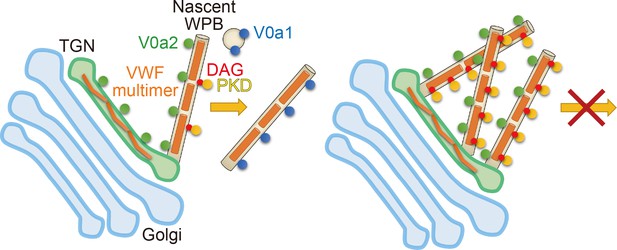
The biogenesis of Weibel–Palade bodies (WPBs) requires vacuolar H+-ATPase V0a1 and PKD for the membrane fission.
V0a1 on small vesicles such as endosomes could drive the membrane fission (see Discussion). In the absence of V0a1, nascent WPBs could not separate from the trans-Golgi network (right). PKD activity was also required for the biogenesis of WPBs. Since the induction of wild-type PKDs did not support the segregation of WPBs from the TGN in the absence of V0a1, V0a1 appeared to be a primary factor in the membrane fission of WPBs.
Videos
V0a2-EGFP is found on bud of newly forming Weibel–Palade bodies.
V0a2-EGFP (green) disappeared as soon as it segregates from the Golgi apparatus (red, mKate2-Giantin).
Weibel–Palade bodies (WPBs) in the control shRNA introduced cells.
Accumulated Weibel–Palade bodies (WPBs) at the Golgi apparatus in the V0a1-depleted cells are static compared to those in the control.
Tables
Reagent type (species) or resource | Designation | Source or reference | Identifiers | Additional information |
---|---|---|---|---|
Gene (Homo sapiens) | ATP6V0A1 | NA | Q93050 (VPP1_HUMAN) | V-type proton ATPase 116 kDa subunit a1 |
Gene (Homo sapiens) | ATP6V0A2 | NA | Q9Y487 (VPP2_HUMAN) | V-type proton ATPase 116 kDa subunit a2 |
Cell line (Homo sapiens) | Lenti-X 293T Cell Line | Clontech | Cat#632180 | |
Cell line (Homo sapiens) | HUVEC | Lonza | Cat#C2519A | |
Antibody | anti-VE-Cadherin (mouse monoclonal) | R&D Systems | Cat#123413RRID:AB_2260374 | (1:1,000) |
Antibody | anti-ZO-1 (rabbit monoclonal) | CST | Clone:D6L1ERRID:AB_2798287 | (1:50) |
Antibody | anti-VWF (rabbit polyclonal) | Dako/Agilent Technologies | Cat#P0226RRID:AB_579516 | (1:1,000) |
Antibody | anti-VWF (sheep polyclonal) | Abcam | Cat#ab11713RRID:AB_298501 | (1:500) |
Antibody | anti-V0c (rabbit polyclonal) | Thermo-Fisher Scientific | Cat#PA5-66746RRID:AB_2662731 | (1:25) |
Antibody | anti-V1A (rabbit polyclonal) | Thermo-Fisher Scientific | Cat#PA5-65137RRID:AB_2662833 | (1:125) |
Antibody | anti-V0a1 (rabbit polyclonal) | Novus Biologicals | Cat#NBP1-89342RRID:AB_11015740 | (1:200) |
Antibody | anti-HA (mouse monoclonal) | Covance | Clone:16B12RRID:AB_10064068 | (1:1,000) |
Antibody | anti-TGN46 (sheep polyclonal) | Bio-Rad | Cat#AHP500GTRRID:AB_2203291 | (1:500) |
Antibody | anti-V0a2 (rabbit polyclonal) | Atlas Antibodies | Cat#HPA044279RRID:AB_10961274 | (1:50) |
Antibody | anti-V0a2 (rabbit polyclonal) | My BioSource | Cat#MBS8527160 | (1:100) |
Antibody | anti-α-tubulin (mouse monoclonal) | CST | Clone:DM1ARRID:AB_1904178 | (1:2,000) |
Antibody | anti-PKD2 (rabbit monoclonal) | Abcam | Clone:EP1495YRRID:AB_922233 | (1:200) |
Antibody | anti-PKD2 (rabbit polyclonal) | GeneTex | Cat#C1C3RRID:AB_1951437 | (1:200) |
Antibody | anti-phospho-PKD/PKCμ(Ser744/448) (rabbit polyclonal) | CST | Cat#2054RRID:AB_2172539 | (1:1,000) |
Antibody | anti-PKD/PKCμ (rabbit monoclonal) | CST | Clone:D4J1NRRID:AB_2800149 | (1:1,000) |
Antibody | Alexa 488-, 555-, 647 secondaries | Thermo Fisher Scientific | (1:400) | |
Antibody | Cy3-secondary | Jackson Immunoresearch | (1:400) | |
Recombinant DNA reagent | pLVSIN-CMV Pur Vector | Clontech | Cat#6183 | |
Recombinant DNA reagent | Mission shRNA plasmid non-targeting control | Sigma-Aldrich | SHC016 | |
Recombinant DNA reagent | Mission shRNA plasmid against V0a1 | Sigma-Aldrich | TRCN0000380234 | Mainly used throughout the study. |
Recombinant DNA reagent | Mission shRNA plasmid against V0a1 | Sigma-Aldrich | TRCN0000333635 | Used in Figure 6—figure supplement 2B. |
Recombinant DNA reagent | Mission shRNA plasmid against V0a2 | Sigma-Aldrich | TRCN0000043494 | |
Chemical compound, drug | Bafilomycin A1 | Cayman Chemicals | Cat#11038 | 100 nM |
Chemical compound, drug | iFluor555-conjugated phalloidin | Cayman Chemicals | Cat#20552 | (1:400) |
Chemical compound, drug | Concanamycin A | AdipoGen Life Sciences | Cat#BVT-0237 | 100 nM |
Chemical compound, drug | Brefeldin A | Thermo Fisher Scientific | Cat#B7450 | 100 ng/ml |
Chemical compound, drug | Histamine | Sigma-Aldrich | Cat#H7250 | 100 μM |
Chemical compound, drug | TransIT Lenti transfection reagent | Clontech | Cat#MIR6600 | |
Chemical compound, drug | Lentiviral High Titer Packaging Mix | Clontech | Cat#6194 |