Distributed coding of duration in rodent prefrontal cortex during time reproduction
Figures
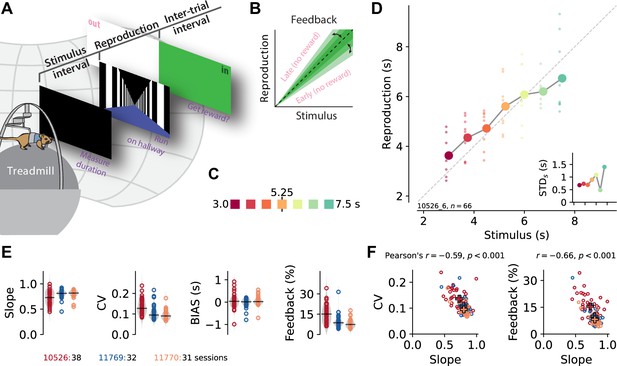
A time reproduction task for rodents.
(A) Experimental apparatus and task. A gerbil was placed on top of a treadmill surrounded by a projection screen. Each trial started with a timed stimulus (black screen). The animal had to measure its duration and, when a virtual linear corridor appeared, reproduce the duration by walking. If the reproduction was close to the stimulus duration (‘in’), a food reward was delivered and the entire screen was set to green for 3–4 s before another trial was initiated; otherwise, screen color was set to white (‘out’). (B) The feedback range was narrowed/widened after each in/out response. (C) Stimulus intervals were randomly sampled from a discrete uniform distribution with seven values between 3 and 7.5 s. Colors identify stimulus duration and will be used throughout the paper. (D) Behavioral responses exhibited specific characteristics. Single reproductions (small dots) and their averages (large connected circles) showed the regression effect. Inset: standard deviation increased with stimulus duration (scalar variability). Same x-axis as in the main panel. Data in the main panel and inset are from one example session (of animal 10526). (E) Slope of the linear regression between stimuli and reproductions – quantifying the strength of the regression effect, with values closer to 1 meaning less regression, – coefficient of variation (CV), average bias, and average tolerance of the feedback range for each session sorted by animal. Values from single sessions are displayed as open circles. Gray violin plots illustrate distributions, and black solid lines mark the medians. Color identifies animals; see also ID numbers below the panels. (F) Slope negatively correlates with CV and feedback range across animals and sessions, indicating stronger regression effects with more variable responses. Open circles correspond to single sessions. Crosses mark averages for each animal. Color code as in (E).
-
Figure 1—source data 1
Source data for Figure 1E&F and Figure 1—figure supplement 2A–C.
- https://cdn.elifesciences.org/articles/71612/elife-71612-fig1-data1-v2.csv
-
Figure 1—source data 2
Source data for Figure 1—figure supplement 2C1 and C2 and Figure 1—figure supplement 3.
- https://cdn.elifesciences.org/articles/71612/elife-71612-fig1-data2-v2.csv
-
Figure 1—source data 3
Source data for Figure 1—figure supplement 2D.
- https://cdn.elifesciences.org/articles/71612/elife-71612-fig1-data3-v2.csv
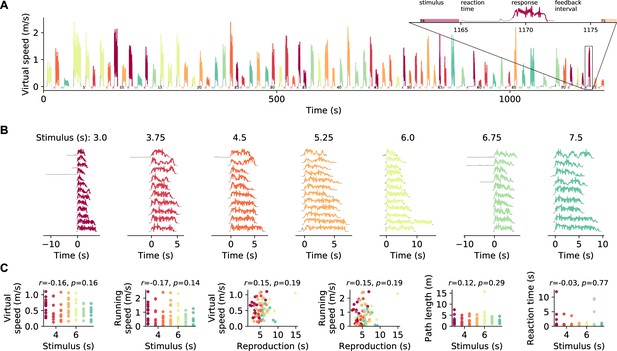
Behavioral data from an example session.
(A) Virtual speed, that is, the speed of visual movement an animal experiences, over the course of a session. Colored traces mark the reproductions (responses); gray otherwise. Color identifies stimulus duration. The 74th trial is magnified and labeled with trial phases. Position was tracked during the response phase but not during measurement and feedback. The interval between the end of stimulus presentation and the begin of the response we call ‘reaction time.’ To finish the response, the animal had to stop for at least 0.5 s. (B) Virtual speed in individual trials sorted by stimulus duration. (C) Averages for each trial of the virtual speed, the running speed of the animal on the treadmill, the path length covered during the response, and the reaction time sorted by stimulus duration or reproduction. Again color identifies stimulus duration. Pearson’s r and p-values are given on top of each panel. The data belongs to the session displayed in Figure 1D.
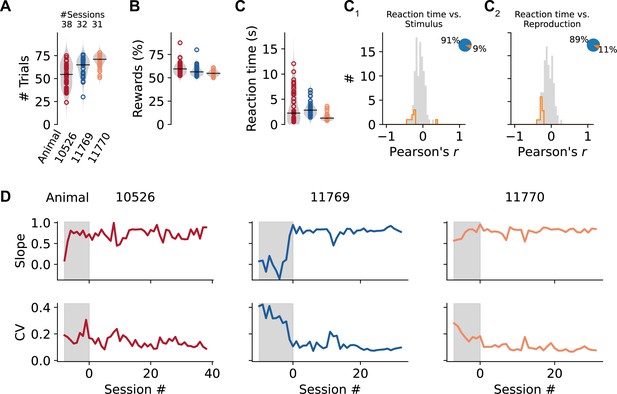
Reward rates, reaction times, and training data.
(A) Number of trials in the experimental sessions sorted by animal. Values from single sessions are displayed as open circles. Violin plots illustrate the distribution, and black solid lines mark the median. Color identifies individual animals. (B) Percentage of rewards in the sessions. (C) Average ‘reaction time’ in each session. (C1, C2) Distributions of Pearson’s correlation coefficients between reaction time and stimulus or reproduced durations. Histograms are displayed in gray with significant values delimited by an orange outline (p<0.05). Pie plots show significant (orange) and nonsignificant (blue) fractions. (D) Development of slope and coefficient of variation across sessions for each animal. Gray shaded areas and negative session numbers mark training sessions. Data from training sessions is not included in any other part of this paper.
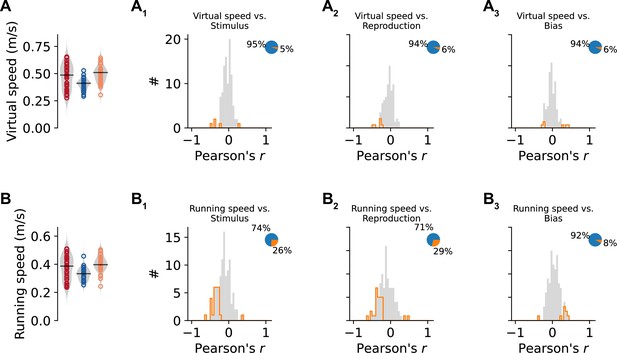
Speed vs. other behavioral parameters.
(A) Average virtual speed in each session and distributions of Pearson’s correlations with stimulus duration (A1), reproduced duration (A2), and bias (i.e., reproduced – stimulus duration, A3). (B) Same as (A) for running speed of the animal on the treadmill. Values from single sessions are displayed as open circles. Violin plots illustrate the distribution, and black solid lines mark the median. Color identifies animals. Histograms are displayed in gray with significant values delimited by an orange outline (p<0.05). Pie plots show significant (orange) and nonsignificant (blue) fractions.
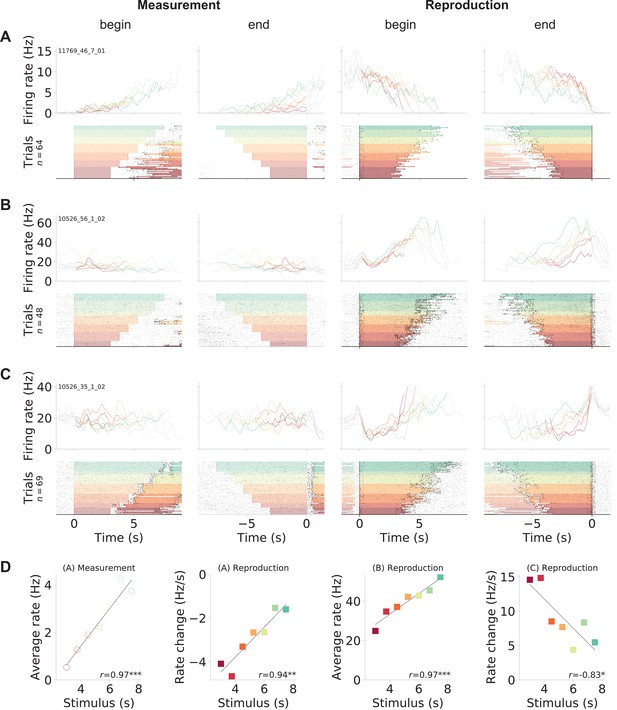
Gerbil medial prefrontal cortex (mPFC) neuron responses during time reproduction.
(A) A cell that linearly increased its firing rate during measurement and ramped down to zero during reproduction. (B) A neuron that scaled its firing with the stimulus duration during reproduction and (C) a ramp-to-threshold cell. (A–C) Panels display spike raster plots sorted by stimulus duration (bottom) and corresponding spike density functions (SDF, top). Each column plots the data with different alignment, that is, measurement begin and end, reproduction begin and end. Color identifies stimulus duration as in Figure 1C. In the raster plots, black ticks are single spikes. For better visualization, we only plot half of the spikes (randomly chosen). Measurement or reproduction phases are delimited by underlayed color. The SDFs are colored in the respective task phase, outside they are displayed as thin gray lines. (D) Dependence of firing on stimulus duration in the example cells. Single markers show the average firing rate or change of firing rate at each stimulus duration. Open dots are used for data from measurement and filled squares for those from reproduction. Solid lines are linear fits. Pearson’s correlation coefficient and significance is given in the lower-right corner. Above each panel, cell and task phase is indicated. The averages of firing rate and its change were calculated from the last half of the SDFs in the corresponding task phase.
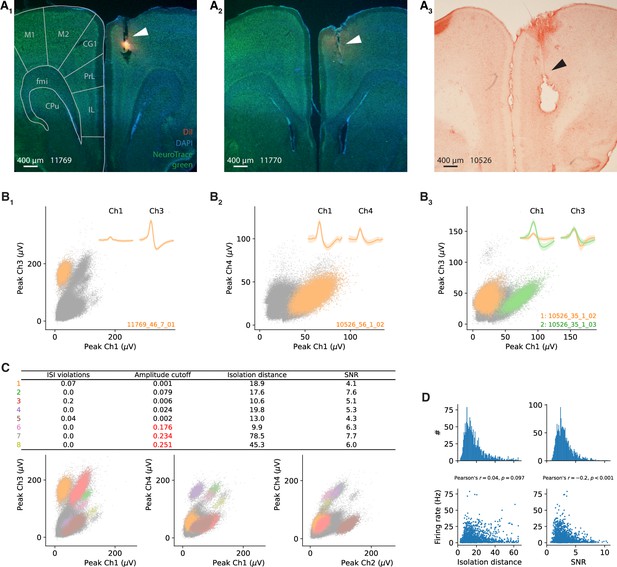
Electrophysiological recordings in gerbil medial prefrontal cortex (mPFC) – histology and spike sorting.
(A) Representative coronal sections of the brains of all three gerbils. Tetrode tracks are visible in right cingulate cortex. In addition, stains from DiI coating of the tetrodes are visible in (A1, A2), and a big postmortem electrolytic lesion in (A3). Background staining was done with DAPI and Neurotracer (A1, A2) and Neutralred (A3). A section from a gerbil brain atlas is overlaid in (A1) (Radtke-Schuller et al., 2016). M1, M2, primary and secondary motor cortex; CG1, cingulate cortex, area 1; PrL, prelimbic cortex; IL, infralimbic cortex; fmi, forceps minor of corpus callosum; CPu, caudate putamen. (B) Spike clusters in feature space of signals recorded at one tetrode; data from three different example sessions. (B1) Cell from Figure 2A in the main text. (B2) cell from Figure 2B in the main text. (B3) cell from Figure 2C (orange) in the main text and Figure 2—figure supplement 3A (green). Peak signals are shown for two different channels of one tetrode as an example projection. Other spikes and voltage deflections are displayed as gray dots. Insets: average waveforms (1 ms length) corresponding to the colored clusters. (C) Three different projections of the feature space from the tetrode recoding in (B1). All eight spike clusters that could be separated are marked (color coded). Other spikes and voltage deflections are again displayed as gray dots. The table lists quality measures for each cluster. Clusters 6–8 had too large amplitude cutoff and were excluded from further analysis. (D) Distributions of isolation distance and signal-to-noise ratio (SNR) for the clusters of all 1766 cells (i.e., excluding clusters/cells of insufficient quality). Both values were weakly correlated with the average firing rate (bottom panels). Isolation distance was calculated as described in Schmitzer-Torbert et al., 2005 for clusters of the peak amplitude (C). SNR was calculated as the ratio of the peak of the average spike waveform to the standard deviation of the background noise, that is, spikes that do not belong to a separated cluster. This was done for every channel individually and then averaged for all channels of a tetrode. Interspike interval (ISI) violations and amplitude cutoff were calculated as described in the Materials and methods section.
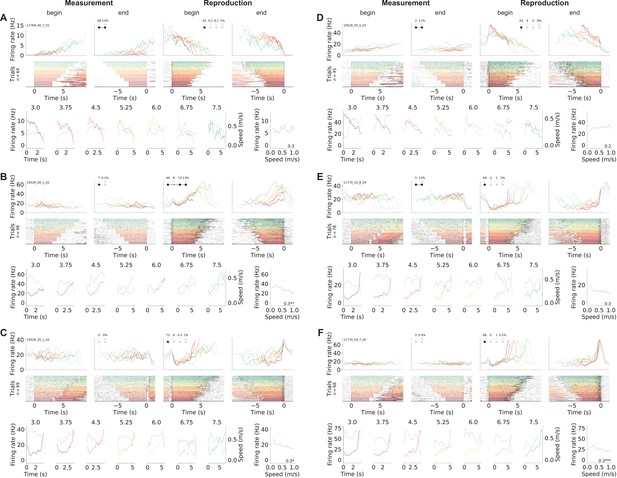
Ramping neurons.
(A–C) Example cells from Figure 2 in the main text. (D) Another cell that linearly increased its firing rate during measurement and ramped down to zero during reproduction. (E) A neuron that responded constantly but somewhat modulated by stimulus duration during measurement and ramps to threshold during reproduction. (F) Another ramp-to-threshold cell. (A–F) Panels display spike rasters sorted by stimulus duration (bottom) and corresponding spike density functions (SDF, top). Each column plots the data with different alignment, measurement begin and end, reproduction begin and end. Color identifies stimulus duration (Figure 1C). In the raster plots, black ticks are single spikes. For better visualization, we only plot half of the spikes (randomly chosen). Measurement or reproduction phases are delimited by underlayed color. The SDFs are colored in the respective task phase, outside they are displayed as thin gray lines. Markers in second and third panels show percent explained variance for each principal component (see Figure 5). Black dots – which may be connected by a line – illustrate the cell type according to categorization (see Figure 6). Bottom panels compare SDF (color coded) to average (virtual) running speed (gray) for each stimulus duration. Bottom-right panels plot the tuning curves for virtual speed (speed response function). Modulation index and significance is given in the lower-right corner.
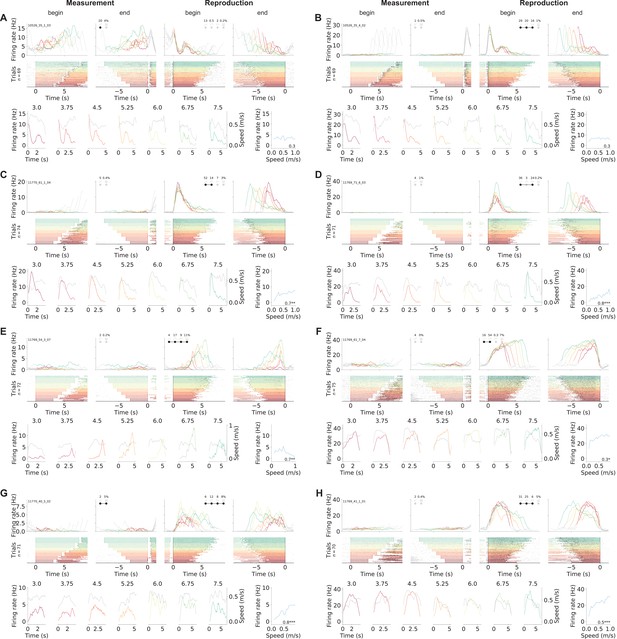
Phasically timing neurons.
(A–H) Examples of neurons that showed phasic responses at specific time points or for a certain duration in the reproduction phase. Note that the cell in (E) signals absolute time as it starts firing at 4 s – and proceeds until shortly before the end of the reproduced interval. (C–H) Neurons with pronounced modulation by (virtual) running speed. Plots are organized as in Figure 2—figure supplement 2.
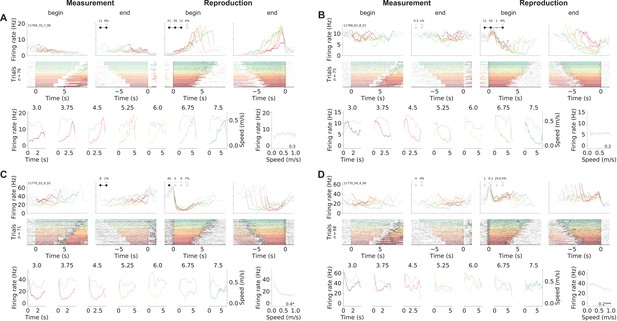
Other example neurons.
(A) A neuron that during reproduction appeared very similar to the cell in Figure 2—figure supplement 3F, but in fact does not signal absolute but relative time as it starts firing later for longer stimuli. (B–D) Cells that during reproduction responded with brief (D) or extended drops of activity (B, C). The amount of activity reduction may (B) or may not (C) have scaled with the stimulus duration. All plots are organized as in Figure 2—figure supplement 2.
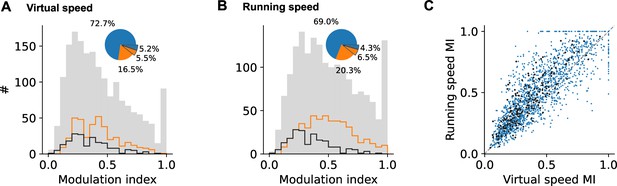
Influence of speed on single neuron responses.
Distributions of modulation indices derived from the speed response functions of each neuron for virtual speed (A) and running speed on the treadmill (B). Neurons with significant speed tuning are delimited by the orange outline (i.e., with variance of their speed response function larger than that of the speed response function for shuffled spike times; significance assessed by Levene’s test p<0.05). Black outline gives histogram for cells with ramp-like responses only (cells explained by PC 1 and those explained by PC 1 + stimulus PC 1, see categorization analysis below and Figure 6). Pie plots show significant (orange) and nonsignificant (blue) fractions. Raised wedges mark ramping neurons. (C) Modulation indices for virtual speed vs. running speed for each neuron (blue dots). Ramping neurons are marked with black dots.
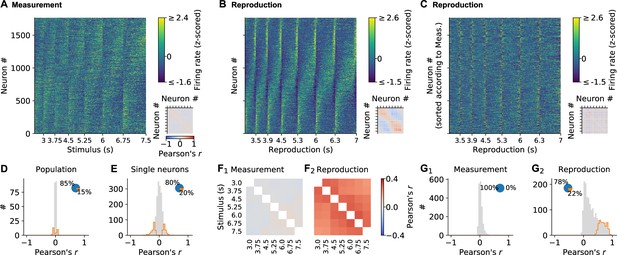
Single neuron and population dynamics in measurement and reproduction.
(A) Normalized (z-scored) spike density functions (SDFs) of all 1766 medial prefrontal cortex (mPFC) neurons for each stimulus interval during the measurement phase sorted by their timing within the intervals. Small panel: matrix of pairwise Pearson correlations between all neurons. Diagonal entries not plotted. (B) Same as (A) but for the reproduction phase. (C) Same as (B) but with neurons sorted as in (A). (D) Population vector correlations in corresponding time bins of measurement and reproduction. (E) Pearson correlations between measurement and reproduction for single neurons. (F) Pairwise correlations of the whole population for different stimuli in measurement (F1) and reproduction (F2). Diagonal entries not plotted. (G) Distributions of average Pearson correlations of single-cell activity for different stimuli in measurement (G1) and reproduction (G2). Histograms in (D, E, G) are displayed in gray with significant values (p<0.05) delimited by an orange outline. Pie plots show significant (orange) and nonsignificant (blue) percentages.
-
Figure 3—source data 1
Spike density function (SDF) source data for Figure 3A and B and all upcoming analyses and figures based on SDFs.
The zip also includes Python source code to load the hdf5 data.
- https://cdn.elifesciences.org/articles/71612/elife-71612-fig3-data1-v2.zip
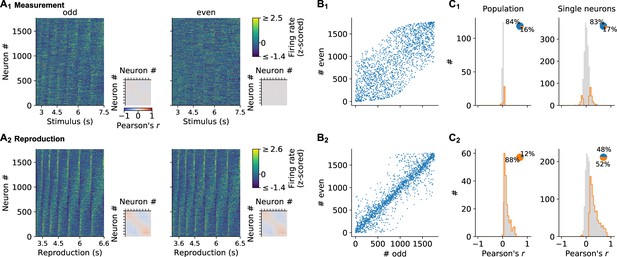
Stability of neuronal responses.
(A) Normalized (z-scored) spike density functions (SDFs) of all neurons for each stimulus interval during (A1) measurement and (A2) reproduction sorted by their timing within the intervals for odd trials; same sorting in (A1) and (A2). The left side displays data for odd trials only; the right panel for even trials. Small panels: matrices of pairwise Pearson correlations between all neurons. Diagonal entries not plotted. (B) Sorting indices assigned to the neurons if sorting would be based only on activity during odd or even trials. The closer a dot appears at the bisecting line, the better sorting corresponds between odd and even trials. During reproduction, activity corresponds well, arguing for stable responses throughout a recording session. During measurement activity is less similar. This has to be attributed to less pronounced and noisier responses in this task phase and not unstable recordings since measurement and reproduction are interleaved throughout the session. (C) Correlations between odd and even trials give a similar picture. Histograms plot Pearson’s correlation coefficients during (C1) measurement and (C2) reproduction of the population in each time bin (left) and for single neurons (right). Histograms are displayed in gray with significant values delimited by an orange outline (p<0.05). Pie plots show significant (orange) and nonsignificant (blue) percentages.
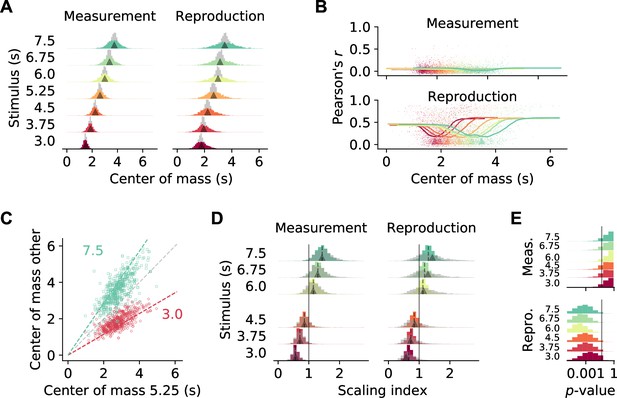
Temporal scaling.
(A) Distributions of center of mass for all 1766 medial prefrontal cortex (mPFC) neurons (colored histograms). Gray histograms give distributions of center of mass for noise spike density functions (SDFs). Arrowheads mark the middle of the stimulus interval and of the average reproduced interval, respectively. (B) During reproduction, the average Pearson correlations of single-cell activity for different stimuli (Figure 3F) were larger for neurons with center of mass at the begin or end of an interval. Dots give single-cell data, solid lines are moving averages. Arrowheads in the lower panel mark the middle of the average reproduced interval. (C) Center of mass of each cell for 5.25 s against 3 s (red) and 7.5 s (green) during reproduction. Colored dashed lines mark the predicted ratio; gray dashed line corresponds to no change. (D) Distributions of scaling indices for all cells, that is, center of mass of the SDF at every stimulus divided by the center of mass for 5.25 s. Solid vertical line marks 1. Dashed lines mark the medians and arrow heads the ratio between 5.25 s and the respective other stimulus duration. Gray histograms give distributions of center of mass for shuffled control SDFs. (E) Bootstrapped distributions of p-values for Kolmogorov–Smirnov tests between scaling indices for recorded and shuffled SDFs. Solid vertical line marks 5%. Logarithmic x-axis.
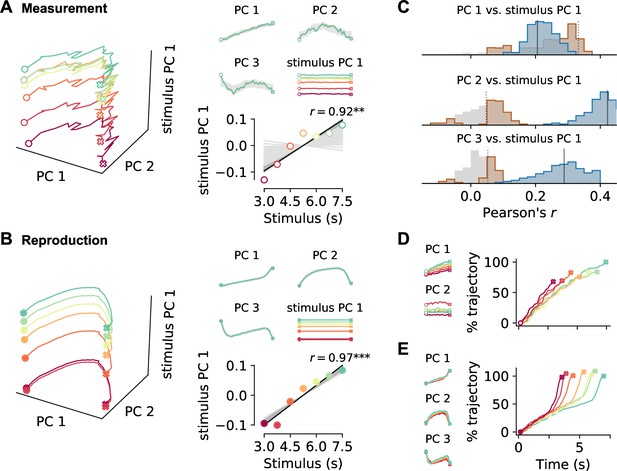
Decomposition of population activity.
Demixed principal component analysis (PCA) yields separate principal components (PCs) for the time course during a trial (PCs 1–3) and for each stimulus duration (stimulus PC 1). (A) PCs for measurement and (B) for reproduction. The small panels display individual PCs at each stimulus interval scaled to the length of the interval. Note that PCs 1–3 for different stimuli lie on top of each other, demonstrating perfect demixing. Stimuli are colored as in previous figures. Circles and crosses mark interval start and end. Open symbols are used for measurement and filled for reproduction. The PCs were calculated for the whole data set and gray-shaded areas delimit the standard deviation of results from bootstrapping with 10% of the cells. Bottom-right panels in (A) and (B): correlation of stimulus PC 1 with the stimulus duration. Black solid line is a linear fit, and gray lines are fits for the bootstrap samples; dark gray significant, light gray nonsignificant cases. (C) Distributions of Pearson’s correlation coefficients between PCs 1–3 and stimulus PC 1 from bootstrap samples. Significant correlations are colored in brown (measurement) and blue (reproduction). Dotted (measurement) and solid (reproduction) black lines mark coefficients for the whole data set. (D, small panels) PCs for conventional PCA during measurement scaled to the length of the interval for each stimulus. Note that, in contrast to demixed PCA, conventional PCs contain both time course-related and stimulus information. (D, large panel) Time course of PC 1 for conventional PCA at each stimulus during measurement. The percentage of covered trajectory is given with respect to PC 1 for the 7.5 s stimulus. (E) Same as (D) for reproduction.
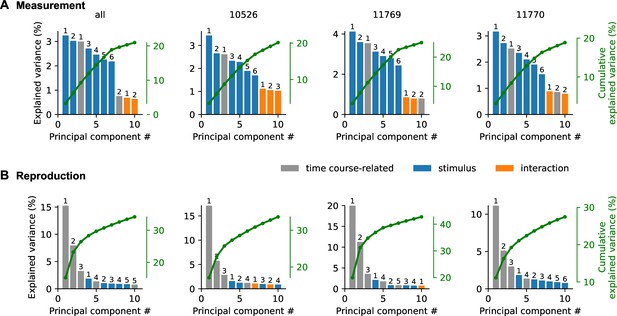
Explained variance of demixed principal component analysis (PCA).
Panels show explained variance for each principal component (bar graphs) and their cumulative explained variance (green line) of demixed PCA for measurement (A) and reproduction (B). Different panels correspond to all or individual animals. Numbers above bars give order with regard to component type, that is, time course-related (gray), stimulus duration (blue), or interaction between both (orange). Results are comparable for individual animals and when pooled across all animals. In measurement, the first time course-related and stimulus-dependent PCs are strongest. Reproduction is best explained by the first three time course-related PCs; only at fourth position stimulus components start contributing.
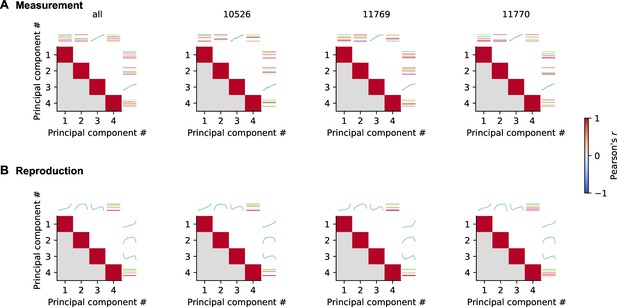
Proper demixing of principal components (PCs) by demixed principal component analysis (PCA).
(A) Each panel shows correlations between all pairs of the first four demixed PCs for measurement. At top and to the left, the PCs are illustrated. Different stimuli are color coded as in other figures. Different panels give results for pooled data (all) and individual animals. (B) Same as (A) for reproduction. The correlations between different PCs are zero, indicating proper demixing.
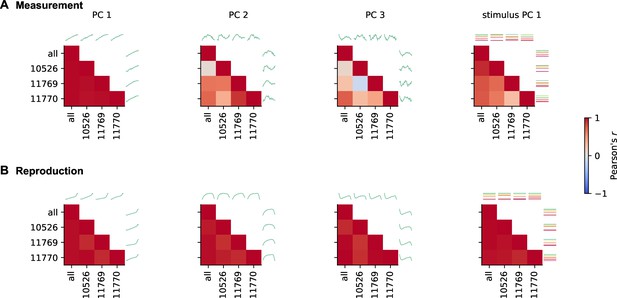
Demixed principal component analysis (PCA) results are similar across animals.
(A) Individual panels show correlations between all pairs of demixed principal components (PCs) calculated for pooled data (all) and single animals for the measurement phase. At top and to the left, the PCs are illustrated. Stimuli are color coded. Different panels give time course-related PCs 1-3 and stimulus-related PC 1. (B) Same as (A) for reproduction. PCs are highly correlated across animals.
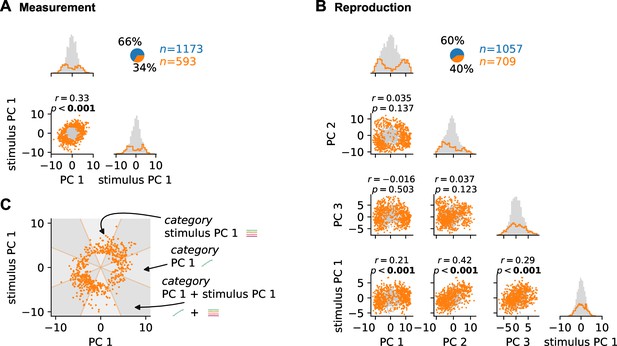
Distributions of and correlations between demixed principal component analysis (PCA) scores.
(A) The scatter plot shows the correlation between scores for time course-related principal component (PC) 1 and stimulus PC 1 in the measurement phase. Pearson’s and -value are listed above the scatter plot. Both were calculated for all cells. Histograms give distributions for the PCs. Cells that can be explained by the two PCs are plotted in orange, all others in gray. Pie plot shows fractions of cells that can (orange) and cannot (blue) be explained by the PCs. (B) Same as (A) for reproduction and for time course-related PCs 1-3 and stimulus PC 1. (C) Categorization procedure at the example of the measurement data in (A). Categories were determined only for cells that could be sufficiently explained by the PCs. Cells were counted as explained by a PC if the ratio of scores (in absolute values) to the other category was below tan(67.5°). For the measurement phase, this results in three different categories: PC 1 only, stimulus PC 1 only, or PC 1 and stimulus PC 1 delineated by the three types of wedges in the plot.
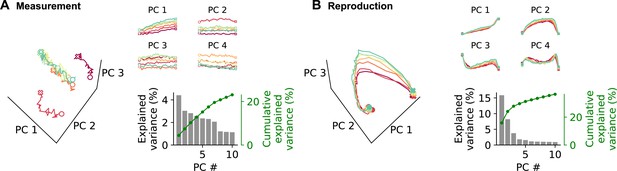
Decomposition with conventional principal component (PC) analysis.
First four PCs for (A) measurement and (B) reproduction. Stimuli are colored as in other figures. Circles and crosses mark interval start and end. Open symbols are used for measurement and filled for reproduction. Bottom-right panels: explained variance for each PC (bar graphs) and their cumulative explained variance (green line).
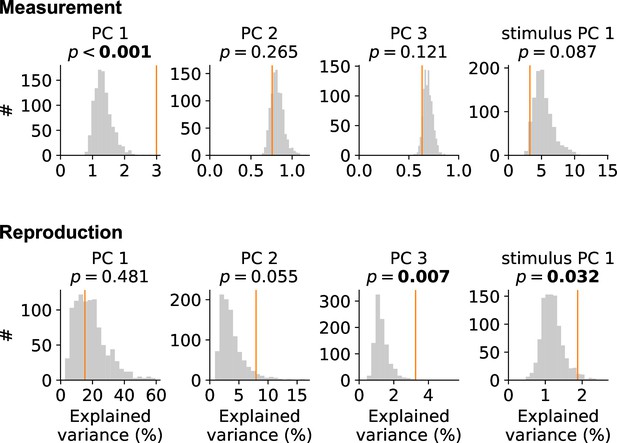
Collective activity adds to principal components (PCs).
We drew 1000 random tensor maximum entropy surrogate samples (Elsayed and Cunningham, 2017a) for measurement (upper panels) and reproduction (lower panels) and determined the explained variance of time course-related components PCs 1–3 and stimulus PC 1 (gray histograms). Orange vertical lines mark values for the real data. During measurement, PC 1 for the data was larger than expected. Similarly, PC 3 was larger than expected during reproduction. Stimulus PC 1 was larger than expected for reproduction.
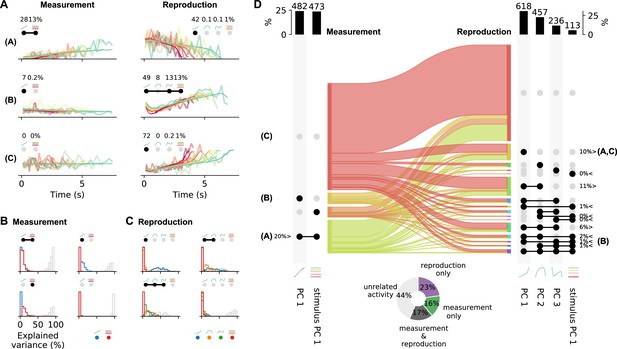
Single-cell activity and their contribution to the population response change from measurement to reproduction.
(A) Reconstructions (thin lines) of the firing patterns (spike density functions [SDFs], thick faint lines) of the example neurons from Figure 2 (identified by bold letters; note that here SDFs were not smoothed). Markers show percent explained variance for each principal component (PC) and illustrate the response type according to the categorization (see D). For instance, the neuron in the first row is reconstructed by the linear combination of PC 1 and stimulus PC 1 during measurement and with (negative) PC 1 during reproduction. (B) Distributions of variance of single-cell responses explained by PCs during measurement. Variance explained by PC 1 is displayed in blue and by stimulus PC 1 in red. The remainder (i.e., variance unexplained) is displayed as gray open bars. Each panel displays distributions for cells belonging to one of the four possible response categories visualized by the markers above the panel. (C) Same as (B) for the reproduction phase. Here the coloring is PCs 1–3, blue, orange, green; stimulus PC 1, red. Distributions are displayed for the four categories with the most cells. (D) Transition between response categories from measurement to reproduction (Sankey diagram). At the margins the numbers of cells are given with activity patterns that can be reconstructed by PCs. For measurement (left), PC 1 and stimulus PC 1 were considered and for reproduction (right) PCs 1–3 and stimulus PC 1. Dark dots indicate the contribution of a PC to a response category. Percentages are only given for categories that contain significant numbers of cells compared to shuffled data. The signs < and > indicate if the number is smaller or larger than for shuffled data. Zero percentages mean less than 1%. Bold letters correspond to the examples from (A).Bar graphs at the top show percentages and cell numbers with contributions of each PC. Pie chart at bottom shows percentages of cells active during the task phases determined from the reconstructions.
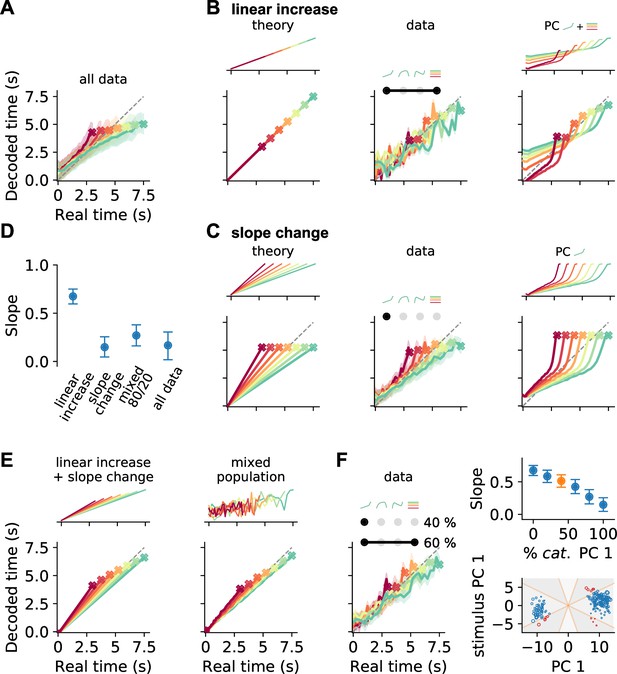
Decoding elapsed time from prefrontal population activity.
(A) Elapsed time (average ± standard deviation from bootstrapping) decoded from the responses of all neurons recorded during the reproduction phase. As before, color identifies stimulus duration. Crosses mark final values. A strong regression effect is visible. (B) Time decoding with neurons that ramp to stimulus-dependent levels but with the same slope and (C) with ramp-to-threshold cells. Left panels illustrate the theoretical prediction for decoded time and example neuronal activity above. Middle panels plot decoding results using only neurons from the respective response category. Right panels give results for decoding using the corresponding components from demixed principal component analysis (PCA). As displayed in the top panels, these components are principal component (PC) 1 and stimulus PC 1 in (B); and PC 1 only in (C). (D) Median slopes of the linear regression between the final values of real and predicted time for decoding from data in (A–C) and for a mixture of 80% slope-changing and 20% linear increasing cells, which aligns well with decoding from all data (A); regarding mixtures see also (F). Error bars delimit interquartile ranges (from bootstrapping). (E) Mixtures of linear increasing activity and slope changes explain behavioral regression effects. Theoretical predictions for mixing both responses in single neurons (left) or as two different response types across a population (right). For the second case, a neuron with noisy linear increasing activity is displayed as an example. (F) Decoding results for a mixed population of 40% slope-changing and 60% linear increasing cells. The cells were sampled at these fractions in each bootstrapping run from the response categories we identified in our recorded data. The upper-right panel shows the regression slope (D) for different fractions of slope-changing cells. The orange marker corresponds to the example in the left panel. The lower-right panel displays the PCA scores of the cells from the linear increasing (red; PC 1 + stimulus PC 1) and slope-changing categories (blue; PC 1 only). The size of the marker illustrates the decoder weight for that cell. See also Figure 5—figure supplement 4C.

Decoding elapsed time from data, shuffled data, and noise.
Decoding results from the whole population, cells with sufficiently large dPCA scores, cells with small dPCA scores (‘unrelated activity’), shuffled data and noise during the reproduction phase. Each panel displays decoded time vs. the real time for each stimulus (color coded); average ± standard deviation (from bootstrapping). Crosses mark final values. Number of neurons is given in the lower-right corner. Rightmost panels display slopes and indifference points of linear regression between final values of real and predicted time for the five data sets. At the indifference point, real and decoded final time match. We calculated it from the slope and intercept of the regression line as . The indifference point estimates the amount of general over-/underestimation. With a regression effect, the indifference point should be at the center of the stimulus distribution (5.25 s), which we only obtain when decoding from all data or the cells with large principal component analysis (PCA) scores.
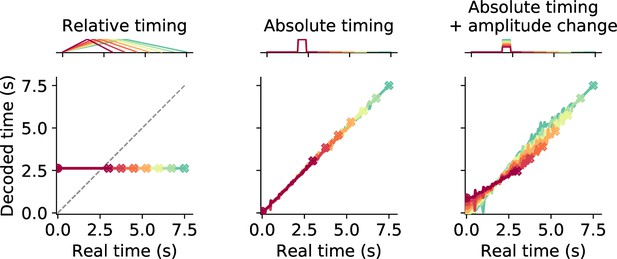
Decoding time from phasically active neurons.
Decoding results for three different theoretical response types with phasic activation. The uppermost panel in each column shows example neuronal activity and the panel below gives the prediction for decoded time. Different stimulus intervals are color coded. Crosses mark final values. Relative timing neurons peak at the center of an interval. Absolute timing neurons peak at a specific time point. In addition, stimulus interval may be coded in the response amplitude (absolute timing + amplitude change). Although only one example is displayed, for both types of absolute timing neurons the population comprises cells peaking at different time points such that the whole interval is tiled. Relative timing neurons can only encode a single time point, whereas absolute timing neurons allow for precise time encoding.
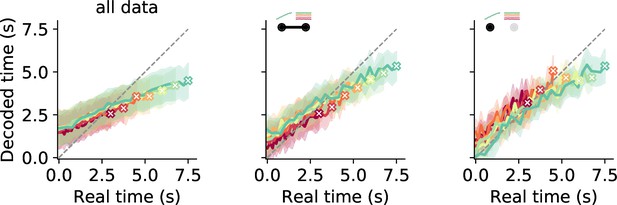
Decoding time during measurement.
Results are displayed for all data (left), cells in the principal component (PC) 1 + stimulus PC 1 category, and cells in the PC 1-only category. For all data, decoding is imprecise with initial overestimation and underestimation at the end of the interval. For PC 1 + stimulus PC 1 cells as well as cells in the PC 1-only category, decoded time starts out more precisely but ends up at a general underestimation for the first response type. However, a regression effect with an overestimation for small stimuli is visible in the second case.