An open-source tool for automated analysis of breathing behaviors in common marmosets and rodents
Figures
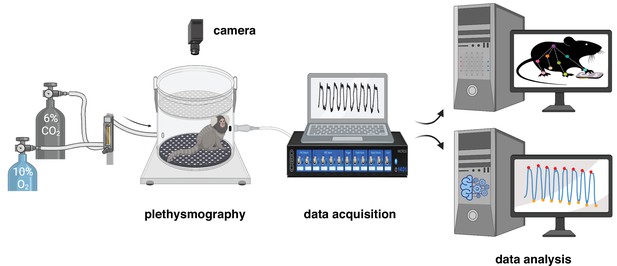
Experimental design for measurement and analysis of marmoset respiratory behaviors.
After a 40 min baseline period at room air (21% O2, ~0% CO2, and 79% N2), the breathing behavior of the animal was studied under either hypoxic (10% O2; 10 min) or hypercapnic (6% CO2; 10 min) conditions. Raw respiratory signal is later cleaned and analyzed offline (see Materials and methods for details). Video of spontaneous activity in the chamber at baseline and during each challenge was used to train a DeepLabCut model to track the animal body.
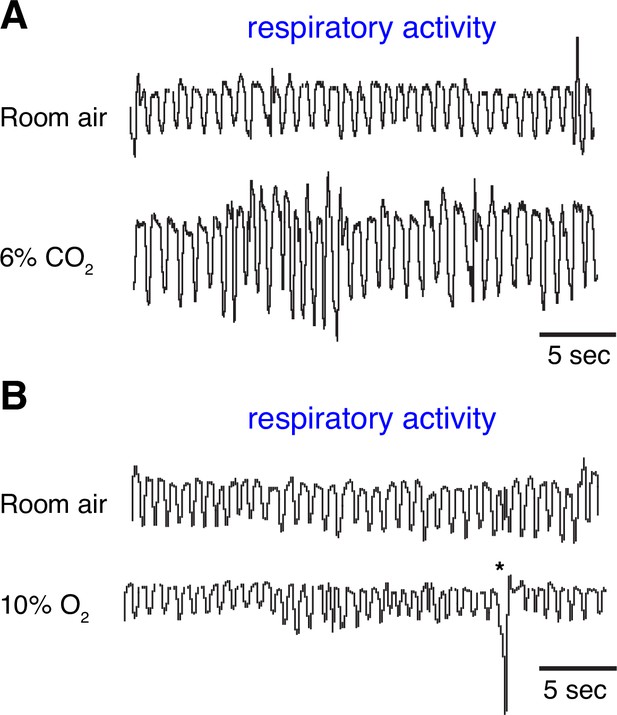
Breathing behaviors in adult marmoset.
Representative raw respiratory traces at baseline and following 5 min exposure to hypercapnia (A) and hypoxia (B) conditions. * represents a sigh breathing.
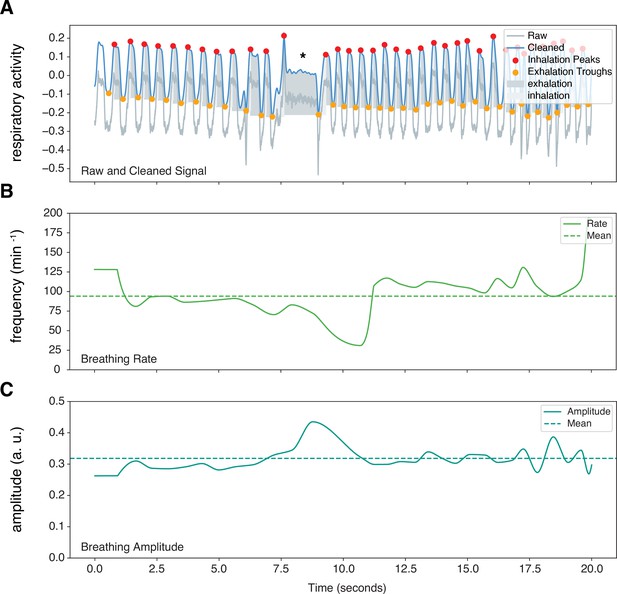
Sample marmoset respiratory trace output from Neurokit2.
Representative respiratory trace is sampled from a single male marmoset during hypercapnia challenge. (A) NeuroKit2 was used for signal detrending and smoothing, peak and trough extraction, as well as respiratory phase. (B and C) Instantaneous measurement of breathing frequency (fR) (B) and breathing amplitude (VT) (C) are illustrated. This sample also contained respiratory changes during a phee call (marked by *). a. u. – arbitrary unit.
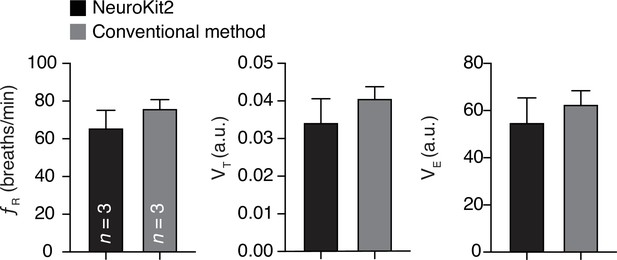
Validation of our tool in analysis of breathing behaviors in common marmoset.
Our analysis tool was bench marked to the conventional method for studying of respiratory frequencies (fR), tidal volumes (VT), and minute ventilations (VE) at baseline (i.e., at room air). Data are shown as mean rate ± SEM. a. u. – arbitrary unit.
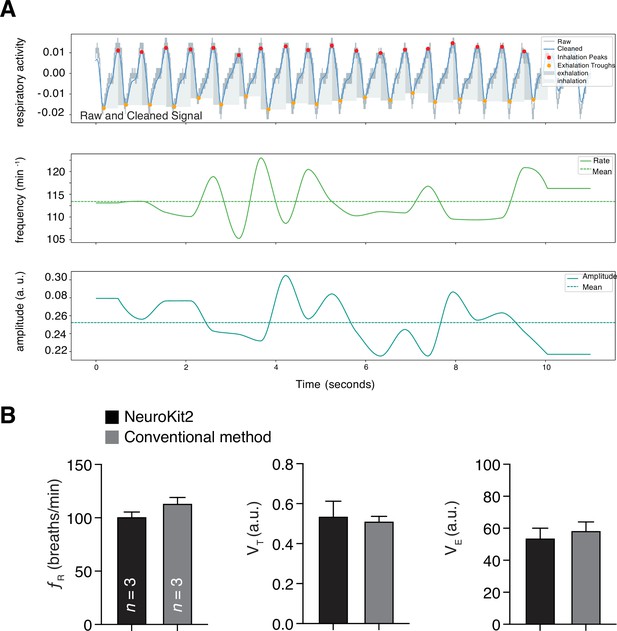
Analysis of rodent respiratory behaviors.
The representative respiratory trace is sampled from a single male rat breathing room air. (A) NeuroKit2 was used for signal detrending and smoothing, peak and trough extraction, as well as respiratory phase. Instantaneous measurement of breathing frequency (fR) (middle) and breathing amplitude (VT) (lower) is illustrated. (B) Validation of our tool in analysis of breathing behaviors in rodents. Our analysis tool was bench marked to the conventional method for studying of respiratory frequencies (fR), tidal volumes (VT), and minute ventilations (VE) at baseline (i.e., at room air) in rats. Data are shown as mean rate ± SEM. a. u. – arbitrary unit.
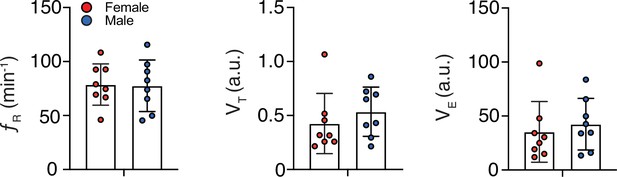
Sex differences in baseline respiratory frequencies.
Baseline (i.e., at room air) respiratory frequencies (fR), tidal volumes (VT), and minute ventilations (VE) were not different between female (n = 8) and male (n = 8) marmosets. Data are shown as mean rate ± SEM. a. u. – arbitrary unit.
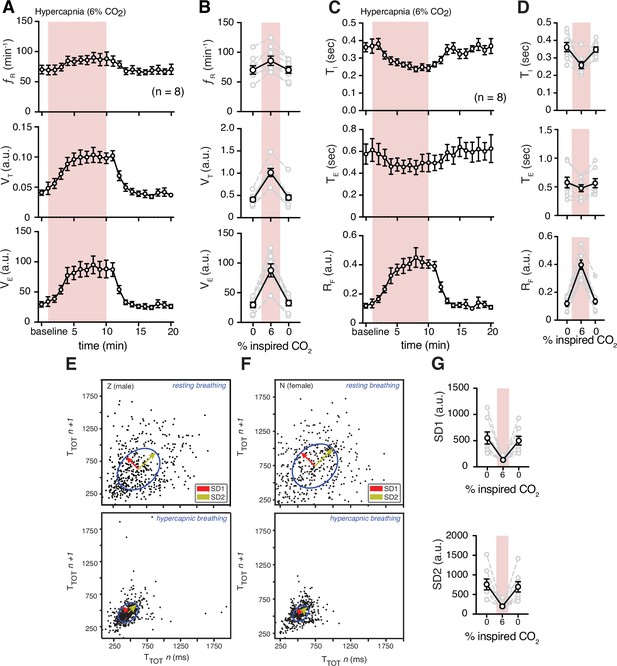
Hypercapnia challenge-induced changes in respiratory features.
(A) Measurements of breathing rate (fR), tidal volume (VT), and minute ventilation (VE) were averaged across 1 min epochs for assessment of local changes in each parameter. (B) Summaries of each feature at baseline, following 5 min exposure to hypercapnia, and in the 5 min immediately following the end of challenge. We observed increases in respiratory frequency (p = 0.023, Wilcoxon matched-pairs signed rank test), VT (p = 0.008, Wilcoxon matched-pairs signed rank test), and VE (p = 0.008, Wilcoxon matched-pairs signed rank test) during hypercapnia. (C) Measurements of inspiratory time (TI), expiratory time (TE), and respiratory drive (RD) were averaged across 1 min epochs for assessment of local changes in each parameter. (D) Summaries of each feature at baseline (0% inspired CO2), following 5 min exposure to 6% hypercapnia, and in the first 5 min following the end of hypercapnic challenge. During hypercapnia, we observed decreases in TI (p = 0.008, Wilcoxon matched-pairs signed rank test), TE (p = 0.078, Wilcoxon matched-pairs signed rank test), and increase in RD (p = 0.008, Wilcoxon matched-pairs signed rank test). Representative Poincaré plots of total cycle duration (TTOT) for the nth cycle vs. TTOT for the nth+1 cycle during baseline (room air) and hypercapnic (6% CO2) conditions in male (E) and female (F) marmosets. (G) Grouped data illustrating changes in SD1 and SD2 before, during, and after hypercapnia challenge. Respiratory rate variability decreased in both measures during hypercapnia compared to baseline (SD1: p = 0.008; SD2: p = 0.008; Wilcoxon matched-pairs signed rank test). In B, D, and G, data are shown as individual (gray lines) and mean values ± SEM (black line). a. u. – arbitrary unit.
-
Figure 3—source data 1
Hypercapnia challenge source data.
- https://cdn.elifesciences.org/articles/71647/elife-71647-fig3-data1-v2.xlsx
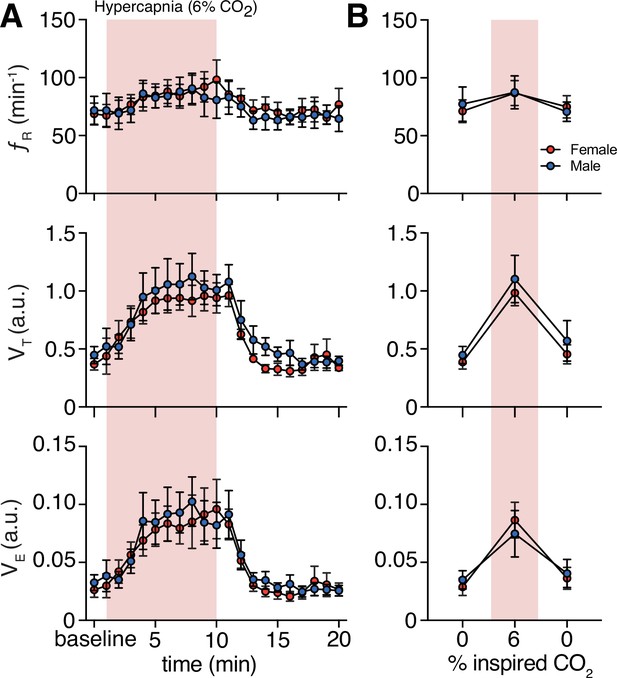
Hypercapnia challenge-induced changes in respiratory behavior by sex.
(A) Measurements of breathing rate (fR), tidal volume (VT), and minute ventilation (VE) were averaged across 1 min epochs for assessment of local changes in each parameter. (B) Summaries of each feature at baseline, following 5 min exposure to hypercapnia, and in the 5 min immediately following the end of challenge. We saw no sex differences in fR, VT, or VE in room air (p = 0.99, p = 0.89, and p = 0.49, respectively; n = 4 animals per sex; Mann–Whitney U rank test), during hypercapnia challenge (p = 0.99, p = 0.89, and p = 0.77, respectively; n = 4 animals per sex; Mann–Whitney U rank test), and post-challenge (p = 0.69, p = 0.99, and p = 0.99, respectively; n = 4 animals per sex; Mann–Whitney U rank test). Data are shown as mean values ± SEM. a. u. – arbitrary unit.
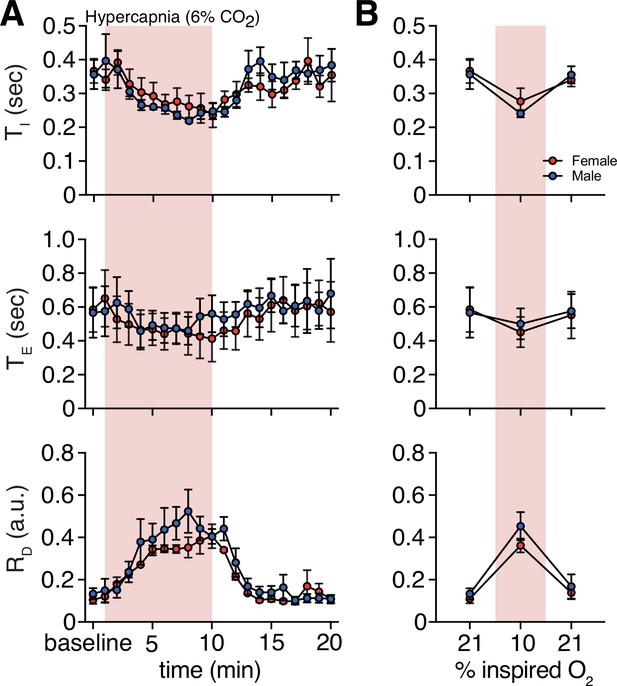
Hypercapnia challenge-induced changes in respiratory features by sex.
(A) Measurements of inspiratory time (TI), expiratory time (TE), and respiratory drive (RD) were averaged across 1 min epochs for assessment of local changes in each parameter. (B) Summaries of each feature at baseline, following 5 min exposure to challenge until end of challenge, and in the 5 min immediately following the end of challenge. We saw no sex differences in TI, TE, or RD in room air (p = 0.99, p = 0.89, and p = 0.34, respectively; n = 4 animals per sex; Mann–Whitney U rank test), during hypercapnia challenge (p = 0.89, p = 0.89, and p = 0.49, respectively; n = 4 animals per sex; Mann–Whitney U rank test), and post-challenge (p = 0.89, p = 0.89, and p = 0.99, respectively; n = 4 animals per sex; Mann–Whitney U rank test). Data are shown as mean values ± SEM. a. u. – arbitrary unit.
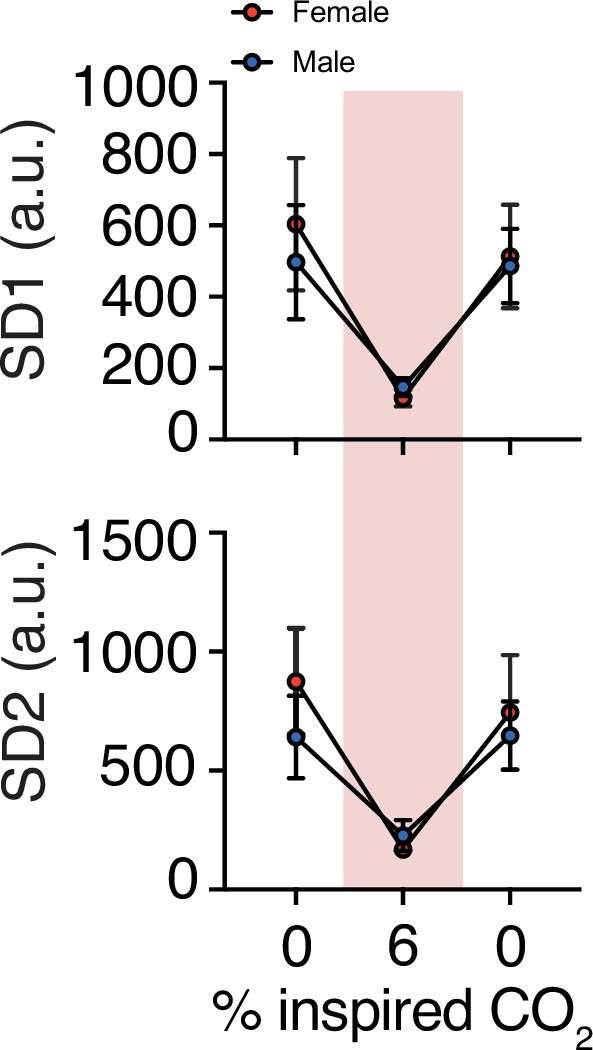
Changes in variability of respiration during hypercapnic challenge.
Hypercapnia challenge-induced changes in respiratory rate variability by sex. We observed no sex differences in SD1 or SD2 at baseline (p = 0.49, p = 0.49, respectively; n = 4 animals per sex; Mann–Whitney U rank test), during hypercapnia challenge (p = 0.20, p = 0.34), or immediately following the challenge (p = 0.99, p = 0.99, respectively; n = 4 animals per sex; Mann–Whitney U rank test).
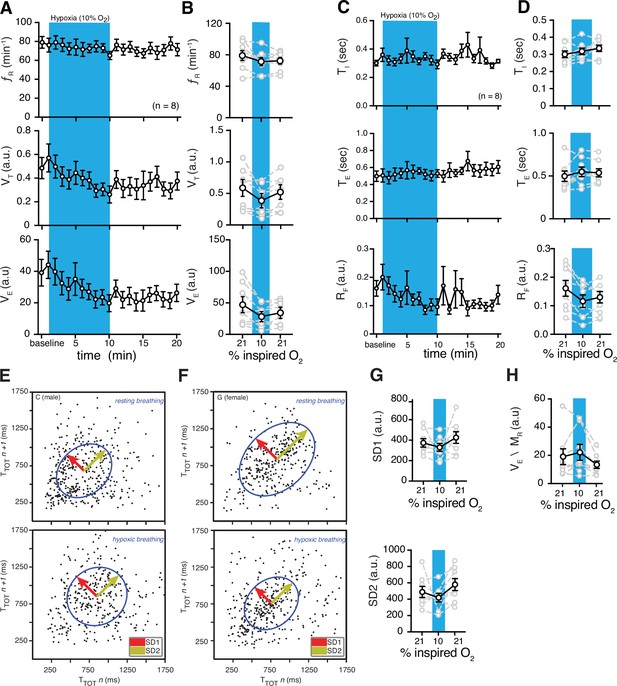
Hypoxic and post-hypoxic challenge-induced changes in respiratory features.
(A) Measurements of breathing rate (fR), tidal volume (VT), and minute ventilation (VE) were averaged across 1-min epochs for assessment of local changes in each parameter. (B) Summaries of each feature at baseline, following 5 min exposure to hypoxic (10% O2) challenge, and in the 5 min immediately following the end of challenge. During hypoxia challenge, we saw no changes in respiratory frequency (p = 0.31, Wilcoxon matched-pairs signed rank test) and VE (p = 0.11, Wilcoxon matched-pairs signed rank test) compared to baseline. VT decreased during hypoxia challenge (p = 0.078, Wilcoxon matched-pairs signed rank test). Immediately following the challenge, we saw no changes in respiratory frequency (p = 0.11, Wilcoxon matched-pairs signed rank test) compared to baseline, and a post-challenge decrease in VT (p = 0.078, Wilcoxon matched-pairs signed rank test) and VE (p = 0.078, Wilcoxon matched-pairs signed rank test) compared to baseline. (C) Measurements of inspiratory time (TI), expiratory time (TE), and respiratory drive (RD) were averaged across 1 min epochs for assessment of local changes in each parameter. (D) Summaries of each feature at baseline, following 5 min exposure to challenge until end of challenge, and in the 5 min immediately following the end of challenge. During hypoxic challenge, we saw no changes in respiratory TI (p = 0.5) or TE (p = 0.4), but a decrease in RD during (p = 0.008, Wilcoxon matched-pairs signed rank test) compared to baseline. We did observe post-hypoxic challenge increase in TI (p = 0.055) and RD (p = 0.023) and no change in TE (p = 0.46, Wilcoxon matched-pairs signed rank test). Representative Poincaré plots of total cycle duration (TTOT) for the nth cycle vs. TTOT for the nth+1 cycle during baseline and hypoxic conditions (10% O2) in male (E) and female (F) marmosets. (G) Summary data illustrating changes in SD1 and SD2 before, during, and after hypoxic challenge. Respiratory rate variability did not change for either measure during (SD1: p = 0.4; SD2: p = 0.6; Wilcoxon matched-pairs signed rank test) or after (SD1: p = 0.6; SD2: p = 0.3; Wilcoxon matched-pairs signed rank test) the hypoxic challenge compared to baseline. (H) Group data illustrating changes in ventilatory efficiency (VE/MR) before, during, and after hypoxic challenge. Ventilatory efficiency was not affected by acute hypoxia (p = 0.6, Wilcoxon matched-pairs signed rank test), however, it was lower during the post-hypoxic challenge (p = 0.055, Wilcoxon matched-pairs signed rank test). In B, D, G, and H, data are shown as individual (gray lines) and mean values ± SEM (black line). a. u. – arbitrary unit.
-
Figure 4—source data 1
Hypoxia challenge source data.
- https://cdn.elifesciences.org/articles/71647/elife-71647-fig4-data1-v2.xlsx
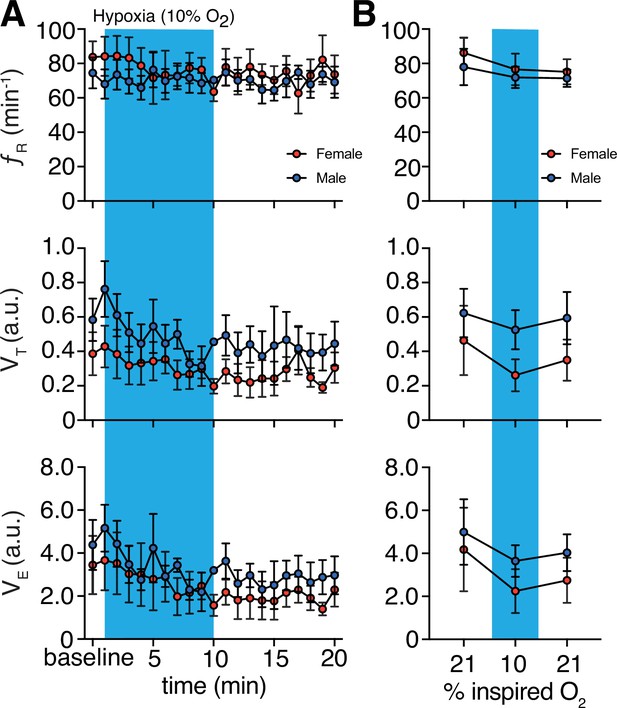
Hypoxic challenge-induced changes in respiratory behavior by sex.
(A) Measurements of breathing rate (fR), tidal volume (VT), and minute ventilation (VE) were averaged across 1 min epochs for assessment of local changes in each parameter. (B) Summaries of each feature at baseline, following 5 min exposure to hypoxic challenge, and in the 5 min immediately following the end of challenge. We saw no sex differences in fR, VT, and VE in room air (p = 0.69, p = 0.89, and p = 0.69, respectively; n = 4 animals per sex; Mann–Whitney U rank test), during hypoxia challenge (p = 0.69, p = 0.20, and p = 0.34, respectively; n = 4 animals per sex; Mann–Whitney U rank test), and post-challenge (p = 0.69, p = 0.37, and p = 0.49, respectively; n = 4 animals per sex; Mann–Whitney U rank test). Data are shown as mean values ± SEM. a. u. – arbitrary unit.
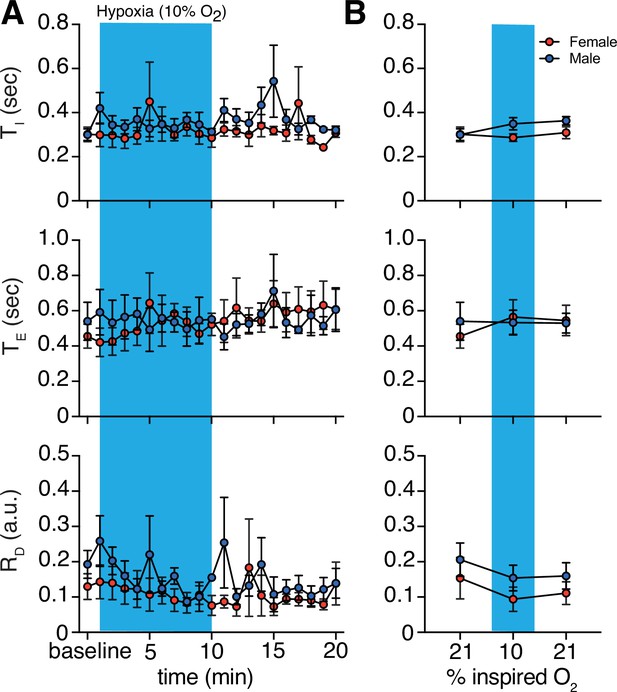
Hypoxic challenge-induced changes in respiratory features by sex.
(A) Measurements of inspiratory time (TI), expiratory time (TE), and respiratory drive (RD) were averaged across 1 min epochs for assessment of local changes in each parameter. (B) Summaries of each feature at baseline, following 5 min exposure to challenge until end of challenge, and in the 5 min immediately following the end of challenge. We saw no sex differences in TI, TE, or RD in room air (p = 0.99, p = 0.49, and p = 0.69, respectively; n = 4 animals per sex; Mann–Whitney U rank test), during hypoxia challenge (p = 0.11, p = 0.99, and p = 0.49, respectively; n = 4 animals per sex; Mann–Whitney U rank test), and post-challenge (p = 0.34, p = 0.99, and p = 0.49, respectively; n = 4 animals per sex; Mann–Whitney U rank test). Data are shown as mean values ± SEM. a. u. – arbitrary unit.
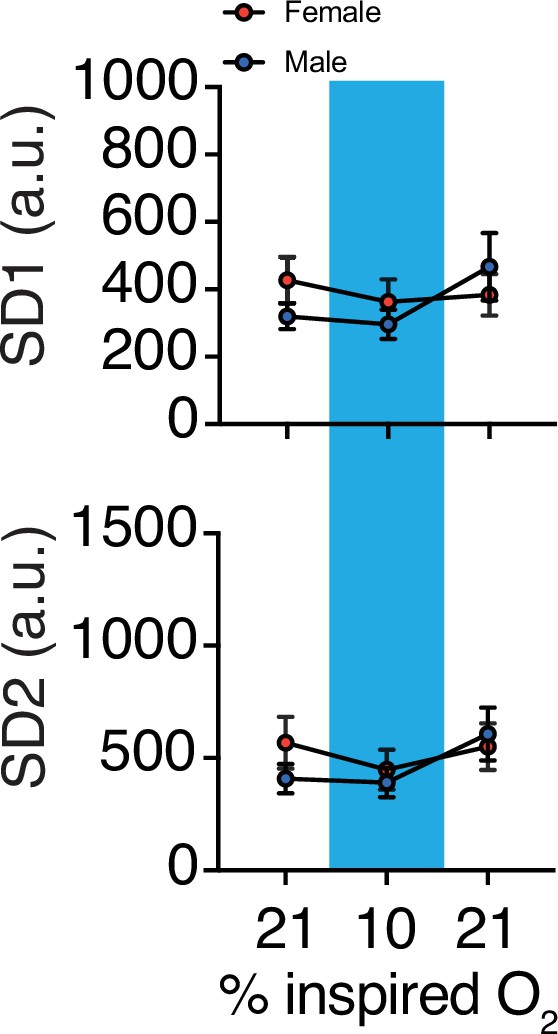
Changes in variability of respiration during hypoxic challenge.
(A) Hypoxia challenge-induced changes in respiratory rate variability by sex. We observed no sex differences in SD1 or SD2 at baseline (p = 0.34, p = 0.34, respectively; n = 4 animals per sex; Mann–Whitney U rank test), during hypercapnia challenge (p = 0.49, p = 0.89, respectively; n = 4 animals per sex; Mann–Whitney U rank test), or immediately following the challenge (p = 0.89, p = 0.89, respectively; n = 4 animals per sex; Mann–Whitney U rank test).
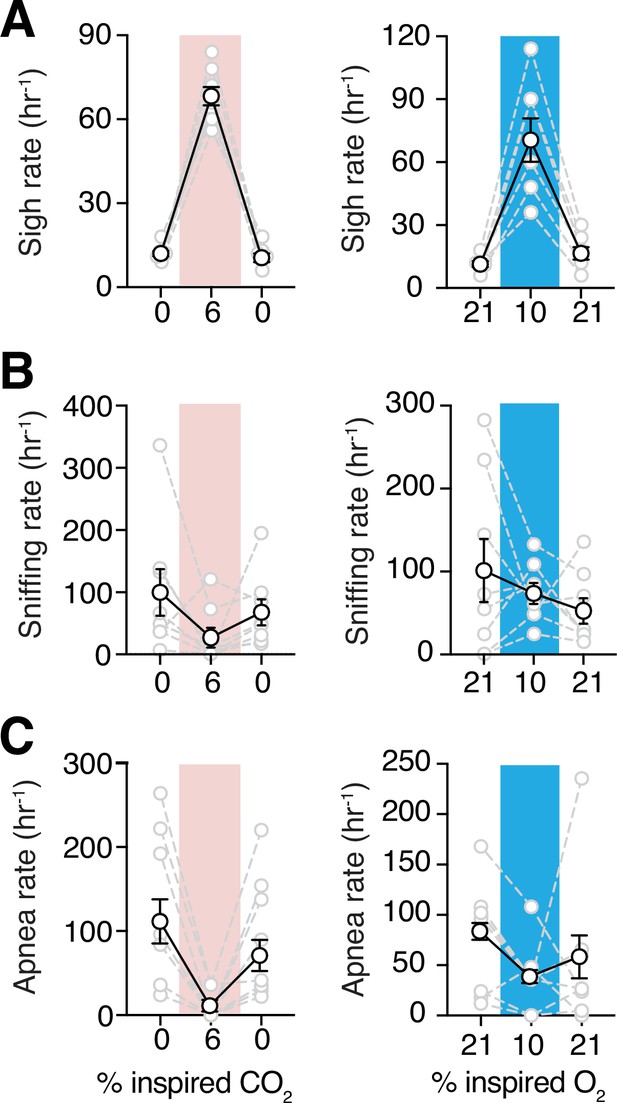
Sigh frequencies, sniffing rate, and apnea index during hypoxia and hypercapnia challenges.
(A) Summary data demonstrating increase in sigh frequency after 5 min of hypoxic (10% O2, left) or hypercapnic (6% CO2, right) challenge (p = 0.008 and p = 0.008, respectively; Wilcoxon matched-pairs signed rank test). (B) Summary data demonstrating no change in sniffing rate during (p = 0.74) and after (p = 0.74) hypoxia challenge (left). Sniffing rate increased during and returned to baseline after hypercapnia challenge (p = 0.008 and p = 0.08 respectively; Wilcoxon matched-pairs signed rank test) (right). (C) Grouped data demonstrating a decrease in rate of spontaneous apneas during hypoxia (p = 0.04, Wilcoxon matched-pairs signed rank test) (left) and hypercapnia (p = 0.008, Wilcoxon matched-pairs signed rank test) (right). Data are shown as individual (gray lines) and mean (black line) values ± SEM.
-
Figure 5—source data 1
Breathing behaviors source data.
- https://cdn.elifesciences.org/articles/71647/elife-71647-fig5-data1-v2.xlsx
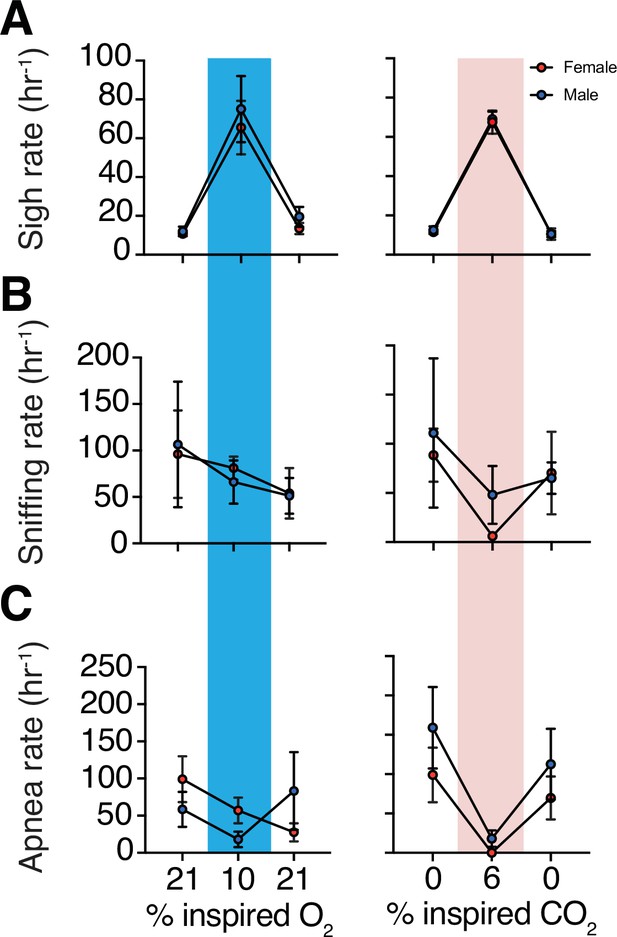
Augmented breath frequencies during hypoxia and hypercapnia challenges by sex.
(A) We observed no sex differences in sigh frequency in animals exposed to hypoxia at baseline (p = 0.97), during challenge (p = 0.66), and following the challenge (p = 0.4; n = 4 animals per sex; Mann–Whitney U rank test) (left). We also did not observe any sex differences in animals exposed to hypercapnia at baseline (p = 0.99), during challenge (p = 0.74), or following the challenge (p = 0.99; n = 4 animals per sex; Mann–Whitney U rank test) (right). (B) We observed no sex differences in sniffing frequency in animals exposed to hypoxia at baseline, (p = 0.69), during challenge (p = 0.99), or following the challenge (p = 0.89; n = 4 animals per sex; Mann–Whitney U rank test) (left). We also did not observe any sex differences in sniffing frequency in animals exposed to hypercapnia at baseline (p = 0.99), during challenge (p = 0.34), or following the challenge (p = 0.69; n = 4 animals per sex; Mann–Whitney U rank test) (right). (C) We observed no sex differences in rate of spontaneous apneas in animals exposed to hypoxia at baseline, (p = 0.23), during challenge (p = 0.17), or following the challenge (p = 0.49; n = 4 animals per sex; Mann–Whitney U rank test) (left). We also did not observe any sex differences rate of spontaneous apneas in animals exposed to hypercapnia at baseline (p = 0.34), during challenge (p = 0.42), or following the challenge (p = 0.34; n = 4 animals per sex; Mann–Whitney U rank test) (right). Data are shown as mean ± SEM.
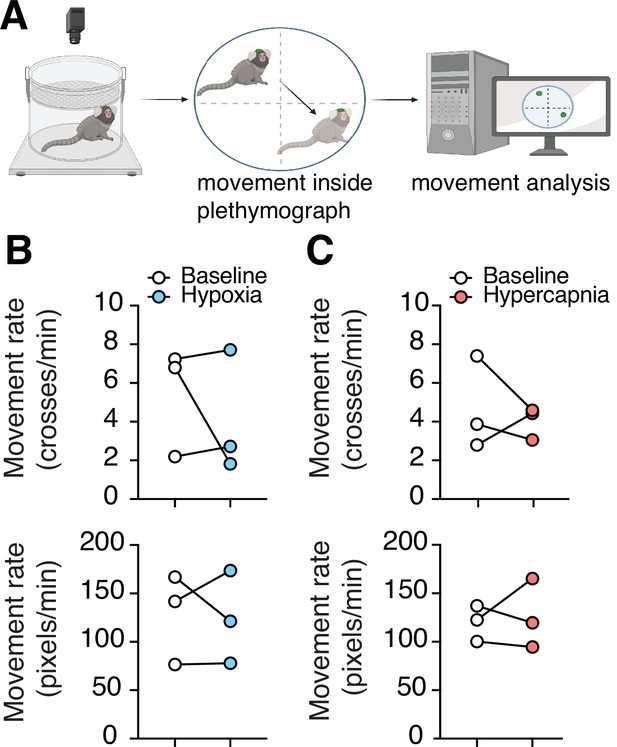
Changes in spontaneous activity during hypoxic and hypercapnic challenges.
(A) Experimental design to analyze subject movement at baseline and during challenge. (B) Hypoxia did not induce any changes in animal movement rate as measured by quadrant changes in the chamber (top) (p = 0.99, n = 3; Wilcoxon matched-pairs signed rank test), or as measured by total change in animal position per second (bottom) (p = 0.99, n = 3; Wilcoxon matched-pairs signed rank test). (C) We detected no changes in animal’s movement rate as measured by quadrant changes in the chamber (top) (p = 0.75, n = 3; Wilcoxon matched-pairs signed rank test), or by total change in position per second (bottom) (p = 0.99, n = 3; Wilcoxon matched-pairs signed rank test) during hypercapnia.
-
Figure 6—source data 1
Spontaneous activity source data.
- https://cdn.elifesciences.org/articles/71647/elife-71647-fig6-data1-v2.xlsx
Tables
Hypoxia decreased metabolic rate in common marmoset.
Pre-hypoxia | Hypoxia | Post-hypoxia | |
---|---|---|---|
Metabolic rate (%) | 100 | 51 ± 4 | 98 ± 1 |