Multisensory-motor integration in olfactory navigation of silkmoth, Bombyx mori, using virtual reality system
Figures
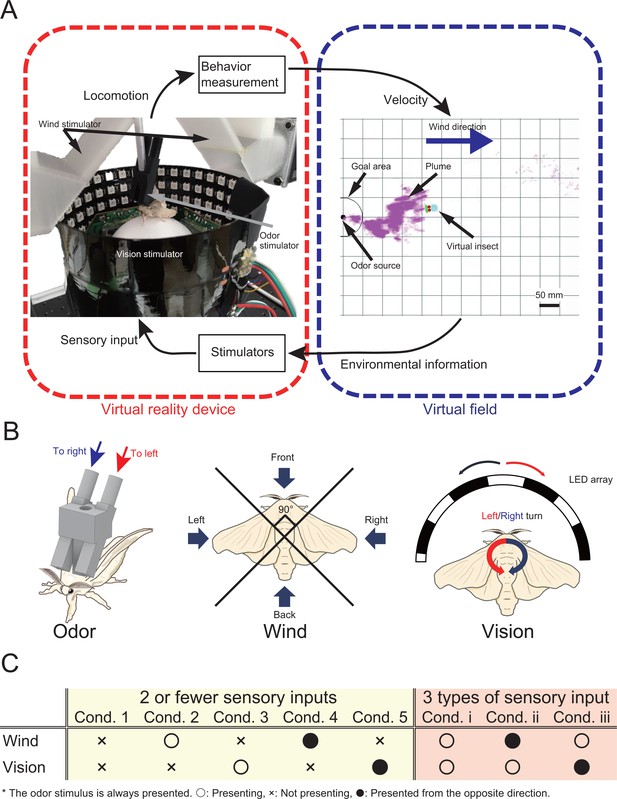
The virtual reality (VR) system for olfactory navigation of the insect and a list of experimental conditions.
(A) The VR system is equipped with a stimulator of odor, vision, and wind, and is connected to a virtual odor field. The insect on the VR device performs olfactory navigation in a virtual space. (B) Definition of the way of presentation of each sensory stimulus. (C) The odor is presented under all conditions. ‘Ο’, ‘X’, and ‘’ indicate presented, not presented, and presented from a direction opposite to the actual direction, respectively.
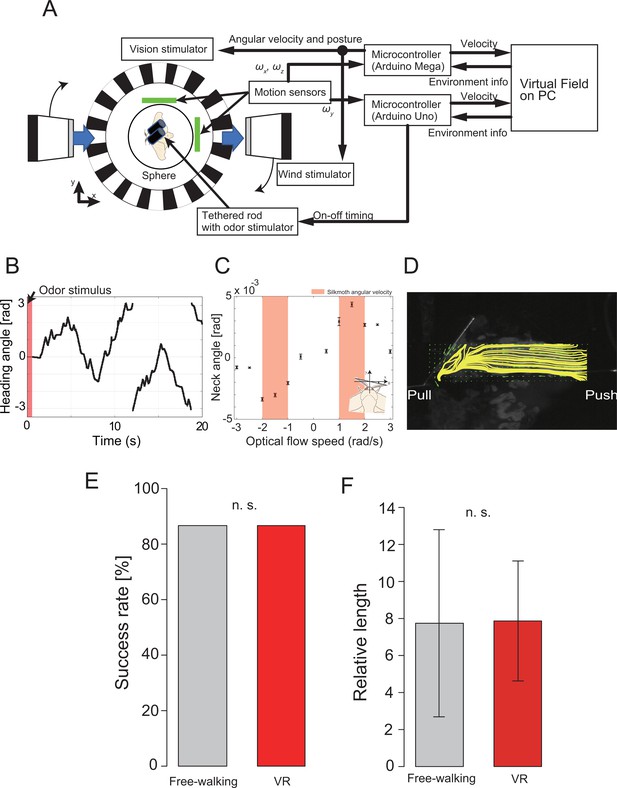
System configuration diagram and evaluation of virtual reality (VR) system.
Data comparison between the free walking experiment and VR experiment. (A) VR system configuration diagram. Two microcomputers were used to control the stimulator and measure the amount of rotation of the sphere. (B—D) Evaluation results of each stimulator. (B) shows the change in the heading angle of the silkmoth. It was confirmed that when the odor was presented from the upper part of the antennae, female search behavior was elicited while changing the heading angle significantly. (C) investigated whether the visual motion reflex was elicited by the visual stimulator by observing the change in the angle of the neck. Because the neck tilt was the largest in the same speed band as the angular velocity of the silk moth, it was confirmed that the visual stimulus was presented correctly. (D) is a snapshot of the push-pull rectifier, which is a wind stimulator, visualized by PIV. Since the yellow line represents the streamline and does not generate vortices, it was confirmed that rectification could be generated by push-pull. (E) Comparison of search success rates between the free walking and VR experiments (Fisher’s exact test, p < 0.05). The free walking experiment was the result of two repeated experiments using 15 silkmoths ( = 30). The environment of the free walking experiment was set to be the same as the virtual environment of the VR system. (F) Comparison of relative length. The relative length is an evaluation value that standardizes the distance actually traveled to the shortest distance to the odor source. This makes it possible to evaluate how much action is taken in response to a sensory input. Because there was no difference between free walking and VR (Welch’s t-test, p < 0.05), it is highly possible that the search behavior expressed by a silkmoth in the VR experiment was the same as the behavior in the free walking experiment .
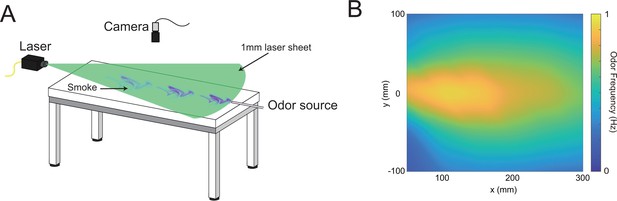
Plume capturing method and odor frequency property of plume.
(A) The plume model used a video of the behavior of odor particles on a two-dimensional plane using PIV technology. (B) Distribution of odor frequency produced by the actual plume photographed in A. We measured the average odor frequency of 10 repetitions of an experiment in which an odor was emitted from the odor source at a frequency of 1 Hz for 5 min. The odor frequency was 1 Hz closer to the odor source, indicating that there was a correlation between the odor frequency and the distance from the odor source.
A video of a silkmoth behavior experiment using a virtual reality (VR) system.
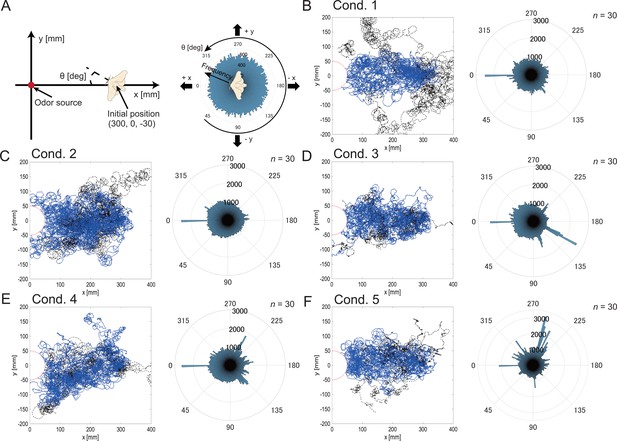
Definition of odor-source search experimental fields and initial heading angle (A), and the result of trajectories and heading angle histograms under each experimental condition (B—F).
The blue and black lines are the trajectories of successful and unsuccessful searches, respectively. Moreover, the amplitude of the heading angle histogram displayed in polar coordinates indicates the frequency. The higher the frequency, the more he silkmoth moved in that direction. Note that the 0°direction is the windward direction and does not necessarily move toward the odor source.
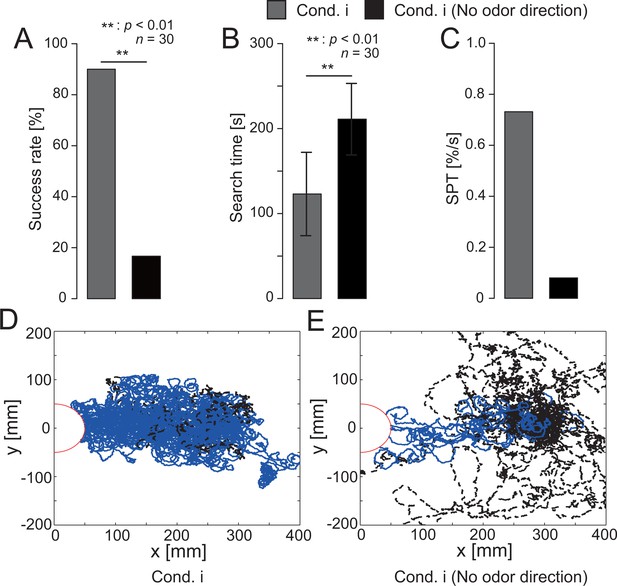
Odor-source search performance of a silkmoth when the direction of odor stimulation is lost.
Regardless of which insect antennae in the virtual environment detected the odor, we provided the odor stimulus to both antennae of the actual silkmoth. This meant that the silkmoth lost bilateral information of odor. Vision and wind were presented in the same way as in cond. i. (A, B, and C) represent the results of the search success rate, search time, and search performance, respectively. (D and E) represent the trajectories with and without bilateral information, respectively. The blue and black lines of the trajectories represent success and failure, respectively. When the bilateral information of the odor is lost, the search success rate drops significantly because the momentary direction information is lost. This means that the left and right odor information is important in the odor-source search, and that our odor stimulator correctly provides the odor information into the left and right antennae.
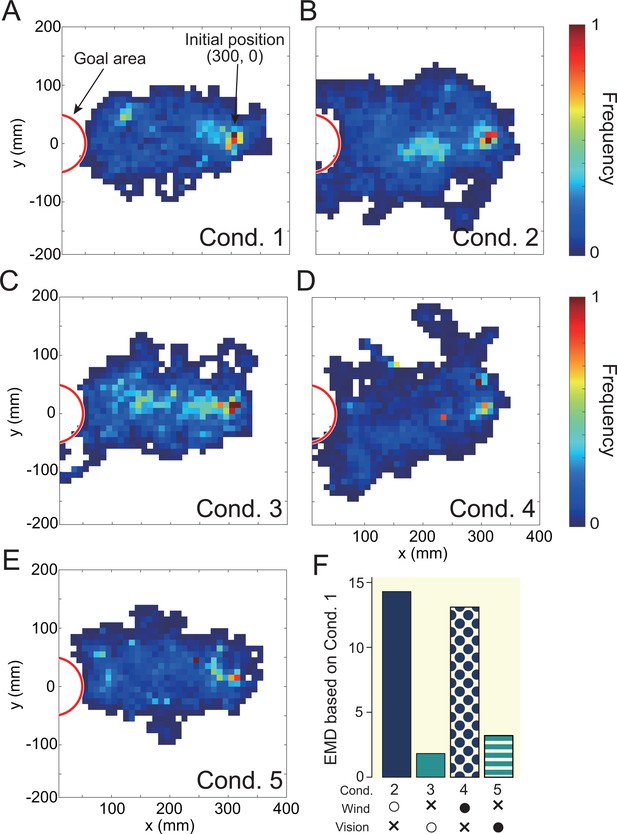
The result of visually expressing the trajectory under each experimental condition with a migration probability map (A—E).
The white area in the figure indicates that the moth did not move into that space. A quantitative evaluation using earth mover’s distance (EMD) is illustrated in F, which is the result of calculating the similarity (EMD) based on the trajectory of cond. 1 (odor presentation only). The lower the value, the higher the similarity, and the higher the value, the lower the similarity of the trajectories.
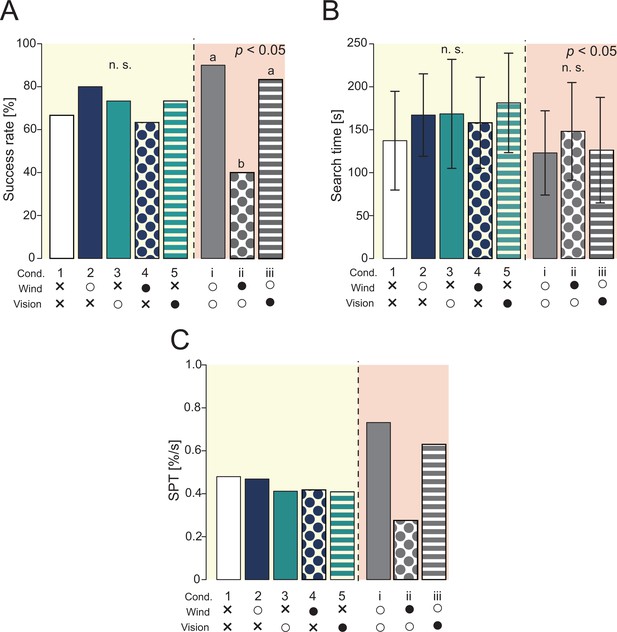
Results of navigation experiments using virtual reality (VR).
The yellow background is the result of providing two or fewer sensory stimuli, and the red background is the result of providing three sensory stimuli. (A) The success rate of the navigation (Fisher’s exact test, p < 0.05). (B) The search time at the time of success (Steel-Dwass test, p < 0.05). (C) Search performance. The success rate per unit time was calculated based on the results of A and B.
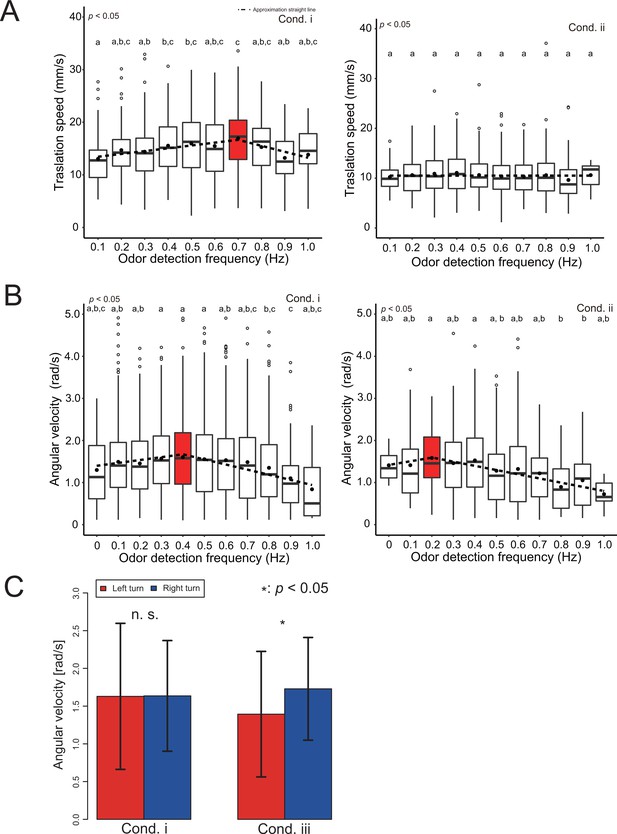
Analysis of the effects of wind and vision direction on behavior.
(A) Changes in the translational velocity and angular velocity when the wind direction is presented correctly (cond.i). Both translation and angular velocity peak, and the behavior is modulated depending on the odor detection frequency. (B) Changes in translational velocity and angular velocity when the wind is presented from the direction opposite to the actual direction (cond. ii). The translational velocity is constant regardless of the odor detection frequency, and the peak position of the angular velocity is 0.5 times that of cond. i. (C) Comparison of angular velocities when the visual information is presented correctly (cond. i) and when it is presented in the opposite direction (cond. iii). Vision was used to equalize the speed of the left-right rotation.
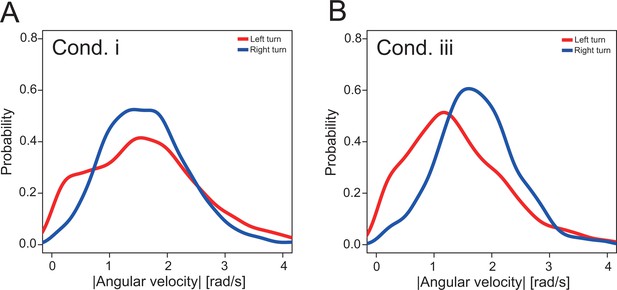
Histogram of the angular velocity of cond. i and cond. iii.
The vertical axis is probability and the horizontal axis is the angular velocity (absolute value). The red and blue lines represent the probability density distribution for left or right rotation, respectively. The peak position of the probability density distribution shifts depending on the presentation conditions of the visual stimulus.
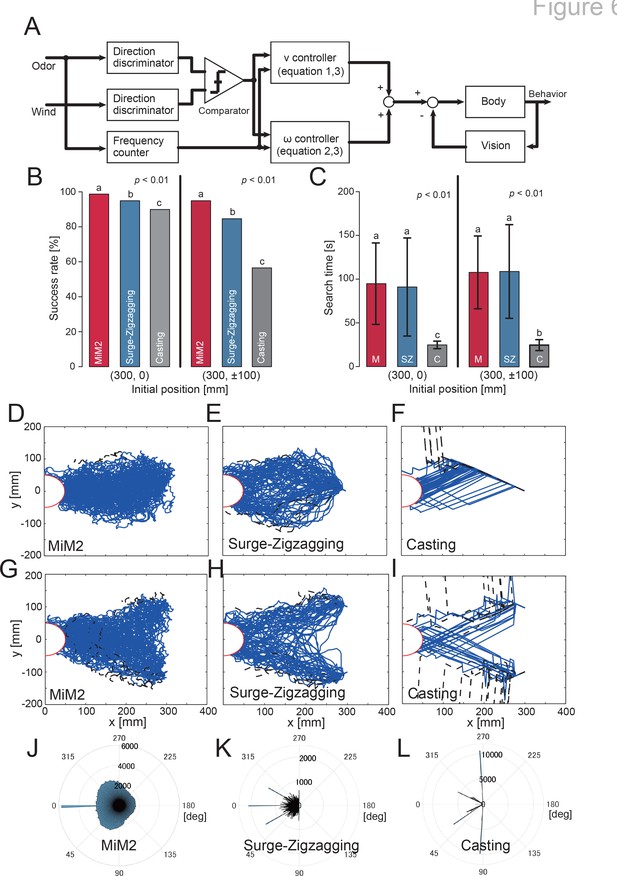
Block diagram of the proposed model and the simulation results.
(A) Control model that modulates speed according to the degree of coincidence between odor detection direction and wind direction. (B) The success rate of navigation. (C) The search time at the time of success. (D—I) The result of trajectories under each experimental condition. The blue and black lines are the trajectories of successful and unsuccessful searches, respectively. (J—L) The result of heading angle histograms under each experimental condition. The amplitude of the heading angle histogram displayed in polar coordinates indicates the frequency: the higher the frequency, the more the agent moves in that direction. Note that the direction is the windward direction and does not necessarily move toward the odor source.
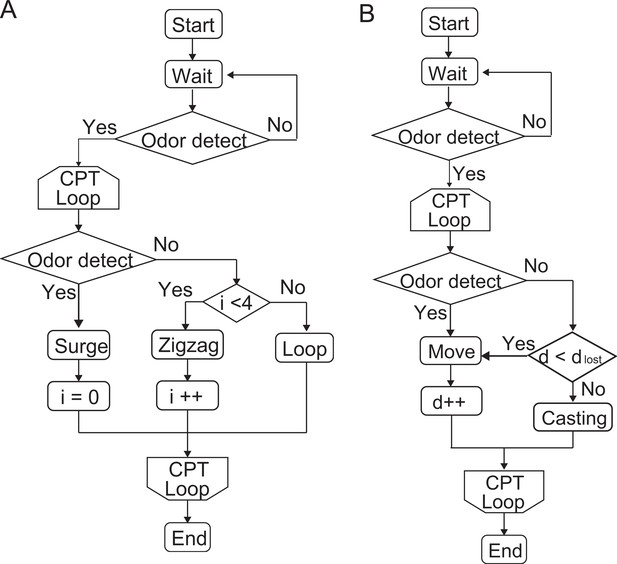
Flowchart of other algorithms.
Flowchart of the algorithm used in the simulation experiment. (A) Flowchart of the surge-zigzagging algorithm. The surge state and the zigzag/loop state are switched by the odor stimulus. (B) Flowchart of the casting algorithm. By detecting the odor, it moves upwind, and when it loses its odor, it casts in the crosswind direction.
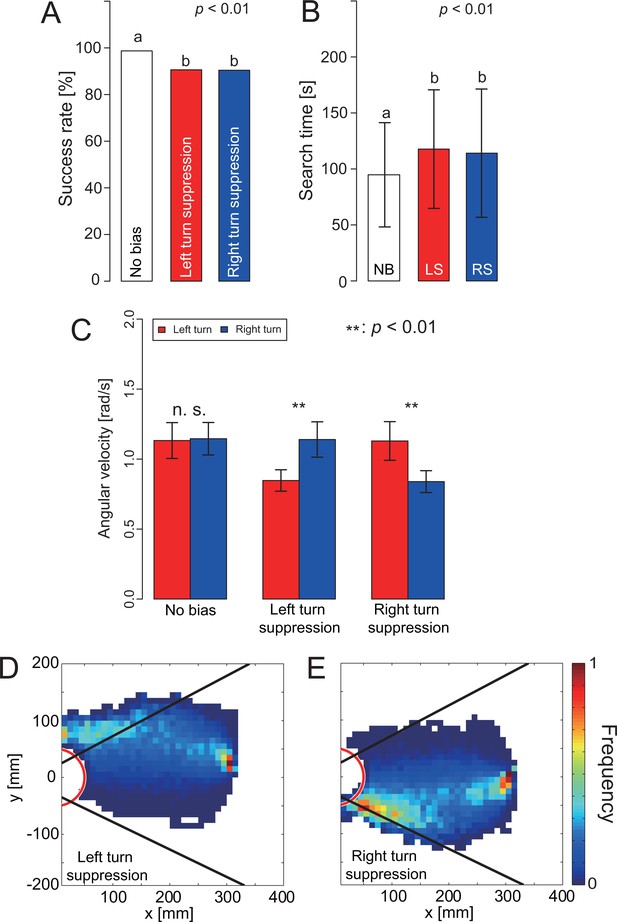
Simulation results when the left and right angular velocities are out of balance in rotational motion.
If the angular velocities in the left and right rotational motions are different, the search trajectory is biased, so that the path becomes longer and the search time is significantly longer. The search success rate also drops, similar to the results of the biological experiments. Accordingly, it is suggested that the balance of left and right rotational motion contributes to an efficient search.
Experimental video of the simulation.
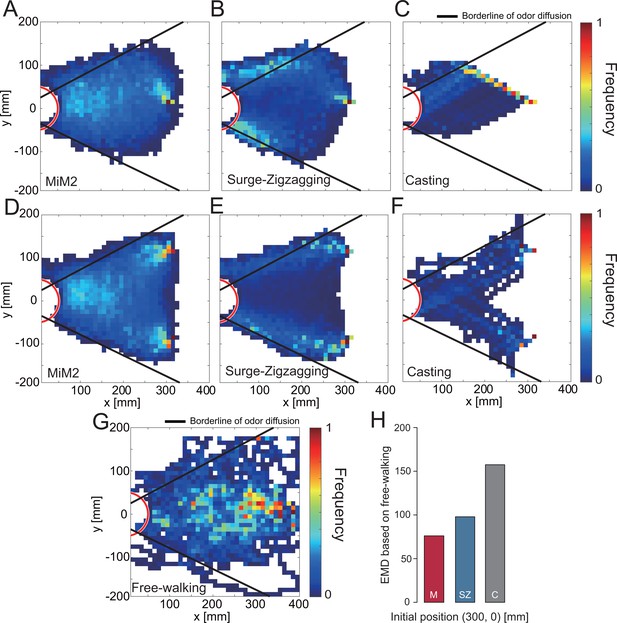
Evaluation of simulation experiments using migration probability map.
(A—F) The migration probability map at the time of success in the simulation. (G) The migration probability of an actual silkmoth when searching for a female by free walking. (H) The EMD of each search algorithm, calculated from the migration probability map from the free-walking experiment (A—C vs.G).
Tables
List of parameters for .
(a) | |||
---|---|---|---|
Odor direction = Wind direction | ≤0.7 | 5.36 | 12.9 |
>0.7 | –11.4 | 24.6 | |
Odor direction ≠ Wind direction | ≤0.7 | 0.0 | 10.5 |
>0.7 | 0.0 | 10.5 | |
(b) | |||
Odor direction = Wind direction | ≤0.4 | 0.638 | 1.40 |
>0.4 | –1.22 | 2.16 | |
Odor direction ≠ Wind direction | ≤0.4 | 0.870 | 1.42 |
>0.4 | –0.997 | 1.80 |