Single-nucleus transcriptomic analysis of human dorsal root ganglion neurons
Figures
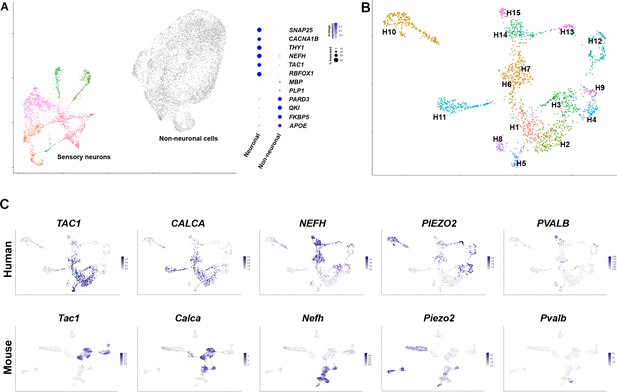
Diverse classes of human dorsal root ganglia (DRG) neurons revealed by single nuclear transcriptomics.
(A) Universal manifold (UMAP) representation of graph-based co-clustered snRNA sequences from human DRG nuclei reveal two well separated groups corresponding to sensory neurons (colored) and non-neuronal cells (gray). To the right, a dotplot highlights the expression of markers that help distinguish these groups of cells (see also Figure 1—figure supplement 2A for more information about preliminary analysis). (B) Reanalyzing 1837 neuronal nuclei clusters human DRG neurons into transcriptomically distinct groups that have been differentially colored. (C) Similarity in expression of differentially expressed genes between human and mouse neuronal types may help functional classification of neuronal types: UMAP representation of human DRG neurons showing relative expression level (blue) of diagnostic markers. For comparison UMAP representation of mouse neurons (Renthal et al., 2020) showing the relative expression patterns of the same markers. In combination, the expression patterns of these and other genes (Figure 1—figure supplements 2 and 4, Figure 1—figure supplement 5) were used to tentatively match several human and mouse transcriptomic classes (Figure 1—figure supplement 4).
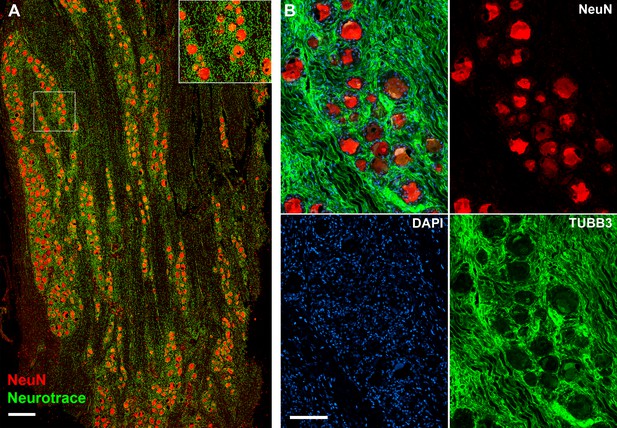
Human dorsal root ganglia (DRG) neurons express NeuN.
(A) Confocal image showing section of a DRG ganglion stained using NeuN histochemistry (red) and neurotrace (green). Note that the large diameter neurons are strongly NeuN positive whereas neurotrace also detects non-neuronal structures (scale bar = 0.5 mm). Inset shows magnified view of the boxed region. (B) Immunohistochemistry (IHC) double staining using anti NeuN (red) anti-β3 tubulin (TUBB3, green) further demonstrates NeuN staining of both small and large diameter DRG soma; the tubulin staining primarily highlights neuronal processes rich in microtubules. Also shown is 4′,6-diamidino-2-phenylindole (DAPI) staining emphasizing the need to enrich for neuronal nuclei prior to sequencing since the number of non-neuronal nuclei greatly exceeds those of neurons (scale bar = 100 µm).
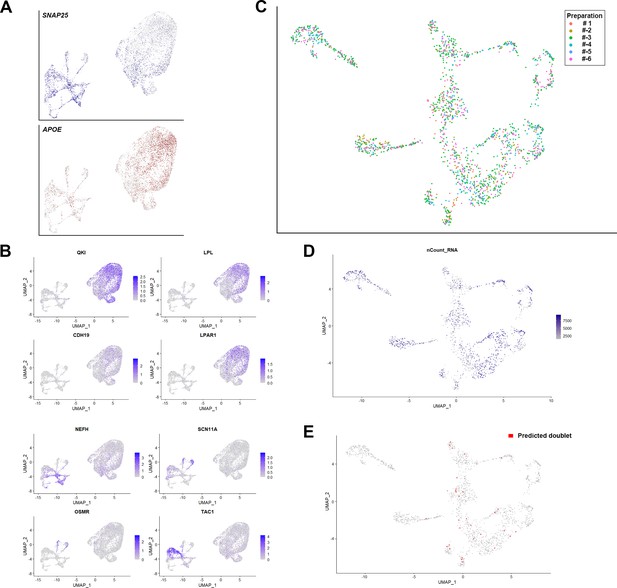
Support for the clustering of human dorsal root ganglia (DRG) neurons.
(A) Universal manifold (UMAP) representation showing relative expression levels of the neuronal marker SNAP25 (blue) and the non-neuronal gene APOE (red) in the initial clustering of sn-RNA sequencing data. It should be noted that the majority of the non-neuronal cells came from a single nuclear isolation where it is likely that neuronal nuclear purification was not effective. Sequence analysis indicated that most of the non-neuronal cells were satellite microglia although other cell types were also seen. (B) UMAP representation showing other markers that help distinguish non-neuronal nuclei from DRG neurons. (C) UMAP representation of the clustering of human DRG neurons highlighting the contributions of the six different preparations to the dataset. Note that clusters were populated with data from multiple different preparations. (D) UMAP representation of the number of genes identified per DRG neuron. (E) UMAP representation of 30 doublets predicted using DoubletFinder (see Methods).
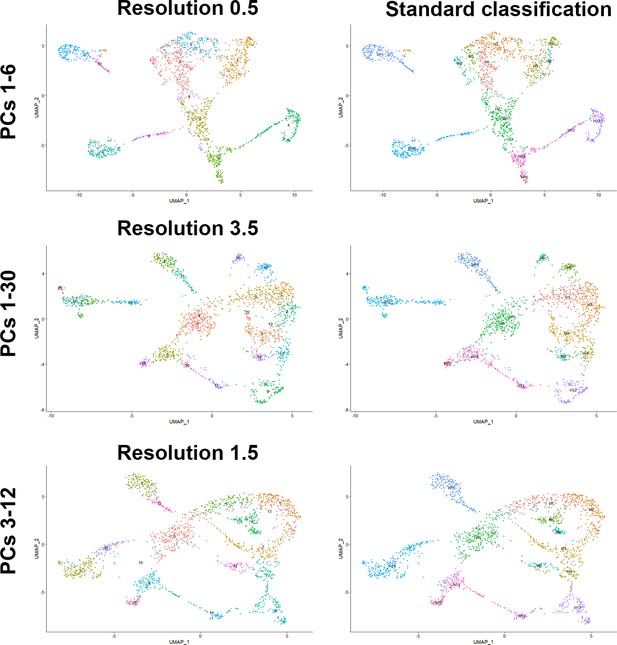
Robust clustering of human dorsal root ganglia (DRG) neurons.
Universal manifold (UMAP) representations showing analysis of human DRG neurons using different parameters in Seurat. The choice of principal components (PCs) used for UMAP display and clustering is indicated to the left and for the left column the resolution of the clustering is shown above the UMAP. Note that the number of clusters detected varies with the resolution chosen. The right column shows the same UMAP as the left column but now the original identity of neurons from Figure 1 has been mapped onto the clustering. Note that the 15 clusters map to relatively contiguous populations of neurons over a wide range of PC choices indicating that there are very robust transcriptomic differences between about a dozen different classes of DRG neurons in our dataset.
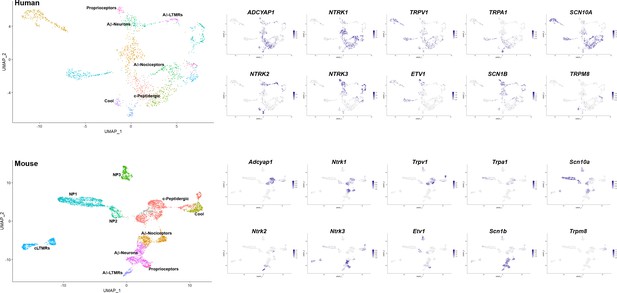
Additional markers that support similarities between dorsal root ganglia (DRG) neuronal clusters across species.
Universal manifold (UMAP) representations: upper panels, human sn-RNA sequencing; lower panels, mouse sn-RNA sequencing. To the left, identity of the different neuronal types is differentially colored. For the mouse, the identities of all clusters are indicated, for the human data, the position of names indicate the clusters with shared markers indicating a match to mouse neuronal types. The expression pattern of several such marker genes (blue) is shown to the right (see also Figure 1 and Figure 1—figure supplement 5). In the human dataset, Aδ nociceptors account for 26% of sequenced neurons; c-peptidergic nociceptors, 23% (including H5, 4%); Aβ neurons, 7%; proprioceptors, 2%; Aδ-LTMRs, 3%; and cool sensors, 3% (totaling 64% of all neurons). Other classes not identified as potential counterparts to mouse neurons based on preliminary analysis of marker expression were H4, 4%; H9, 2%; H10, 13%; H11, 10%; and H12, 7%.
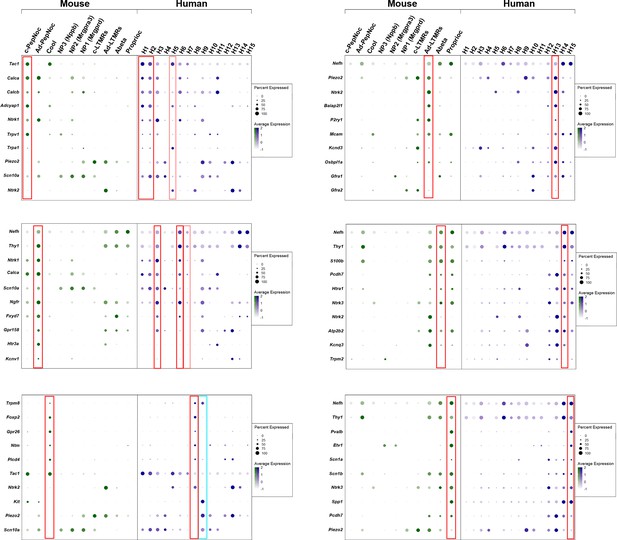
Dotplots of gene expression supporting similarity of several dorsal root ganglia (DRG) neuronal classes in mice and humans.
Dotplots displaying information about the fractional expression and relative expression level of marker genes in the different identity classes of mouse (left, gray-green scale) and human (right, gray-blue scale). Marker genes were chosen based on their expression (or lack of expression) in particular classes of mouse neurons. Red boxes highlight the relationship between a particular mouse class and human neurons; fainter red boxes indicate human classes that share some characteristics with the highlighted mouse neurons. Left panel top to bottom: c-peptidergic nociceptors (c-PepNoc); Aδ-peptidergic nociceptors (Ad-PepNoc); cool sensing cells (Cool). Right panel top to bottom: AδLTMRs (Ad-LTMRs); Aβ neurons (Abeta); proprioceptors (Proprioc). The cyan box highlights H9 a human-specific cell type that expresses TRPM8 but also nociceptor markers and the mechanosensitive channel PIEZO2 unlike the putative cool sensing cells in human (H8) and in mice.
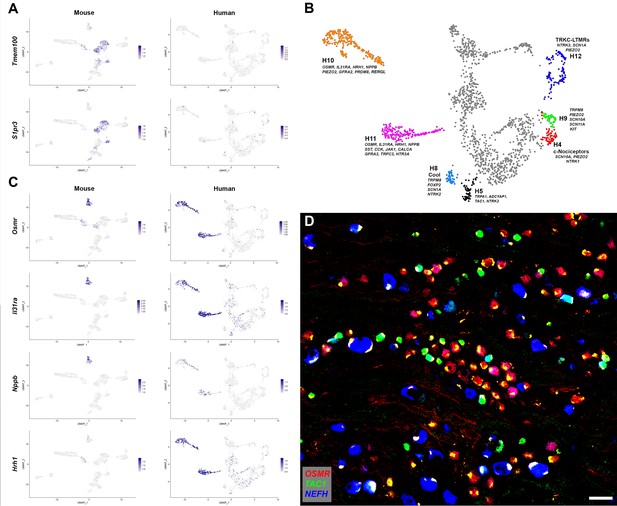
Human DRG neurons exhibit specialization that distinguishes them from mouse counterparts.
(A) Universal manifold (UMAP) representation of mouse and human dorsal root ganglia (DRG) neurons showing relative expression level (blue) of two genes that have been linked to pain sensation in mice. Note that both TMEM100 and S1PR3 are more sporadically expressed by the human somatosensory neurons. (B) Classes of DRG neurons that are selectively detected in humans are highlighted together with their expression of key genes. H9 neurons coexpress the cool and mechanosensory ion channels; for comparison cool sensitive neurons (H8) that correspond more closely with their rodent counterparts are also highlighted. (C) Expression profiles of several itch-related genes in the mouse and human DRG transcriptome. (D) Confocal image of a region from a human DRG that was labeled using multiplexed in situ hybridization (ISH) for OSMR, TAC1, and NEFH as indicated in the key. Almost all neurons were OSMR, TAC1, or NEFH positive (Figure 2—figure supplement 1). However, few neurons were strongly positive for more than one of these markers (see Figure 2—figure supplement 2 for individual channels). Note that autofluorescence in all channels from lipofuscin associated with many human neurons should not be confused with real signal (see Figure 2—figure supplement 2 for more detail). Also note that NEFH is typically expressed in larger diameter neurons than the other two markers. Scale bar = 100 µm.
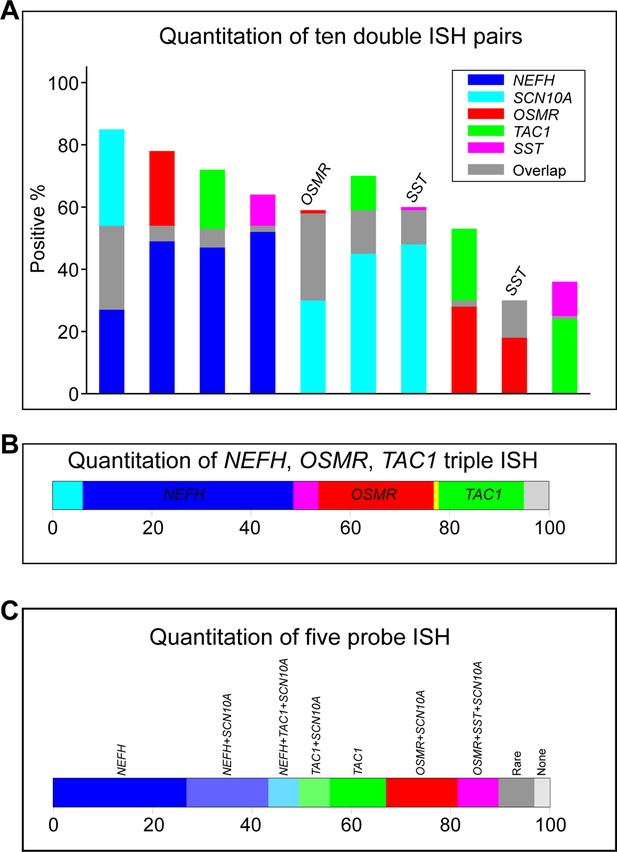
Quantification of in situ hybridization (ISH) data.
The extensive autofluorescence in ISH of human dorsal root ganglia (DRG) section prevents automated signal detection. Thus, quantitation of ISH data involves manually scoring positive and negative cells. Therefore, we only quantitated the highest intensity signals with the most clear-cut expression patterns. In total we scored 1101 total cells in sections from a 35-year-old female and an 18-year-old male donor. The proportion of positively scored cells for five probes where signal strength, consistency in expression level and frequency of positives allow confidence in the accuracy of scoring and the representative nature of the quantitation were: NEFH, 54%; SCN10A, 58%; OSMR, 29%; TAC1, 25%; and SST, 12%. (A) Bar graphs displaying the 10 pairs of combinations of these five probes (overlap, gray) represents cells expressing both markers in that bar; where overlap is extensive the identity of the second gene has been added above the bar. (B) Bar graph displaying expression of the three probes shown in Figure 2D; NEFH, blue; OSMR, red; TAC1, green; overlap between NEFH and TAC1, cyan; NEFH and OSMR, magenta; OSMR and TAC1, yellow; only about 5% of cells were not positive for any of these three probes. (C) Bar graph displaying expression of the five probes; all populations that make up more than 5% of cells are colored and labeled. Rare combinations include cells positive for NEFH, SCN10A, and OSMR, 3%; NEFH, SCN10A, SST, and OSMR, 2%; SCN10A only, 2%; SST and OSMR, 2%; and SCN10A, TAC1, SST, and OSMR, 1%. None represents 3% of cells that were not positive for expression of these genes but were detected by other probes TRPM8, NTRK2, PIEZO2, and TRPV1 used for hybridization of these sections.
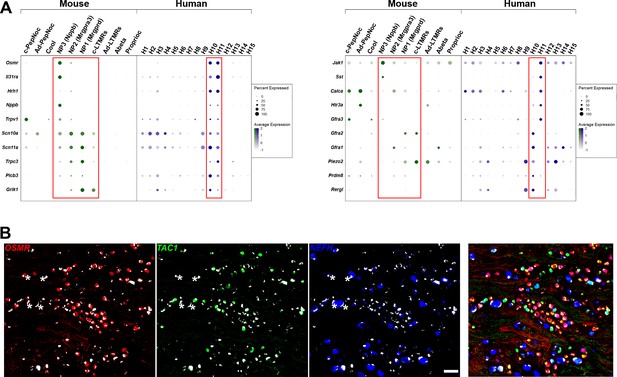
H10 and H11 are classes of human dorsal root ganglia (DRG) neurons that express a range of itch-related genes.
(A) Dotplots displaying information about the fractional expression and relative expression level of marker genes in the different identity classes of mouse (left, gray-green scale) and human (right, gray-blue scale). Left panel, a selection of genes with relatively similar expression in H10 and H11 cells includes functionally relevant genes that tune mouse NP3 cells to respond to itch. Right panel shows genes that distinguish H10 and H11; some of these are also markers for mouse NP3 cells, others are more highly expressed in the other classes of mouse small diameter nonpeptidergic neurons. Red boxes highlight H10 and H11 and the four classes of mouse small diameter nonpeptidergic neurons. (B) Left three panels show individual channels for the in situ hybridization (ISH) shown in Figure 2D highlight NEFH as a marker for large diameter neurons and further emphasize the relatively limited overlap between these three probes. Strong autofluorescence signals that are present in all channels have been masked in white; four examples of autofluorescence (where there is also signal for one probe in that cell) are highlighted by stars. Right panel: the merged channels with brightness and contrast adjusted to help show that areas devoid of neurons are occupied with nerve fibers.
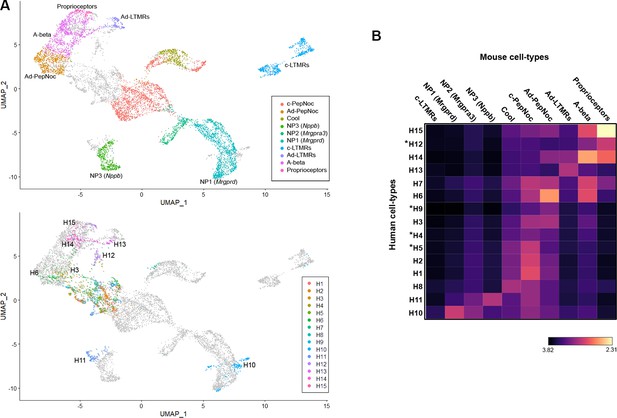
Co-clustering of human and mouse neurons support tentative assignments based on select genes.
(A) Universal manifold (UMAP) representation of the co-clustering of mouse and human neurons. Upper panel shows the mouse neurons colored by their identity when analyzed alone (Figure 1—figure supplement 1); lower panel shows human neurons colored by their identity when analyzed alone (Figure 1). Note that large diameter human neurons match their expected mouse counterparts reasonably well and that the two classes of neurons expressing itch-related transcripts H10 and H11 best match NP1 and NP3 neurons, respectively. (B) Heatmap showing the natural logarithm (see scale bar) of Kullback–Leibler divergences for the various human neuron classes when compared to each class of mouse cells as a reference distribution; potentially human-specific classes based on functional markers are marked by *; see also Figure 3—figure supplement 1.
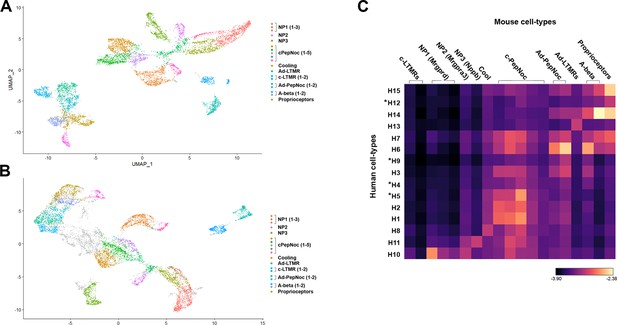
More detailed analysis of relationships between human and mouse cell types.
(A) Universal manifold (UMAP) representation of clustered mouse neurons using parameters that identify 19 different groups of cells and (B) their distribution in the co-clustering with human neurons. (C) Heatmap showing the natural logarithm (see scale bar) of Kullback–Leibler divergences for the various human neuron classes when compared to each class of mouse cells as a reference distribution; potentially human-specific classes based on functional markers are marked by *. Note that despite the increased granularity of clustering results were highly consistent with those in Figure 3.
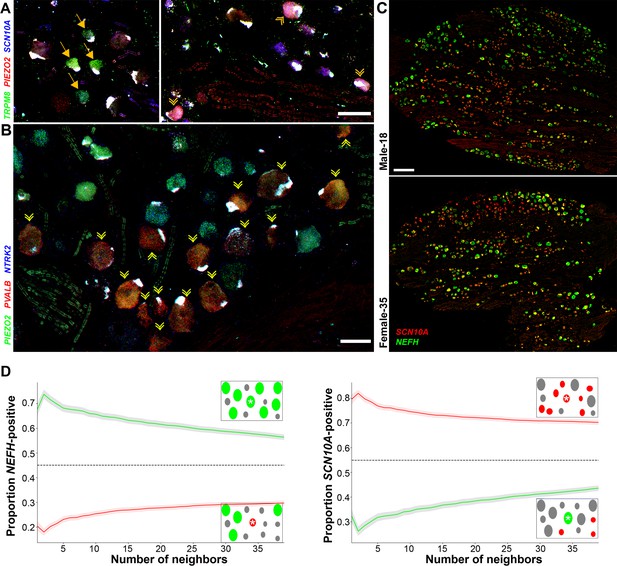
Transcriptomically related classes of human dorsal root ganglia (DRG) neurons are spatially clustered in the ganglion.
Confocal images of sections through a human DRG probed for expression of key markers using multiplexed in situ hybridization (ISH); see Figure 4—figure supplement 1 for the individual panels and additional probes. (A) Left panel shows a group of four H8-neurons (yellow arrows) that express TRPM8 (green) but not PIEZO2 (red) or SCN10A (blue). By contrast, right panel shows a different region of the ganglion where three H9-neurons coexpress these three transcripts (double arrowheads). (B) Other regions of the ganglia were dominated by larger diameter neurons. Putative proprioceptors, highlighted by double arrowheads, expressing PIEZO2 (green) and PVALB (red), but not NTRK2 (blue) were typically highly clustered in the ganglion. (C) Lower magnification images of complete sections stained for NEFH (green) and SCN10A (red) highlight the extensive co-clustering of large and small diameter neurons in different individuals. Scale bars = 100 µm in (A and B); 500 µm in (C). (D) The nearest n neighbors of NEFH and SCN10A single positive neurons in (C) were identified (for n = 1–40): green lines represent proportion (mean, solid line ± standard error of mean [SEM], shaded) of cells surrounding NEFH-positive neurons; red lines, proportion (mean, solid line ± SEM, shaded) of cells surrounding SCN10A-positive neurons. Left panel: proportion of surrounding cells that were NEFH positive. Right panel: proportion of surrounding cells that were SCN10A positive. Dashed black lines are the proportions expected for randomly distributed cells. Insets schematically show a central cell (highlighted by a star) and the surrounding neurons. Neighboring NEFH-positive cells are colored green and SCN10A-positive cells are colored red when these are being scored in the associated graph; gray cells are positive for the other marker. Clustering was statistically significant across the complete range (1–40 neighbors), p ≤ 6.96 × 10−42 (one-tailed Mann–Whitney U-test); n = 803 single positive cells confirming both short and long-range grouping of similar classes of human DRG neurons.
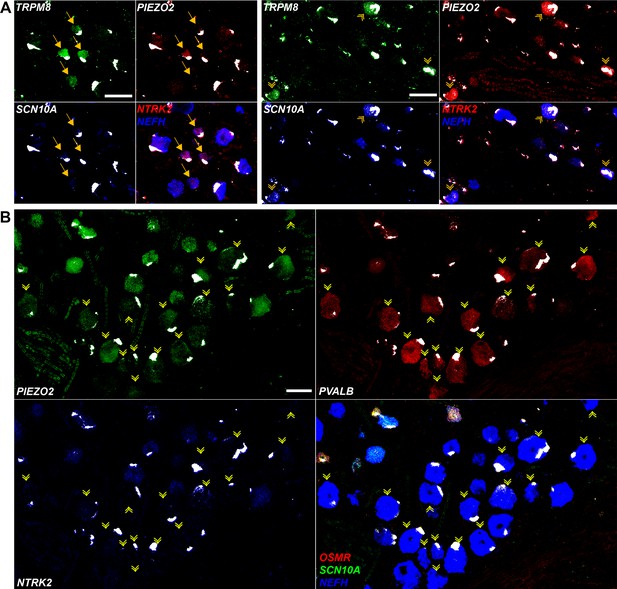
Expression profiles of clusters of H8 (cool), H9 (human-specific), and H15 (proprioceptive) neurons.
Individual channels for the in situ hybridization (ISH) images in Figure 4 are shown to highlight the expression patterns described in the text. Strong autofluorescence signals that are present in all channels have been masked in white. Additional combinations of gene expression data in these regions of the ganglion are shown to identify neurons and reveal extra transcriptomic information. (A) Spatial clusters containing H8 putative cool sensing neurons (left) and H9 cool/mechanosensory nociceptors (right) are shown and identified as in Figure 4A. H8 neurons are generally NTRK2 positive but are only weakly positive for NEFH. By contrast, H9 cells are NEFH positive but do not express significant NTRK2, in keeping with transcriptomic data. Note that clusters of these neurons segregate in distinct fields of the ganglion. (B) H15 (presumptive proprioceptors) form a subcluster of NEFH-positive cells that are essentially devoid of nociceptors (marked by SCN10A) including nonpeptidergic neurons expressing OSMR. Many of the PVALB-negative large diameter neurons in this region of the ganglion were NTRK2 positive; by contrast this gene was not detectable in H15 cells in keeping with the transcriptomic data. Scale bars = 100 µm; arrows and arrowheads are as in Figure 4.
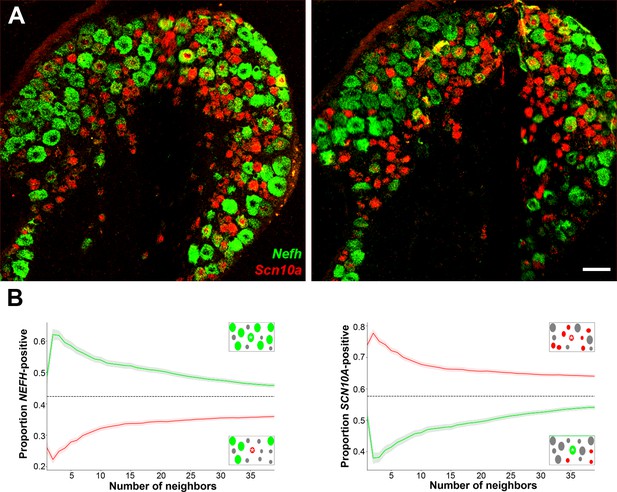
Expression profiles of clusters of H8 (cool), H9 (human-specific), and H15 (proprioceptive) neurons.
(A) Confocal images of mouse lumbar dorsal root ganglia (DRG) in situ hybridization (ISH) showing Nefh (green) and Scn10a (red); scale bars = 100 µm. (B) The nearest n neighbors of Nefh and Scn10a single positive neurons were identified (for n = 1–40): green lines represent proportion (mean, solid line ± standard error of mean [SEM], shaded) of cells surrounding Nefh-positive neurons; red lines, proportion (mean, solid line ± SEM, shaded) of cells surrounding Scn10a-positive neurons. Left panel: proportion of surrounding cells that were Nefh positive. Right panel: proportion of surrounding cells that were Scn10a positive. Dashed black lines are the proportions expected for randomly distributed cells. Insets schematically show a central cell (highlighted by a star) and the surrounding neurons. Neighboring Nefh-positive cells are colored green and Scn10a-positive cells are colored red when these are being scored in the associated graph; gray cells are positive for the other marker. Clustering was statistically significant across the complete range (1–40 neighbors), p ≤ 3.98 × 10−15 (one-tailed Mann–Whitney U-test); n = 1117 single positive cells confirming both short- and long-range grouping of similar classes of mouse DRG neurons.
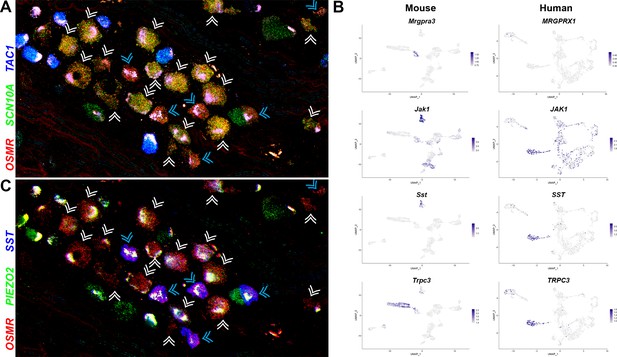
Two related classes of human nonpeptidergic small diameter neurons that may mediate itch.
(A) Confocal image of a section through a human dorsal root ganglia (DRG) probed for nociception-related genes using multiplexed in situ hybridization (ISH). In this view, many neurons expressing the itch-related transcript, OSMR (red), are grouped together (arrowheads); cyan arrowheads point to cells that express relatively higher levels of OSMR than SCN10A (green). Peptidergic nociceptors marked by expression of TAC1 (blue) and additional SCN10A-positive cells are also present in this region of the ganglion. (B) Universal manifold (UMAP) representation of mouse and human DRG neurons showing relative expression level (blue) of genes that distinguish H10 and H11 and mark-specific sets of mouse NP1–3 neurons. MRGPRX1 is the human chloroquine receptor and the functional equivalent of Mrgpra3, which in mice marks NP2 cells. Note that coexpression patterns of SST and JAK1 in H11 neurons resembles their expression in mouse NP3 pruriceptors but the ion channel TRPC3 which also marks these cells is primarily expressed in mouse NP1 neurons; see Figure 5—figure supplement 1 for additional breakdown of similarities and differences between nonpeptidergic neurons in mice and humans. (C) Confocal image of the group of candidate pruriceptors shown in (A) probed for expression of genes that distinguish H11 (SST, blue) from H10 cells (PIEZO2, green); note that neurons highlighted with cyan arrowheads have gene expression expected for H11 cells, whereas some H10 cells express lower levels of SST and also exhibit variation in the level of PIEZO2 expression. Scale bars = 100 µm; see Figure 5—figure supplement 1B for individual channels and for expression of additional markers.
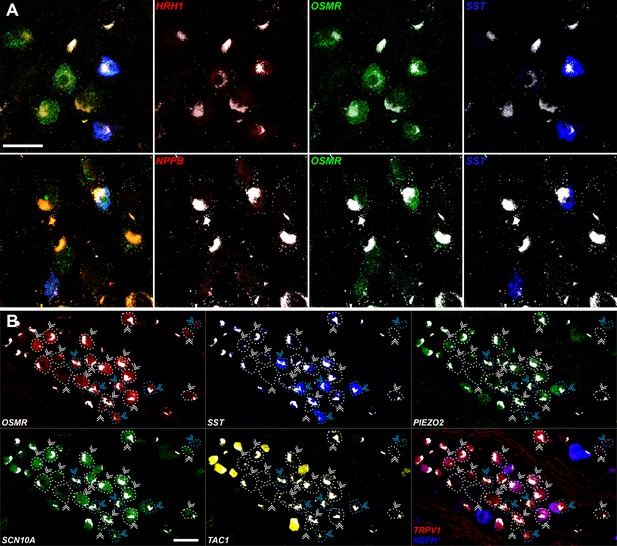
Expression profiles of human H10 and H11 dorsal root ganglia (DRG) neurons.
(A) Confocal in situ hybridization (ISH) images illustrating weak expression of itch-related transcripts HRH1 (upper panels, red) and NPPB (lower panels, red) in H10 and H11 neurons. Left image shows multiplex ISH of a region of a DRG section where H10 and H11 cells can be recognized by expression of OSMR (green); H11 cells also express SST (blue). To the right, individual channels demonstrating low-level expression of HRH1 and NPPB (punctate red signal) in H10 and H11 neurons. (B) Individual channels for the ISH images in Figure 5 are shown to highlight the expression patterns described in the text. Strong autofluorescence signals that are present in all channels have been masked in white. In addition, ISH reveals that H10 and H11 neurons also express TRPV1 but as expected from the transcriptomic data at most express very low levels of NEFH. Scale bars = 100 µm; arrowheads are as in Figure 5; to further highlight the relevant cells, OSMR-positive cells are highlighted by dotted outlines that match the coloring of arrowheads.
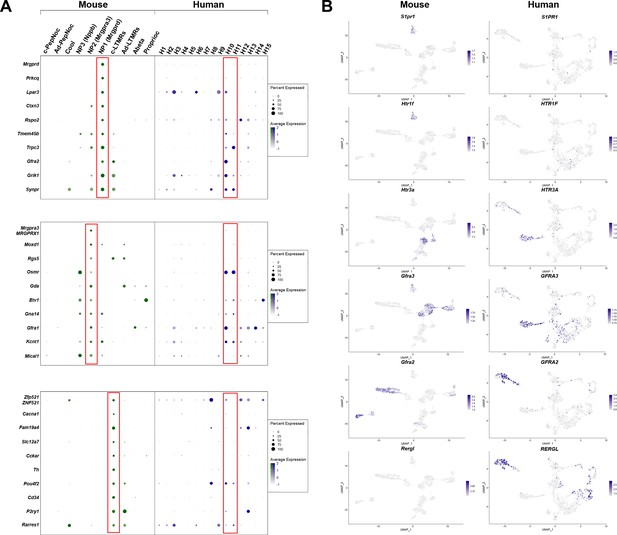
Gene expression patterns in H10 and H11 classes of human dorsal root ganglia (DRG) neurons are distinct from classes of mouse small diameter nonpeptidergic neurons.
(A) Dotplots displaying information about the fractional expression and relative expression level of marker genes in the different identity classes of mouse (left, gray-green scale) and human (right, gray-blue scale). From top to bottom, markers of NP1, NP2, and cLTMRs in mice generally show only limited expression in the presumptive human nonpeptidergic nociceptors H10 and H11. NP1-selective genes confirm greater similarity of H10 neurons to this type of mechanonociceptor. Right panels: Universal manifold (UMAP) representation of mouse and human DRG neurons showing relative expression level (blue) of several genes that highlight differences between H10 and H11 neurons and potential mouse counterparts. (B) UMAP representation of mouse and human DRG neurons showing relative expression level (blue) of several genes highlighting differences between H10 and H11 neurons and potential mouse counterparts.
Tables
Reagent type (species) or resource | Designation | Source or reference | Identifiers | Additional information |
---|---|---|---|---|
Strain, strain background (Mus musculus) | C57BL/6NCrl | Charles River | Strain code: 027 | Male and female |
Antibody | anti-NeuN (rabbit polyclonal) | Millipore | Cat#ABN78 | (1:4000 for nuclei isolation)(1:1000 for IHC) |
Antibody | anti-Tubb3 (mouse monoclonal) | Proteintech | Cat#66375-1-Ig | (1:500) |
Antibody | anti-rabbit Cy3-conjugated(donkey polyclonal) | JacksonImmuno Research | Cat#711-166-152 | (1:1000) |
Antibody | anti-mouse FITC-conjugated(donkey polyclonal) | JacksonImmuno Research | cat#715-096-150 | (1:1000) |
Sequence-based reagent | Chromium Next GEM Single Cell 3′ GEM, Library & Gel Bead Kit v3.1 | ×10 Genomics | Cat#1000128 | |
Sequence-based reagent | Chromium Next GEM Chip G Single Cell Kit | ×10 Genomics | Cat#1000127 | |
Commercial assay or kit | RNAscope HiPlex8 Detection reagents | Advanced Cell Diagnostics | Cat#324110 | |
Commercial assay or kit | RNAscope HiPlex12 Ancillary reagents | Advanced Cell Diagnostics | Cat#324120 | |
Commercial assay or kit | Human RNAscope probes (HiPlex 12) | Advanced Cell Diagnostics | NEFH (cat# 448141); TRPM8 (cat# 543121); PIEZO2 (cat# 449951); SCN10A (cat# 406291); NTRK2 (cat# 402621); TAC1 (cat# 310711); OSMR (cat# 537121); SST (cat# 310591); TRPV1 (cat# 415381); PVALB (cat# 422181) | |
Commercial assay or kit | RNAscope Fluorescent Multiplex assay | Advanced Cell Diagnostics | Cat #320851 | |
Commercial assay or kit | Mouse RNAscope probes(MultiPlex) | Advanced Cell Diagnostics | Scn10a (cat#426011); Nefh (cat#443671) | |
Commercial assay or kit | Human RNAscope probes(MultiPlex) | Advanced Cell Diagnostics | OSMR (cat#537121); SST (cat# 310591); HRH1 (cat#416501); NPPB (cat#448511); | |
Commercial assay or kit | NeuroTrace Green | Fisher Scientific | Cat#N21480 | (1:100) |
Software, algorithm | CellRanger | ×10 Genomics | Version 2.1.1 | GRCh38.v25. premRNA |
Software, algorithm | Seurat | Satija lab | Versions 3-4.04 | https://satijalab.org/seurat/ |
Software, algorithm | Python | python.org | Version 3.7. | |
Software, algorithm | scipy.stats | scipy.org | Version 1.5.2 | |
Software, algorithm | scikit-learn | scikit-learn.org | Version 0.23.2 | |
Software, algorithm | RStudio | https://www.rstudio.com/ | Version 1.4.1106 | |
Software, algorithm | R | https://www.r-project.org/ | R version 4.1.1 | |
Software, algorithm | DoubletFinder | https://github.com/chris-mcginnis-ucsf/DoubletFinder | McGinnis lab (McGinnis, 2021) | |
Software, algorithm | ImageJ | http://imagej.nih.gov/ij | ImageJ 1.53 c | |
Software, algorithm | Adobe Photoshop | https://www.adobe.com/ | 25.5.1 release | |
Software, algorithm | kl_divergence | https://gist.github.com/lars-von-buchholtz/636f542ce8d93d5a14ae52a6c538ced5636f542ce8d93d5a14ae52a6c538ced5 | ||
Other | RNA-later | Thermo Fisher | Cat# AM7021 | |
Other | Spectrum Bessman tissue pulverizer | Fisher Scientific | Cat# 08-418-3 | |
Other | Dounce homogenizer | Fisher Scientific | Cat# 357538 | |
Other | 40 μm cell strainer | Thermo Fisher | Cat# 08-771-1 | |
Other | Low bind microfuge tubes | Sorenson BioScience | Cat# 11,700 | |
Other | SUPERaseIn RNase inhibitor | Thermo Fisher | Cat# AM2696 | 0.2 U/μl |
Other | anti-rabbit IgG microbeads | Miltenyi biotec | Cat# 130-048-602 | |
Other | LS column | Miltenyi biotec | Cat# 130-042-401 | |
Other | Mouse DRG dataset | Woolf lab | GSE154659 | Renthal et al., 2020, Neuron |
Sequencing data for the five individual DRG nuclear preparations.
Preparation# | DRG1-F36 | DRG2-M36 | DRG3a-F34 | DRG3b-F34 | DRG4-F35 | DRG5-F55 |
---|---|---|---|---|---|---|
Number of reads | 273,123,313 | 121,333,026 | 105,113,253 | 101,427,234 | 90,306,793 | 94,069,716 |
Valid barcodes | 97.50% | 97.60% | 98.20% | 96.10% | 93.60% | 97.90% |
Sequencing saturation | 93.20% | 93.10% | 39.70% | 82.10% | 38.70% | 55.90% |
Q30 bases in barcode | 96.30% | 97.40% | 97.80% | 97.50% | 97.70% | 96.80% |
Q30 bases in RNA read | 88.30% | 85.90% | 87.40% | 91.50% | 93.40% | 92.20% |
Q30 bases in sample index | 96.00% | 96.80% | 97.40% | 96.60% | 96.60% | 94.80% |
Q30 bases in UMI | 95.50% | 97.00% | 97.60% | 97.00% | 97.20% | 96.10% |
Reads mapped confidently to genome | 86.50% | 81.20% | 71.20% | 16.90% | 10.80% | 18.10% |
Reads mapped confidently to intergenic regions | 4.10% | 4.20% | 4.00% | 1.30% | 0.90% | 1.20% |
Estimated number of cells | 584 | 273 | 6,180 | 223 | 872 | 999 |
Mean reads per cell | 467,676 | 444,443 | 17,008 | 454,830 | 135,189 | 94,163 |
Median genes per cell | 1917 | 709 | 786 | 481 | 872 | 812 |
Total genes detected | 24,646 | 17,559 | 28,759 | 17,338 | 24,798 | 25,027 |
Median UMI counts per cell | 2929 | 862 | 965 | 663 | 1,293 | 988 |
DRG cells in final object | 212 | 152 | 770 | 80 | 281 | 342 |