Metabolic sensing in AgRP neurons integrates homeostatic state with dopamine signalling in the striatum
Figures
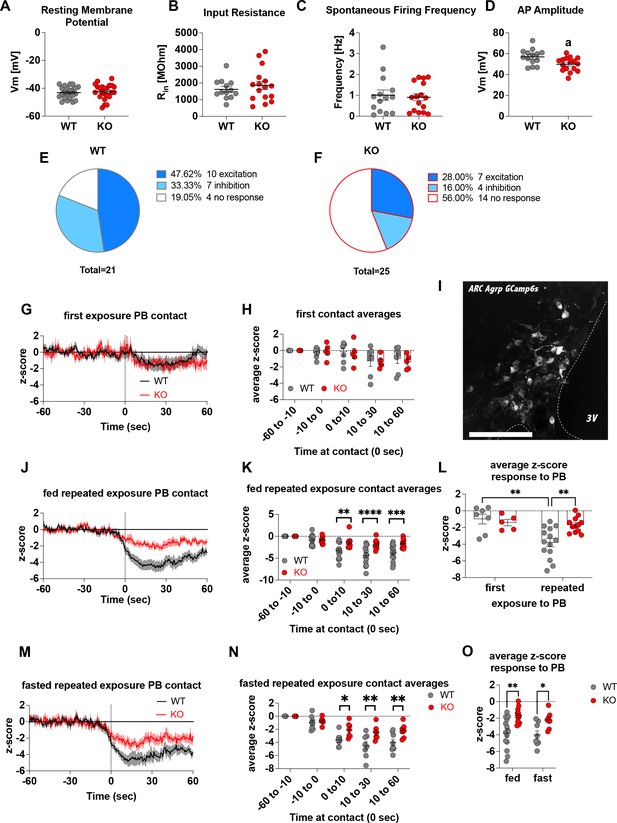
Deletion of Crat in AgRP neurons is a model of impaired metabolic sensing.
Intrinsic electrophysiological properties were little affected in WT versus KO mice including resting membrane potential (A, n = 22/23 cells WT/KO), input resistance (B n = 13/16 cells WT/KO), spontaneous action potential firing frequency (C n = 14/16 cells WT/KO), although action potential amplitude was significantly reduced in KO AgRP neurons (D, n = 14/16 cells WT/KO). Glucose-responsive profiles of AgRP neurons from WT (21 cells) and KO (25 cells) mice characterised by their response to increased extracellular glucose concentration from 2 mM to 5 mM (E–F). In vivo fiber photometry analysis of AgRP neuronal activity in WT and KO mice (G–O). First exposure to a peanut butter chip (PB) (G, n = 7 WT; n = 5 KO) and time binned summary data (H). GCaMP6s expression in AgRP neurons [scale bar = 100 µm] (I). AgRP neural activity in ad libitum-fed mice in response to repeated PB exposure (J; n = 14 WT; n = 12 KO) and time binned summary data (K–L). AgRP neural activity in fasted mice in response to repeated PB exposure (M; n = 8 WT; n = 7 KO) and time binned summary data (N), n = 8 WT, n = 7 KO). Time binned summary data comparing the response to repeated PB exposure in ad libitum-fed or fasted WT and KO mice (O). Data± SEM; ANOVA with Tukey’s post hoc analysis (H, K, L, N, O) and unpaired students t-test (A–D); a, significant at p < 0.05; ** p < 0.01, *** p < 0.001, **** p < 0.0001. Dashed lines in I, J, and M indicated the moment of contact with a PB contact.1,2 Videos 1 and 2 accompany this figure.
-
Figure 1—source data 1
Deletion of Crat in AgRP neurons is a model of impaired metabolic sensing.
- https://cdn.elifesciences.org/articles/72668/elife-72668-fig1-data1-v3.zip
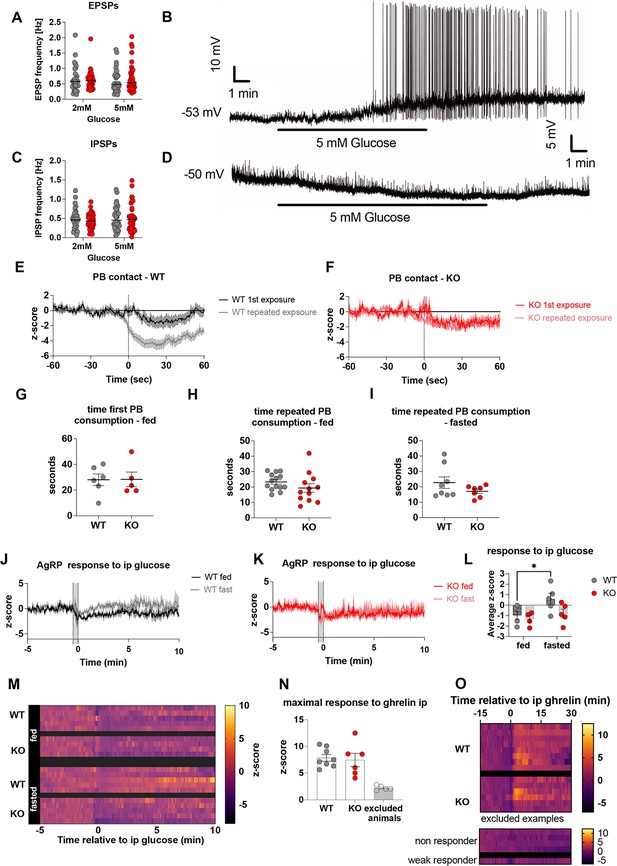
Deletion of Crat in AgRP neurons is a model of impaired metabolic sensing.
Spontaneous excitatory (A) and inhibitory (C) post-synaptic potentials compared in WT and KO mice in response to an increase in glucose from 2 mM to 5 mM. Sample traces showing examples of glucose excited (B) and glucose inhibited (D) neurons in response to an increase in glucose from 2 to 5 mM glucose. Comparison of AgRP activity at first PB and repeated PB exposure in WT (E) and KO (F) mice (WT n = 7 first exposure, n = 14 repeated; KO n = 5 first exposure, n = 12 repeated exposure). Time interval from contact with peanut butter chip until complete consumption of the ~70 mg piece in fed naïve mice (G) after repeated exposure in fed (H) and fasted mice (I). Average traces of AgRP neuron response to IP glucose (2.25 g/kg) injection in fed (n = 5) and fasted (n = 5) WT (J) and fed (n = 4) and fasted (n = 5) KO (K) mice, average z-score over 10 min post injection (L) and heatmap of traces showing individual animals (M). N, To validate GCaMP6 responsiveness in AgRP neurons, we injected IP ghrelin (1 mg/kg) and took mice with a peak maximal z-score of <4; using this criterion 5 mice were excluded from subsequent experimentation to a lack of AgRP ghrelin responsiveness. Heat maps showing the individual responses to IP ghrelin in excluded mice and those used for experiments (O). Data± SEM, * p < 0.05.
-
Figure 1—figure supplement 1—source data 1
Deletion of Crat in AgRP neurons is a model of impaired metabolic sensing.
- https://cdn.elifesciences.org/articles/72668/elife-72668-fig1-figsupp1-data1-v3.zip
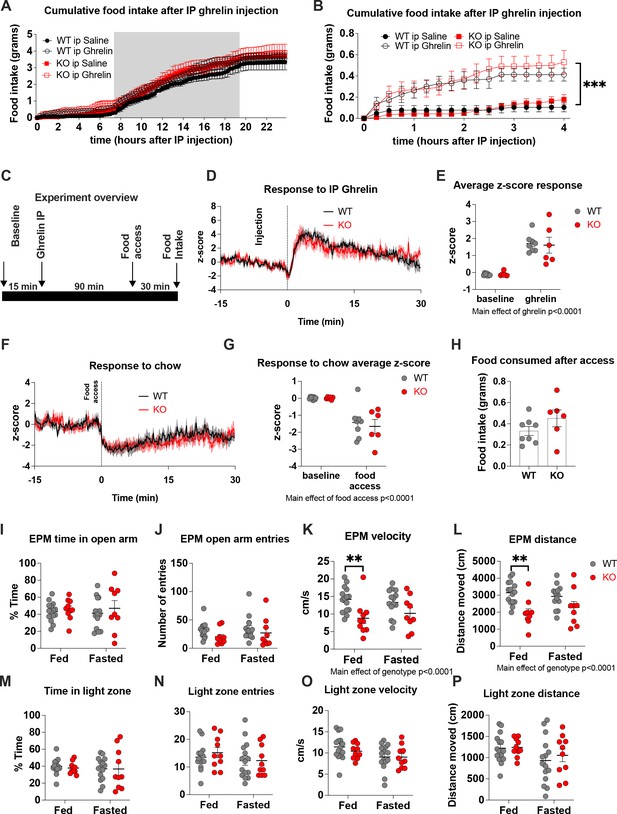
Deletion of Crat from AgRP neurons does not affect ghrelin-induced food intake or AgRP activation.
Cumulative food intake measured in BioDaq feeding cages over a 24-hr (A) and 4-hr time frame (B) in WT and KO mice treated with saline (WT n = 5, KO n = 7) and ghrelin (WT n = 5, KO n = 7). No genotypes difference in food intake were observed, however, ghrelin significantly increased food intake compared to saline (B, two-way ANOVA, main effect of treatment). Experimental approach for neural recordings of AgRP activity in response to ghrelin and subsequent chow access (C). Note, mice were treated with IP ghrelin (1 mg/kg) for 90 min before chow access to prevent confounding interactions. IP ghrelin increased AgRP neural activity in both WT and KO (D) and although we observed a main effect for ghrelin (average z-score from 0 to 30 min) to increase AgRP neural activity (E) relative to baseline (average z-score from –15–0 min), no genotype differences were observed (WT n = 8; KO n = 6; two-way ANOVA). 90 min after IP ghrelin injection mice were given access to normal chow food, which was their primary daily food source. Food access resulted in an equal suppression of AgRP neural activity in WT and KO mice (F) and although we observed a main effect of food access to reduce AgRP activity, no genotypes differences were observed (WT n = 8; KO n = 6; two-way ANOVA). No differences in food intake were observed in during the food access period (H). Analysis of anxiety-like behaviour showed no differences in time spent or entries in the open arm of the elevated-plus maze (EPM) or light zone of light-dark box (I, J, M, N). No differences were observed in distance moved or velocity in the light-dark box (O, P), although velocity and distance moved was significantly lower in KO mice in the elevated-plus maze (K, L) (WT n = 14–15, KO n = 9–10; two-way ANVOA, main effect of genotype; Tukey’s post hoc test). Data± SEM; ** p < 0.01, *** p < 0.001.
-
Figure 1—figure supplement 2—source data 1
Deletion of Crat from AgRP neurons does not affect ghrelin-induced food intake or AgRP activation.
- https://cdn.elifesciences.org/articles/72668/elife-72668-fig1-figsupp2-data1-v3.zip
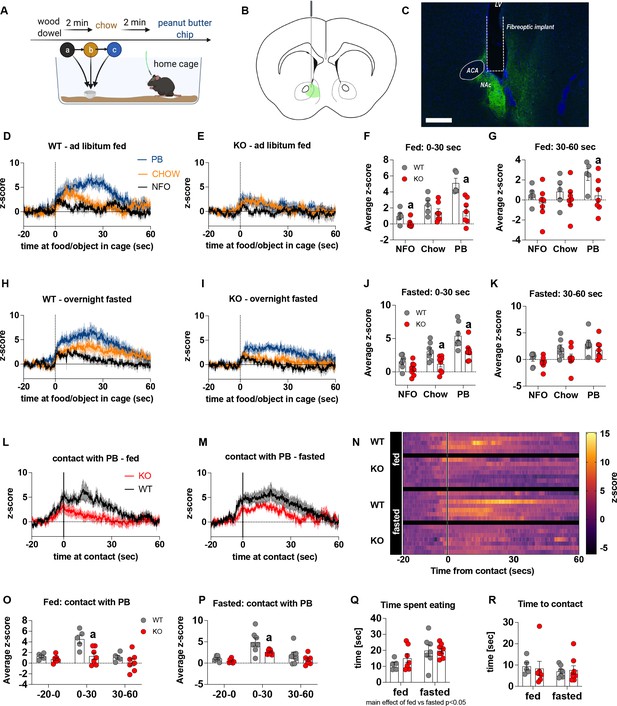
Impaired metabolic sensing in AgRP neurons affects dopamine release in the nucleus accumbens.
Experimental design to examine dopamine signalling in nucleus accumbens (A). Female (3 WT, 4 KO) and male (4 WT, 4 KO) mice, trained to receive peanut butter chips, were tethered to a fiber optic cable in their home cage. Following acclimation, dopamine responses in the nucleus accumbens to a non-food object (NFO; wood dowel, a, black), standard chow (b, tangerine), and peanut butter chip (PB, c, blue) were measured. Schematic of GRAB-DA (AAV-hSyn-DA4.3) injection site in the nucleus accumbens (B) and fiber placement [scale bar = 500 µm] (C). Average z-scored dopamine release traces aligned (time = 0) to object dropping into cage of WT and KO fed mice (D, E) or WT and KO fasted (H, I) mice. The average z-score of WT and KO ad libitum-fed mice from 0 to 30 s (F) and 30–60 s (G) in response to a non-food object (NFO), standard chow, or peanut butter chip (PB) dropped into cage. The average z-score of WT and KO fasted mice from 0 to 30 s (J) and 30–60 s (K) in response to a non-food object (NFO), standard chow, or peanut butter chip (PB) dropped into cage. Average z-score dopamine release traces aligned to first contact with peanut butter chip (PB) from fed (L) and fasted (M) WT and KO mice, with individual traces shown in a heatmap (N). The average z-score of WT and KO ad libitum-fed mice from –20–0, 0–30, and 30–60 s (O), relative to first contact. The average z-score of WT and KO fasted mice from –20–0, 0–30, and 30–60 s (P), relative to first contact. Time spent eating during the recording session (Q) and time passed between drop and contact with peanut butter (P). Data± SEM, two-way ANOVA with Tukey’s post hoc analysis (F, G, J, K, O, P, Q – main effect of fasting, p < 0.05; R); a – significant compared to WT, p < 0.05. Dashed lines at time = 0 (D, E, H, I) represent the time at which food or an object was dropped into the cage. A continuous line at time = 0 (L, M) represents the time at which mice contact PB chip. Videos 3–6 accompany this figure.
-
Figure 2—source data 1
Impaired metabolic sensing in AgRP neurons affects dopamine release in the nucleus accumbens.
- https://cdn.elifesciences.org/articles/72668/elife-72668-fig2-data1-v3.zip
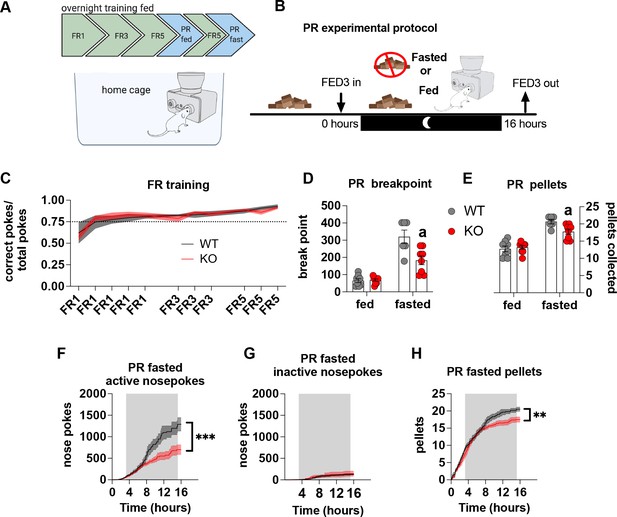
Impaired metabolic sensing in AgRP neurons affects motivation for sucrose rewards during fasting.
Mice (8 WT, 9 KO) were trained to nose poke for sucrose rewards using fixed ratio schedule (A - FR1, FR3, FR5) to reliably nose poke on average 75% correct for three consecutive nights (C), before undergoing PR schedules with or without chow accessible. For PR under fasting conditions, mice were not fasted prior to testing but progressively fasted from the beginning until the end of the PR session (B). This important distinction tests the motivation to the progressively increasing energy deficit rather than in response to an already higher energy deficit. Breakpoint from fed and fasted mice (D) and pellet retrieval at the end of PR sessions (E). The number of active nose pokes (F), inactive (G) and pellets collected during PR fasted sessions over time. Data± SEM, two-way repeated measures ANOVA with Tukey’s post hoc analysis (F, H – main effect of genotype). Data± SEM, two-way ANOVA with Tukey’s post hoc analysis (D, E). a – significant compared to WT, p < 0.05, ** p < 0.01, *** p < 0.001.
-
Figure 3—source data 1
Impaired metabolic sensing in AgRP neurons affects motivation for sucrose rewards during fasting.
- https://cdn.elifesciences.org/articles/72668/elife-72668-fig3-data1-v3.zip
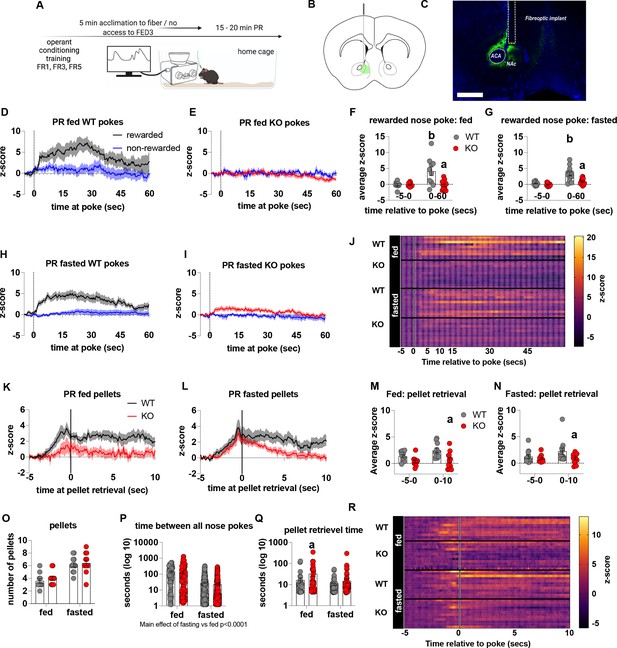
Impaired metabolic sensing in AgRP neurons reduces dopamine release in the nucleus accumbens during a progressive ratio session.
Experimental design (A) - mice with fiberoptic implant in the nucleus accumbens were trained to nose poke for a sucrose pellet prior to experimental testing. During testing, mice were tethered to a fiberoptic cable in their home cage and after 5 min acclimation, mice gained access to FED3 on PR schedule. Schematic of GRAB-DA (AAV-hSyn-DA4.3) injection site in the nucleus accumbens (B) and fiber placement [scale bar = 500 µm] (C). Combined average dopamine release response aligned (time = 0) to rewarded and non-rewarded correct nose pokes during a PR session from WT (D; n = 10 mice) and KO (E; n = 12 mice) ad libitum-fed mice and WT (H; n = 12 mice) or KO (I, n = 12 mice) after an overnight fast. The average z-score of rewarded nose pokes from WT and KO ad libitum-fed mice at –5–0 s and 0–60 s (F) or from WT and KO fasted mice at –5–0 s and 0–60 s (G). Heat maps of averaged responses from each experimental animal aligned to nose poke (J). Average z-score dopamine release traces aligned to pellet retrieval (time = 0) from ad libitum-fed (K) or fasted (L) WT and KO mice. The average z-score at –5–0 s and 0–10 s after pellet retrieval in ad libitum-fed (M) or fasted mice (N). Rewarded nose pokes /Pellets received (O) and the time between rewarded and non-rewarded nose pokes during the recording PR session in ad libitum-fed or fasted WT and KO mice (P). WT and KO mice with pellet retrieval time for fed and fasted WT and KO mice shown in Q. Heatmaps of averaged responses from each experimental animal aligned to pellet retrieval (R). Data± SEM, two-way ANOVA with Tukey’s post hoc analysis. a – significant compared to WT, p < 0.05, b – significant compared to WT –5–0 s, p < 0.05. Dashed lines at time = 0 (D, E, H, I) represent the time at which a nose poke was made. A continuous solid line at time = 0 (L, M) represents the time at which mice collected a pellet from the pellet dispenser.
-
Figure 4—source data 1
Impaired metabolic sensing in AgRP neurons reduces dopamine release in the nucleus accumbens during a progressive ratio session.
- https://cdn.elifesciences.org/articles/72668/elife-72668-fig4-data1-v3.zip
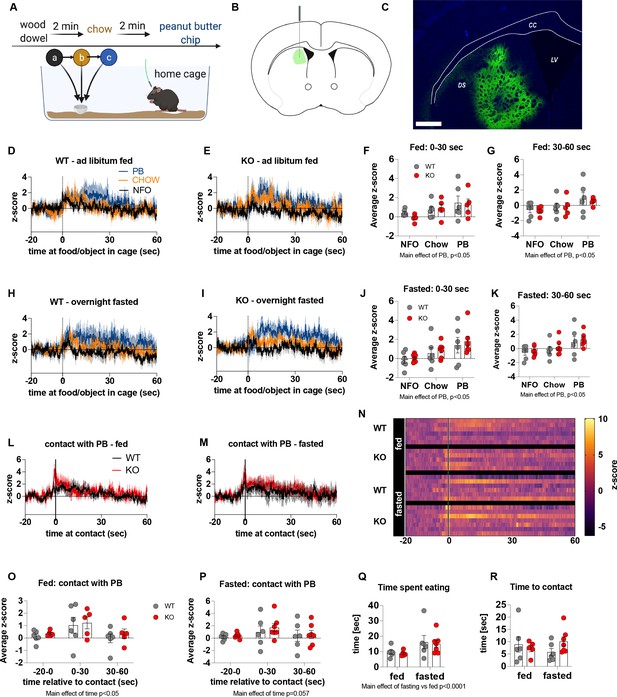
The effect of impaired metabolic sensing in AgRP neurons on dopamine release in the dorsal striatum.
Experimental design to examine dopamine signalling in dorsal striatum (A): Female (2 WT, 3 KO) and male (4 WT, 4 KO) mice, trained to receive PB chips, were tethered to a fiber optic cable in their home cage. Following acclimation, dopamine responses in the dorsal striatum to a non-food object (NFO; wood dowel, a, black), standard chow (b, tangerine), and peanut butter chip (PB, c, blue) were measured. Schematic of GRAB-DA (AAV-hSyn-DA4.3) injection site in the dorsal striatum (B) and fiber placement [scale bar = 500 µm] (C). Average z-scored dopamine release traces aligned (time = 0) to object dropping into cage of WT and KO fed mice (D, E) or WT and KO fasted (H, I) mice. The average z-score of WT and KO ad libitum-fed mice from 0 to 30 s (F) and 30–60 s (G) in response to a non-food object (NFO), standard chow, or peanut butter chip (PB) dropped into cage (n = 6 WT, n = 5 KO). The average z-score of WT and KO fasted mice from 0 to 30 s (J) and 30–60 s (K) in response to a non-food object (NFO), standard chow, or peanut butter chip (PB) dropped into cage (n = 6 WT, n = 7 KO). Average z-score dopamine release traces aligned to first contact with peanut butter chip (PB) from fed (L) and fasted (M) WT and KO mice, with individual traces shown in a heatmap (N). The average z-score of WT and KO ad libitum-fed (O) and fasted mice from –20–0, 0–30, and 30–60 s (P), relative to first contact. Time spent eating during the recording session (Q) and time passed between drop and contact with peanut butter (R). Dashed lines at time = 0 (D, E, H, I) represent the time at which food or an object was dropped into the cage. A continuous line at time = 0 (L, M) represents the time at which mice contact PB chip. Data± SEM, two-way ANOVA with Tukey’s post hoc analysis (F, G, J, K – main effects of PB, p < 0.05; (O) – main effect of time, p < 0.05; (P) – main effect of time p = 0.057; (Q) – main effect of fasting vs fed p < 0.05).
-
Figure 5—source data 1
The effect of impaired metabolic sensing in AgRP neurons on dopamine release in the dorsal striatum.
- https://cdn.elifesciences.org/articles/72668/elife-72668-fig5-data1-v3.zip
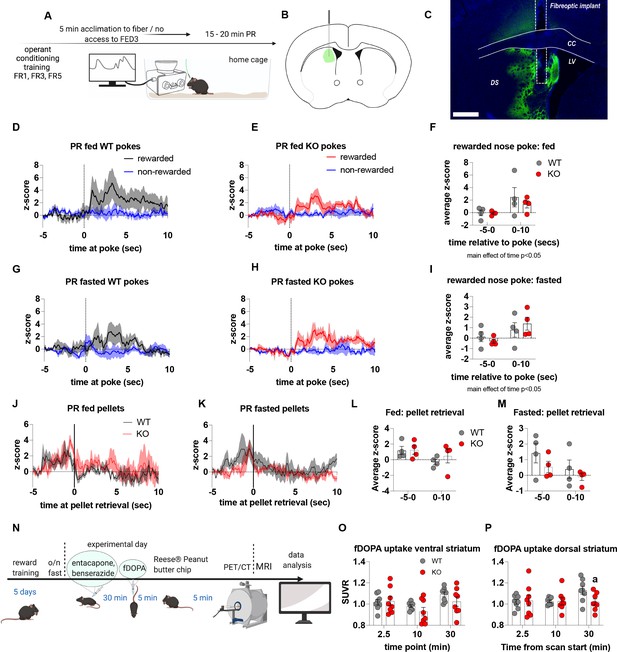
Impaired metabolic sensing in AgRP neurons does not affect dopamine release in the dorsal striatum during a progressive ratio session.
Experimental design (A) - mice with a fiberoptic implant in the dorsal striatum were trained to nose poke for a sucrose pellet prior to experimental testing. During testing, mice were tethered to a fiber optic cable in their home cage and after 5 min acclimation, mice gained access to FED3 on PR schedule. Schematic of GRAB-DA (AAV-hSyn-DA4.3) injection site in the dorsal striatum (B) and fiber placement [scale bar = 500 µm] (C). After 5 min acclimation mice gained access to FED3 on PR schedule. Combined average dopamine response of rewarded and non-rewarded correct nose pokes of 4 WT (D) and 4 KO (E) in fed state and 4 WT (G) and 4 KO (H) after overnight fast. The average z-score of rewarded nose pokes from WT and ad libitum-fed mice at –5–0 s and 0–10 s (F) or from WT and fasted mice at –5–0 s and 0–10 s (I). Average z-score dopamine release traces aligned to pellet retrieval (time = 0) from ad libitum-fed (J) or fasted (K) WT and KO mice. The average z-score at –5–0 s and 0–10 s relative to pellet retrieval in ad libitum-fed (L) or fasted (M) WT and KO mice. Experimental design for fDOPA PET scan (N): Mice (WT n = 8 male, KO n = 8 male) were trained to receive Reese peanut butter (PB) chips and then fasted overnight before the experimental day. On the experimental day, mice received ip injection of benserazide and entacapone to prevent peripheral breakdown of fDOPA (iv injection 30 min later). Mice were allowed to recover 5 min and then given one peanut butter chip, which they ate within 5 min. Then mice were anaesthetised and prepared for PET/CT scan and dopamine uptake in dorsal and ventral striatum measured. Baseline fDOPA uptake in ventral striatum (O) and dorsal striatum (P). Dashed lines at time = 0 (D, E, G, H) represent the time at which a nose poke was made. A continuous line at time = 0 (J, K) represents the time at which mice collected the pellet from the pellet dispenser. Data± SEM, two-way ANOVA with Tukey’s post hoc analysis (F, I, L, M – main effect of time, p < 0.05). a – significant compared to WT, p < 0.05.
-
Figure 6—source data 1
Impaired metabolic sensing in AgRP neurons does not affect dopamine release in the dorsal striatum during a progressive ratio session.
- https://cdn.elifesciences.org/articles/72668/elife-72668-fig6-data1-v3.zip
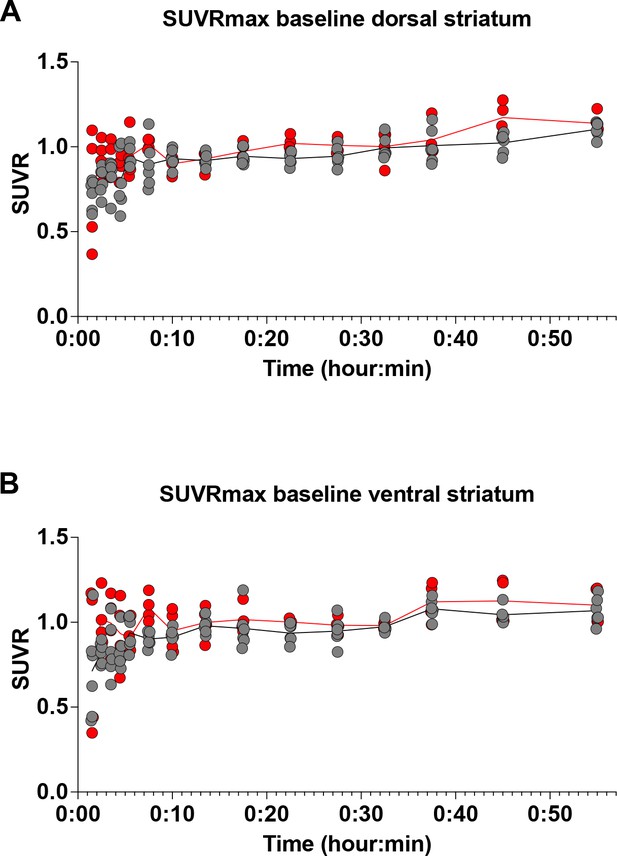
Dynamic basal dopamine uptake in dorsal (A) and ventral striatum (B) in fasted mice without reward presentation and observed no differences in fDOPA uptake.
-
Figure 6—figure supplement 1—source data 1
Dynamic basal dopamine uptake in dorsal and ventral striatum in fasted mice without reward presentation and observed no differences in fDOPA uptake.
- https://cdn.elifesciences.org/articles/72668/elife-72668-fig6-figsupp1-data1-v3.zip
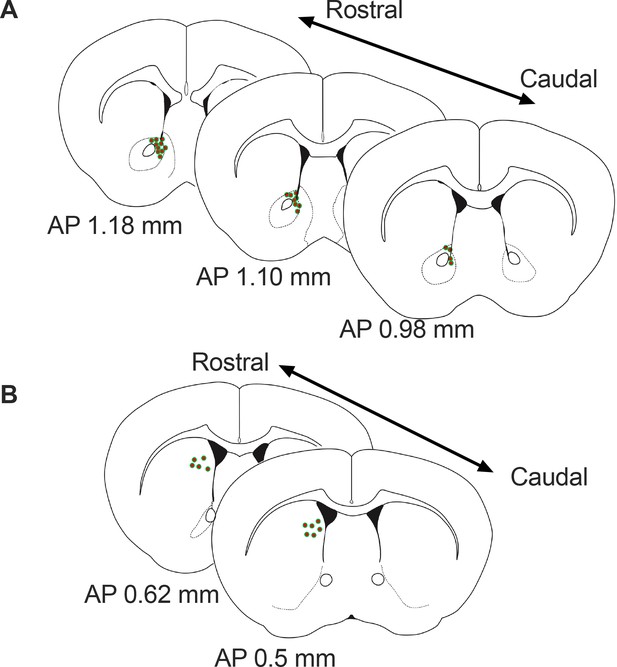
Schematic representation of the approximate viral injections sites for GRAB-DA in the Nucleus Accumbens (A) and dorsal striatum (B).
AP = anterior posterior position relative to bregma.
Videos
GCaMP6s AgRP neural activity time locked to behaviour at 10 x normal speed in an ad libitum-fed WT mouse previously exposed to a peanut butter pellet.
The video shows raw data collected in mV at the photoreceiver prior to df/f calculations.
GCaMP6s AgRP neural activity time locked to behaviour at 10 x normal speed in an ad libitum-fed KO mouse previously exposed to a peanut butter pellet.
The video shows raw data collected in mV at the photoreceiver prior to df/f calculations.
GRAB-DA activity (dopamine release) time locked to behaviour at 10 x normal speed in an ad libitum-fed WT mouse.
The mouse is first exposed to wood dowel, followed by chow, followed by a ~ 70 mg PB chip. The video shows raw data collected in mV at the photoreceiver prior to df/f calculations.
GRAB-DA activity (dopamine release) time locked to behaviour at 10 x normal speed in a fasted WT mouse.
The mouse is first exposed to wood dowel, followed by chow, followed by a ~ 70 mg PB chip. The video shows raw data collected in mV at the photoreceiver prior to df/f calculations.
GRAB-DA activity (dopamine release) time locked to behaviour at 10 x normal speed in an ad libitum-fed KO mouse.
The mouse is first exposed to wood dowel, followed by chow, followed by a ~ 70 mg PB chip. The video shows raw data collected in mV at the photoreceiver prior to df/f calculations.
GRAB-DA activity (dopamine release) time locked to behaviour at 10 x normal speed in a fasted KO mouse.
The mouse is first exposed to wood dowel, followed by chow, followed by a ~ 70 mg PB chip. The video shows raw data collected in mV at the photoreceiver prior to df/f calculations.