Assembly status transition offers an avenue for activity modulation of a supramolecular enzyme
Figures
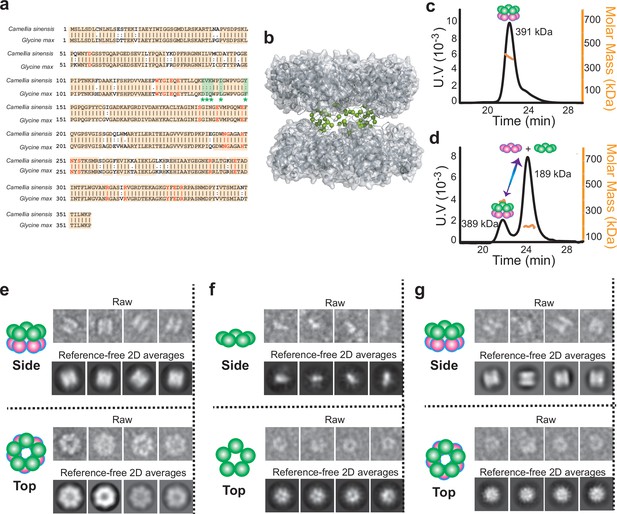
Quaternary assembly property comparison of GSIIs from Camellia sinensis (CsGSIb) and Glycine max (GmGSβ2).
(a) Amino acid sequence alignment of CsGSIb and GmGSβ2 reveals very high level of conservation. Identical amino acids are shown with orange boxes, while the residues involved in substrate-binding and catalysis are shown in red. Amino acids variations located at the pentamer ring-ring interface are highlighted in green boxes with the symbol of *. (b) Model structure built based on the crystal structure of a maize GSII (GmGSβ2, pdb code 2D3B). The amino acid variations between CsGSIb and GmGSβ2 that are located at the ring-ring interface are highlighted as spheres in green. (c–d) SEC-MALS analysis of GmGSβ2 (c) and CsGSIb (d). (e–g) Quaternary assembly analysis of GmGSβ2 and CsGSIb using negative-stain electron microscopy. Left: A schematic representation of the averages is shown for clarity; Right upper: Examples of single raw images; Right lower: reference-free two-dimensional class averages. GmGSβ2 adopts a homogenous double-ringed structure (e), while the CsGSIb demonstrates a mixture of two major classes of particles: isolated pentamer ring (f) and double-ringed structure (g).
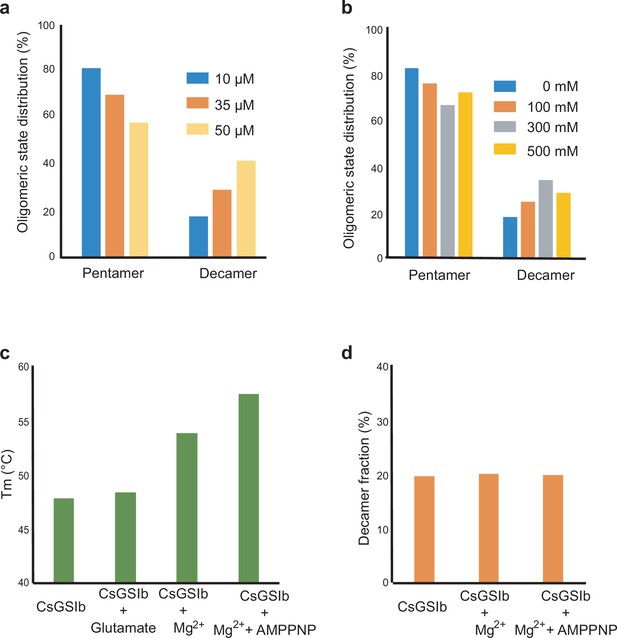
CsGs1b exhibits a pentamer-decamer dynamic equilibrium in solution.
Using SEC-MALS to evaluate the dependence of CsGSIb assembly status on: (a) protein concentrations; (b) salt concentrations. (c) Melting temperatures of CsGSIb in presence of various substrates as measured by differential scanning fluorimetry. (d) Effects of various substrates on the quaternary assembly status of CsGSIb as measured by SEC-MALS.
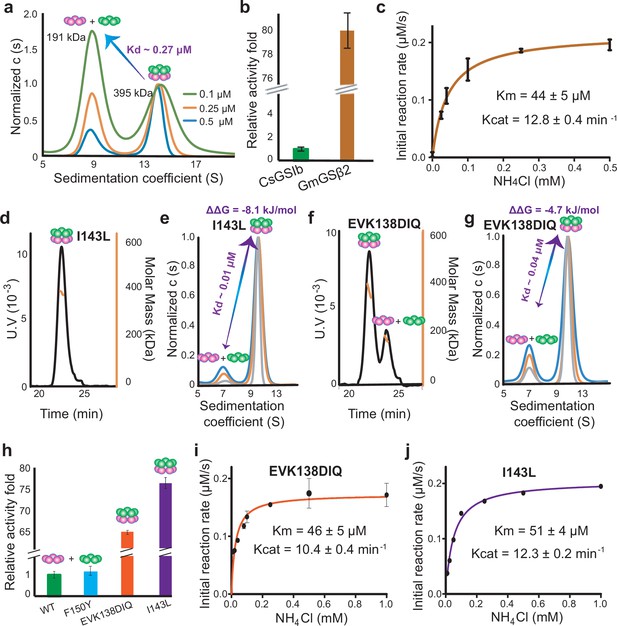
The enzymatic activities of CsGSIb is dependent on its quaternary assembly status.
(a) Application of analytical ultracentrifugation (AUC) to assess the pentamer-decamer dissociation constant of CsGSIb. Experiments were performed at room temperature with three different sample concentrations shown as monomer concentration. A global fit of sedimentation distribution profiles yielded a dissociation constant of 0.27 μM. (b) Enzymatic activity comparison of GmGSβ2 with CsGSIb. Reactions were performed for 30 min at 37 °C in presence of 1 μM (monomer) enzyme and saturated amounts of substrates. (c) Steady-state kinetic analysis of GmGSβ2. Assay conditions were the same as that in (b), except the concentrations of NH4Cl were varied. (d-g) Mutation effects on the quaternary assembly property of CsGSIb evaluated using SEC-MALS (d and f) and AUC (e and g). The corresponding mutants are labeled in the figures. (h) Activity comparison of the wild type CsGSIb with its mutants as labeled. Reactions were performed in the same condition as (b). (i-j) Steady-state kinetic analysis of CsGSIb mutants of EVK138DIQ (i) and I143L (j). Reaction conditions were same as in (c). All enzyme assays were repeated at least three times and data were shown as means ± s.d.
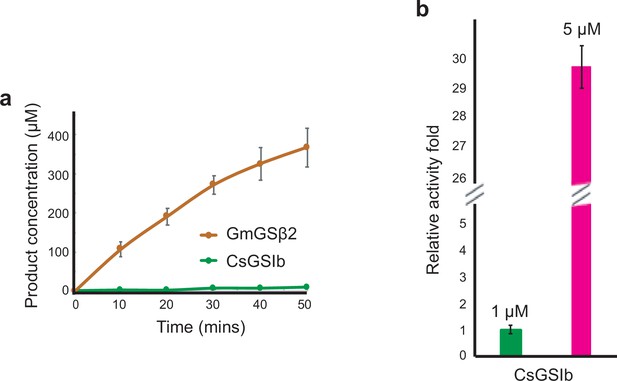
Enzymatic characterizatioin of GmGSβ2 and CsGSIb.
(a) Time courses of GS activity for GmGSβ2 (orange) and CsGSIb (green). (b) GS activity of CsGSIb demonstrates significant concentration-dependence. Green bar: 1 μM enzyme; Pink barL 5 μM enzyme, Enzyme concentrations are shown as monomer concentration. Reaction conditions are same as that in Figure 2. All experiments were repeated three times and data are shown as means ± s.d.
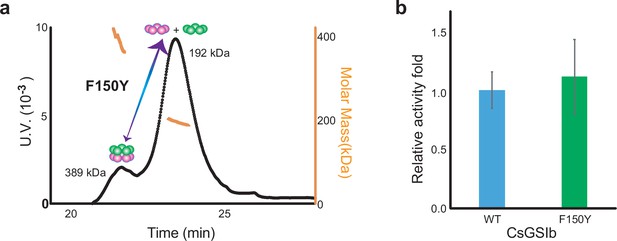
Mutation effects of F150Y on the quaternary assembly property and enzyme activity of CsGSIb.
(a) SEC-MALS profile of CsGsIb F150Y mutant reveals no change in the quaternary assembly property upon mutation. (b) Wild-type CsGsIb and F150Y demonstrate similar GS activities. Activity assays were performed three times in the condition same as that in Figure 2. Data are shown as means ± s.d.
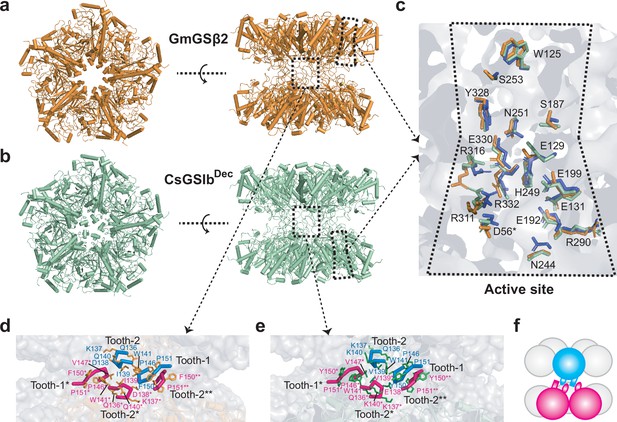
Overall structures, catalytic centers and ring-ring interfaces of GmGSβ2 and CsGSIbDec.
(a-b) Overall double-ringed structures of GmGSβ (a) and CsGSIbDec (b). Left: top-view; Right: Side-view. (c) Superimposed structures of the catalytic centers of GmGSβ2 (orange), CsGSIbDec (green) and GSII from maize (purple). High structure similarities in catalytic sites suggest the catalytic mechanism for these three GSII species are essentially identical. (d-e) The detailed ring-ring interaction interfaces between GmGSβ2 (d) and CsGSIbDec (e). The interactions between two pentameric rings are primarily mediated by two regions, namely, the tooth-1 and tooth-2, respectively. (f) A schematic representation of the two GSII pentameric rings interlocked by tooth-1 and tooth-2 for clarity.
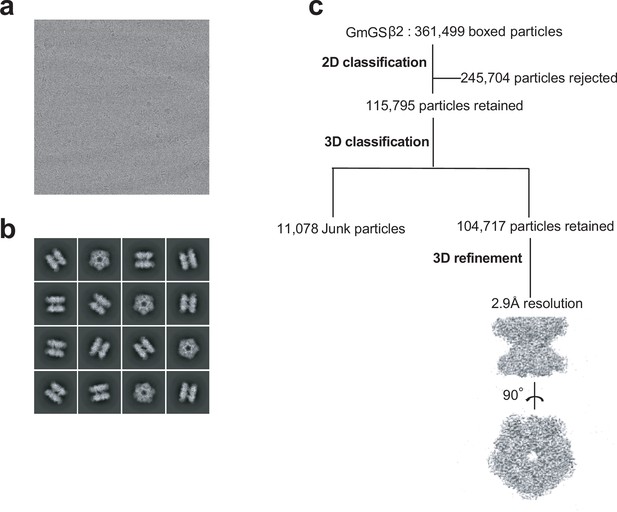
Cryo-EM analysis of GmGSβ2.
(a) Representative cryo-EM micrograph; (b) Subset of representative, reference-free 2D class averages; (c) Data processing workflow.
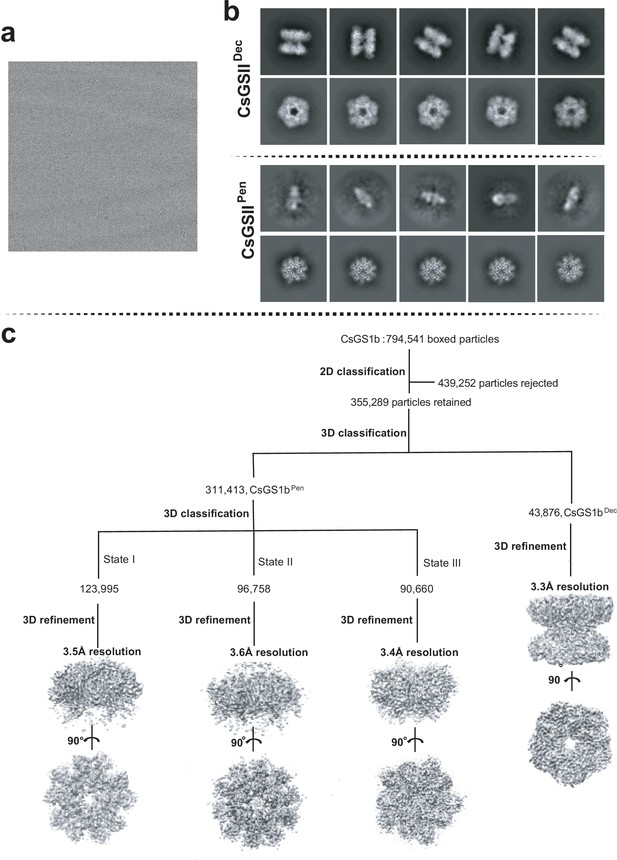
Cryo-EM analysis of CsGSIb.
(a) Representative cryo-EM micrograph; (b) Subset of representative, reference-free 2D class averages; (c) Data processing workflow.
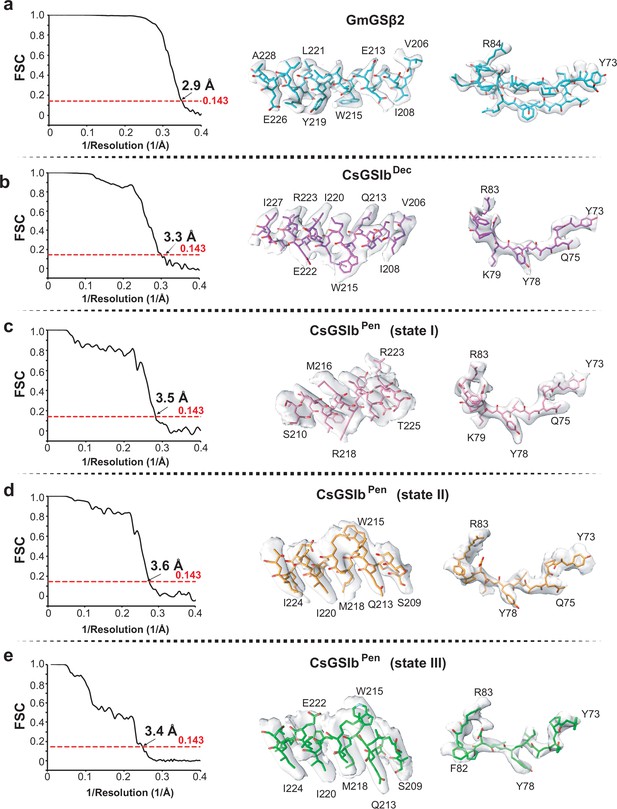
Validation of cryo-EM structures.
(a-e) GmGSβ2, CsGSIbDec, and three states of CsGSIbPen, respectively. Left: Gold-standard FSC plots generated from cryoSPARC; Right: View of model fitted in representative density.
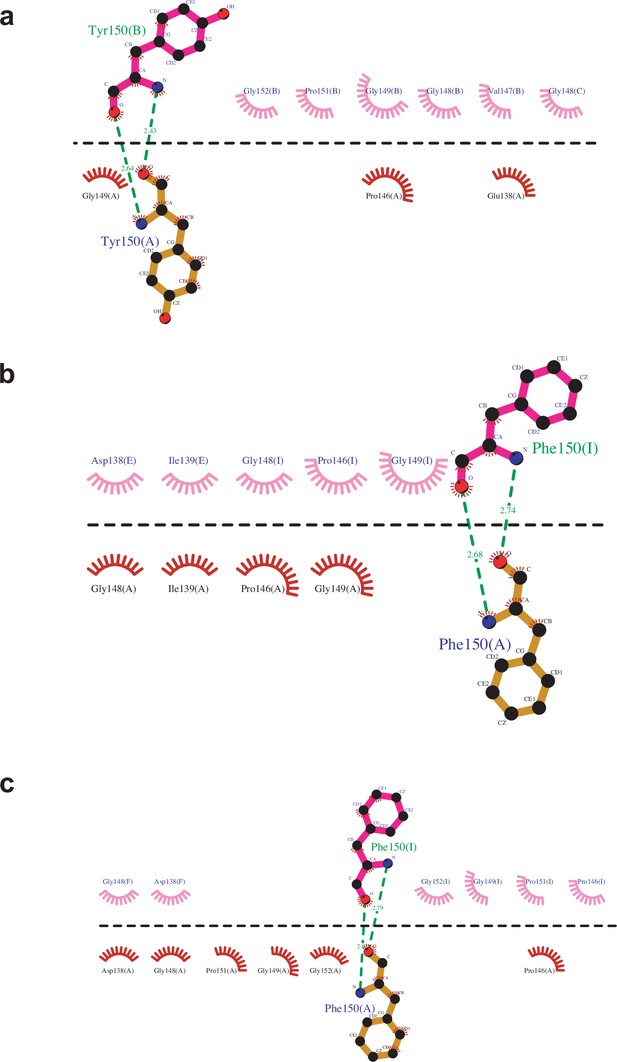
Detailed analysis of interactions between two GSII pentameric rings using the program of Ligplot.
(a) CsGSIb; (b) GmGSβ2; and (c) Maize GS using the pdb code of 2d3a.
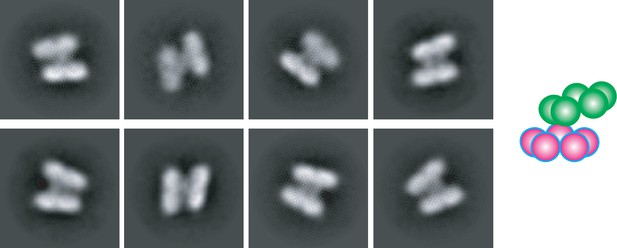
Swinging motion of CsGSIb rings.
Two-dimensional class averages of CsGsIb particles reveals a few class of particles in which two pentameric rings are no longer parallel. This swinging motion of the rings with respect to each other is likely to be owing to flexibility of the inter-ring connections. Right: A schematic representation of the averages is shown for clarity.
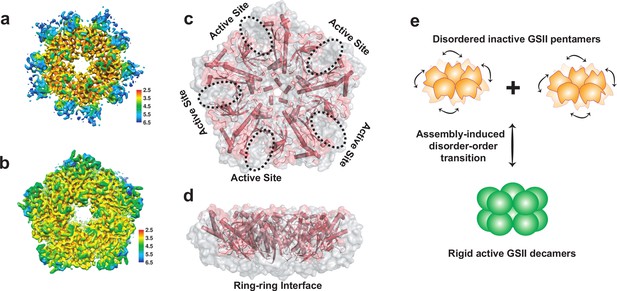
Cryo-EM structure of CsGSIbPen features high conformational flexibility.
For simplicity, only one out three CsGSIbPen cryo-EM structures are presented here, and all three structures are shown in Figure 4—figure supplement 1. (a) Local resolution of the density map of CsGSIbPen indicates a decreased resolution near the edges of the pentamer ring. (b) Local resolution of the density map of CsGSIbDec map. The conformational flexibility is apparent when the missing density at the rim of CsGSIbPen, which yields a largely decagram-shaped map (a), is compared to the intact density of CsGSIbDec that displays a pentagon-shaped map (b). (c–d) Superimposed of Cryo-EM structure of CsGSIbPen (pink) with that of CsGSIbDec (grey surface). (c): Top-view; (d): Side-view. The results reveal two major regions being highly disordered: the rim region including the catalytic center, and ring-ring interface. (e) Proposed activation mechanism model of CsGSIb.
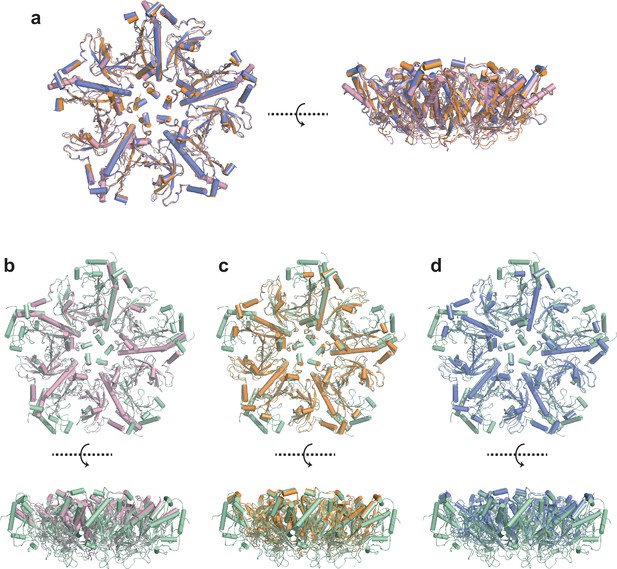
Three cryo-EM structures of CsGSIb pentamers in isolation.
(a) Structure alignment of three CsGSIbPen structures determined using Cryo-EM. Left: Topview; Right: Sideview. These three structures, colored in golden, pink and purple, respectively, are highly similar to each other, with only a few structural variations at the peripheral regions. (b–d) Structure comparison of three structures of CsGSIb pentamer in isolation (colored the same as in a) with that in the context of decamer (in color of green). Upper: Topview; Lower: Sideview. Note a large portion is missing in the structure of CsGSIbPen arising from electron density missing, indicating those regions are highly dynamic.
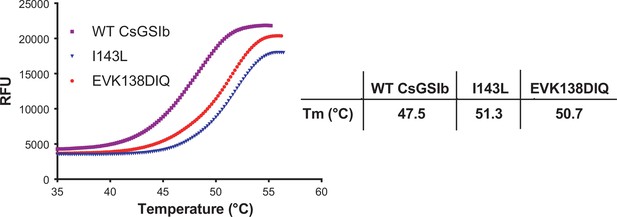
Thermal shift assays.
The thermal stabilities of wild-type CsGsII and mutants were analyzed by measuring SYPRO Orange dye fluorescence over a temperature ranging from 35 to ~55 °C using a real-time PCR thermocycler. Left: Representative unfolding curves; Right: Derived meting temperatures. RFU: Relative fluorescence unit. The lower value of meting temperature for WT CsGSIb indicates structural instability for the wild-type CsGSIb.
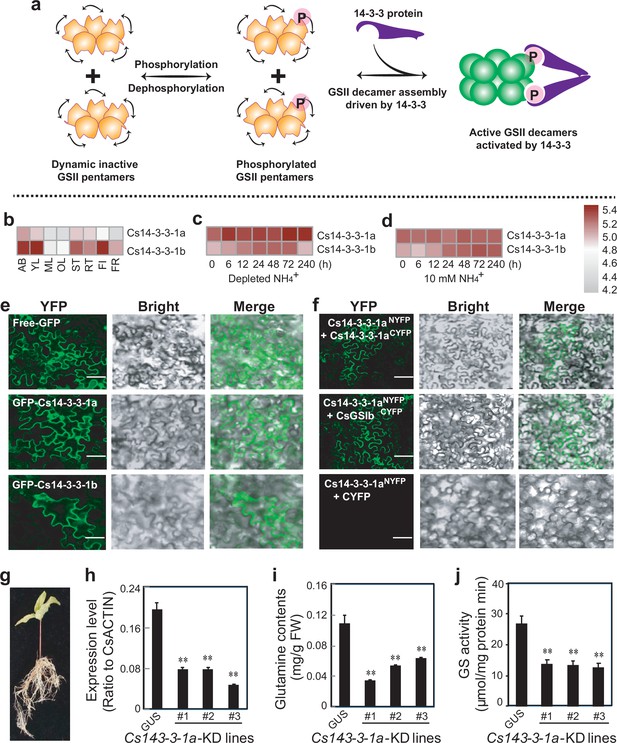
Activation of the CsGSIb by the 14-3-3 scaffold protein.
(a) Proposed working model of how 14-3-3 protein modulates the activity of GSIIs. P in pink sphere denotes the post-translational modification of phosphorylation. (b) Expression patterns of Cs14-3-3-1a and Cs14-3-3-1b genes in various tea plant tissues. (c) Induction of Cs14-3-3-1a and Cs14-3-3-1b genes by depletion of NH4+ from culture medium. (d) Expression of Cs14-3-3-1a and Cs14-3-3-1b genes in tea plant roots fed with 10 mM NH4+. AB, apical buds of unopened leaves at the top of actively growing shoots; YL, first and second young leaves below the apical buds; ML, mature leaves geminated in the spring and harvested in the autumn; OL, old leaves at the bottom of tea tree plant; FL, flowers; FR, fruits of tea plants; ST, stem tissues at the 2nd and 3rd internodes; RT, roots. (e) Subcellular localization of Cs14-3-3-1a and Cs14-3-3-1a fusion proteins in tobacco leaf epidermal cells. bar = 50 μM. (f) BIFC assay of interaction among Cs14-3-3-1a, Cs14-3-3-1b and CsGSIIb in tobacco leaf cells. bar = 50 μM. (g) Generation of tea plant transgenic hairy roots with RNAi knockdown(KD) of Cs14-3-3-1a gene. (h) Expression of Cs14-3-3-1a in at least three tea plant transgenic hairy roots of Cs14-3-3-1a-KD compared with GUS roots. i, Glutamine contents in three tea plant transgenic hairy roots of Cs14-3-3-1a-KD compared with GUS roots. (j) GS activity in three tea plant transgenic hairy roots of Cs14-3-3-1a-KD compared with GUS roots. All experiments were conducted at least three three independent experiments. At least five transgenic hairy roots for Cs14-3-3-1a and GUS genes were examined. Data are expressed as means ± s.d. Differences in two-tailed comparisons between transgenic lines and GUS controls were analyzed, **p < 0.01 in student’s t-test. See Materials and methods for experimental details.
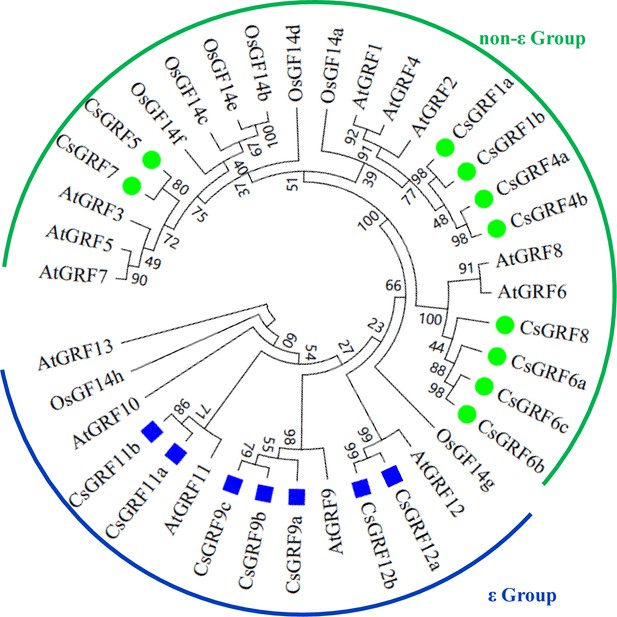
Phylogenetic analysis of Cs14-3-3s from Camellia sinensis genome as compared with 14-3-3s from Arabidopsis and rice.
Amino acid sequences were aligned by Clustal W. MEGA 6.0 software was used to construct the phylogenetic tree by the NJ method with 1000 bootstrap replicates. They are divided into two groups of non-ε Group and ε Group.
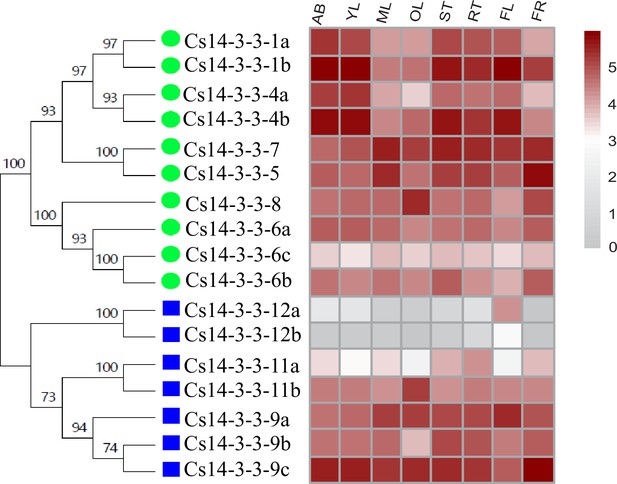
Expression of Cs14-3-3 genes in eight tissues of Camellia sinensis plants.
AB, apical bud refers to unopened leaves on the top of activity growing shoots; YL, young leaf includes the first and second leaf below the apical bud; ML, mature leaf is for these geminated in the spring and are harvested in the autumn; OL, old leaf for these in the bottom of tea tree plant; FL, Flower; FR, fruit of tea plants, ST, Stem for the 2nd, 3rd internodes; RT, roots, were retrieved from RNA Sequencing data. Expression levels were calculated using Log10 (FPKM).
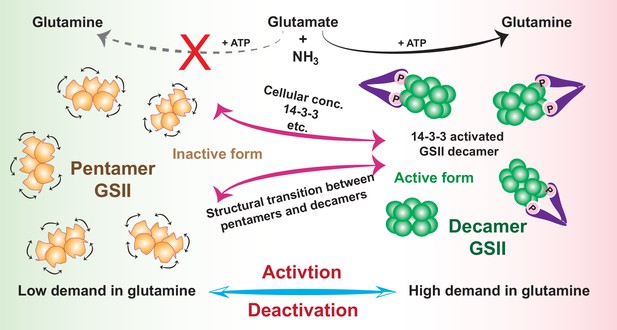
Schematic model of how activity of GSII is regulated by assembly states transition in cells to meet metabolic needs.
In low demand of glutamine, GSII is kept in the dephosphorylated state and exists as isolated inactive pentamers (orange); In the physiological context of high demand of glutamine, phosphorylation and subsequent binding to 14-3-3 (or by increase in cellular concentration) trigger a rapid transition of quaternary assembly of GSII from the pentamer to decamer (green) and activates GSII.
Tables
Reagent type (species) or resource | Designation | Source or reference | Identifiers | Additional information |
---|---|---|---|---|
Gene (Camellia sinensis) | CsGSIb | Genbank | No. MK716208 | |
Gene (Glycine max) | GmGSβ2 | Genbank | No. NM001255403 | |
Strain, strain background (Agrobacterium tumefaciens) | EHA105 strain | C58 | ||
Strain, strain background (Agrobacterium rhizogenes) | 15834 strain | ATCC | ||
Strain, strain background (Escherichia coli) | Rosetta(DE3) | Sigma-Aldrich | 70,954 | Electrocompetent cells |
Sequence-based reagent | CsGSII-1b(I143L)_FWD | This paper | PCR primers | GAAGTGAAGTGGCCGCTTGGTTGGCCTGTG |
Sequence-based reagent | CsGSII-1b (I143L)_REV | This paper | PCR primers | CACAGGCCAACCAAGCGGCCACTTCACTTC |
Sequence-based reagent | CsGSII-1b(Y150F)_FWD | This paper | PCR primers | CCTGTGGGAGGTTTTCCTGGACCACAG |
Sequence-based reagent | CsGSII-1b (Y150F)_REV | This paper | PCR primers | CTGTGGTCCAGGAAAACCTCCCACAGG |
Sequence-based reagent | CsGSII-1b (EVK138DIQ)_FWD | This paper | PCR primers | CACTTTGTTGCAGAAAGATATCC AGTGGCCGATTGGTTGGCC |
Sequence-based reagent | CsGSII-1b (EVK138DIQ)_REV | This paper | PCR primers | GGCCAACCAATCGGCCACTGGA TATCTTTCTGCAACAAAGTG |
Sequence-based reagent | Forward primer for Cs14-3-3-1a-KDKnockdown | This paper | RNAi-PCR primers | GGGGACAAGTTTGTACAAAAAAGCAGGCTT CCGACGCGCGAAGAGAACGTGT |
Sequence-based reagent | Reverse primer for Cs14-3-3-1a-KDKnockdown | This paper | RNAi-PCR primers | GGGGACCACTTTGTACAAGAAAGCTGGGTGG GAGCAAGTTCTGCACTTGC |
Sequence-based reagent | Forward primer for tagged fusion geneCs14-3-3-1a-GFP | This paper | PCR primers | GGGGACAAGTTTGTACAAAAAAGCAGGCTT CATGGCGGTGGCACCATCGACG |
Sequence-based reagent | Reverse primer for tagged fusion geneCs14-3-3-1a-GFP | This paper | PCR primers | GGGGACCACTTTGTACAAGAAAGCTGGG TTCACTTCTGTTCTTCATCTTG |
Sequence-based reagent | Forward primer for Cs14-3-3-1b-GFP-F | This paper | PCR primers | GGGGACAAGTTTGTACAAAAAAGCAGGCTT CATGGCGGTGGCAGCATCGGCA |
Sequence-based reagent | Reverse primer for Cs14-3-3-1b-GFP | This paper | PCR primers | GGGGACCACTTTGTACAAGAAAGCTGGGTT CACTGCTTTTCATCATCGTG |
Sequence-based reagent | Forward primer for Cs14-3-3-1a-CYFP-F | This paper | PCR primers | TATATTCTGCCCAAATTCGCGA TGGCGGTGGCACCATCGACG |
Sequence-based reagent | Reverse primer for Cs14-3-3-1a- CYFP-R | This paper | PCR primers | GTCGGCGAGCTGCACGCTGCCC TTCTGTTCTTCATCTTGCTT |
Sequence-based reagent | Forward primer for Cs14-3-3-1a-NYFP-F | This paper | PCR primers | TATATTCTGCCCAAATTCGCGAT GGCGGTGGCACCATCGACG |
Sequence-based reagent | Reverse primer for Cs14-3-3-1a- NYFP-R | This paper | PCR primers | CTCCTCGCCCTTGCTCACCATCTT CTGTTCTTCATCTTGCTT |
Sequence-based reagent | Forward primer for CsGSIb-CYFP-F | This paper | PCR primers | TATATTCTGCCCAAATTCGCGATG TCTCTTCTTTCCGATCTT |
Sequence-based reagent | Reverse primer for CsGSIb-CYFP-R | This paper | PCR primers | GTCGGCGAGCTGCACGCTGCCC GGTTTCCAGAGGATGGT |
Sequence-based reagent | Forward primer for CsGSIb-NYFP-F | This paper | PCR primers | TATATTCTGCCCAAATTCGCGA TGTCTCTTCTTTCCGATCTT |
Sequence-based reagent | Reverse primer for CsGSIb-NYFP-R | This paper | PCR primers | CTCCTCGCCCTTGCTCACCAT CGGTTTCCAGAGGATGGT |
Commercial assay or kit | ClonExpress II One Step Cloning Kit | Vazyme | C112-01 | |
Chemical compound, drug | AMP-PNP | Sigma Aldrich | CAS:25612-73-1 | |
Chemical compound, drug | ATP | Sigma Aldrich | CAS:34369-07-8 | |
Chemical compound, drug | L-ascorbic acid | Sigma Aldrich | CAS:50-81-7 | |
Chemical compound, drug | ammonium molybdate tetrahydrate | Sigma Aldrich | CAS:12054-85-2 | |
Chemical compound, drug | sodium citrate tribasic dehydrate | Sigma Aldrich | CAS:6132-04-3 | |
Chemical compound, drug | Methyl Alcohol | Tedia | MS1922-801 | |
Chemical compound, drug | Shigeki Konishi solution | SINOPHARM | ||
Chemical compound, drug | Glutamine | Sigma Aldrich | G3126 | |
Biological sample (Camellia sinensis) | Camellia sinensis var sinensis cultivar Shuchazao | Dechang seed seedling Co., Ltd | ||
Software, algorithm | Cryosparc | DOI:10.1038/nmeth.4169 | Cryosparc v2 | |
Software, algorithm | I-TASSER | DOI:10.1093/nar/gkv342 | I-TASSER server | |
Software, algorithm | UCSF Chimera | doi:10.1002/jcc.20084 | UCSF Chimera 1.15 | https://www.cgl.ucsf.edu/chimera |
Software, algorithm | COOT | doi:10.1107/S0907444910007493 | http://www2mrc-lmbcamach.uk/personal/pemsley/coot/ | |
Software, algorithm | Phenix | doi:10.1107/S2059798318006551 | https://www.phenix-online.org/ | |
Software, algorithm | PyMOL | PyMOL Molecular Graphics System, Schrodinger LLC | https://www.pymol.org/ |
Additional files
-
Supplementary file 1
Structural comparisons of GmGSβ2 and CsGSIbDec with glutamine synthetase from various species.
- https://cdn.elifesciences.org/articles/72535/elife-72535-supp1-v1.docx
-
Supplementary file 2
Cryo-EM data collection, refinement, and validation statistics.
- https://cdn.elifesciences.org/articles/72535/elife-72535-supp2-v1.docx
-
Transparent reporting form
- https://cdn.elifesciences.org/articles/72535/elife-72535-transrepform1-v1.docx