The LRRK2 G2019S mutation alters astrocyte-to-neuron communication via extracellular vesicles and induces neuron atrophy in a human iPSC-derived model of Parkinson’s disease
Figures
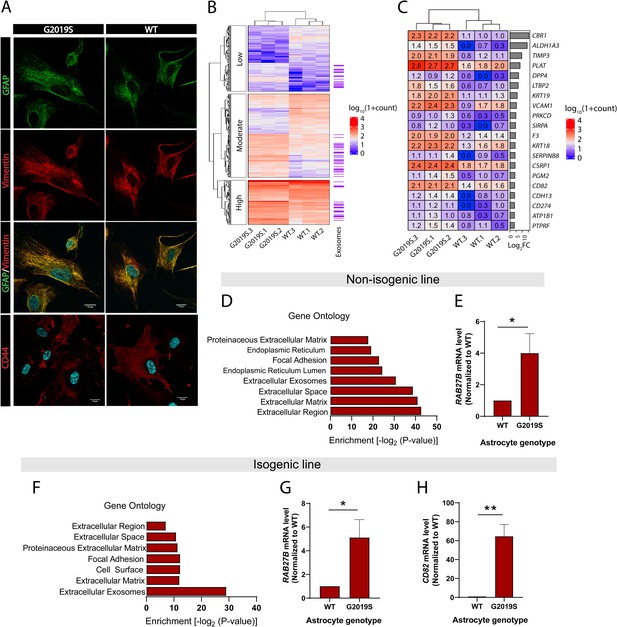
Expression of exosome components is dysregulated in LRRK2 G2019S astrocytes.
(A) Astrocytes were prepared from induced pluripotent stem cells (iPSCs) carrying the Parkinson’s disease (PD) mutation LRRK2 G2019S or its isogenic control. Confocal images of immunostained iPSC-derived astrocytes show expression of astrocyte markers GFAP (green), vimentin (red), merged GFAP (green) and vimentin (red) with the nuclear marker DAPI (blue), and merged astrocyte marker CD44 (red) with DAPI (blue). (B) Heatmap representing the hierarchical clustering of significantly upregulated and downregulated genes in LRRK2 G2019S vs. WT astrocytes using a 1.4-fold threshold for upregulated genes and a 0.7-fold threshold for downregulated genes, and a false discovery rate of 0.05. Sequencing counts were normalized using the median of ratios method and calculated by the EBseq package in R, as described in Materials and methods, then transformed using log10(1 + normalized sequencing counts). The genes are separated into three categories based on their log10(1 + normalized sequencing counts) value using k-means clustering with k = 3 to reveal groups of low-, moderate-, or high-expression genes. Genes encoding exosome-related components are indicated on the right (purple lines, with each indicating one gene). (C) Heatmap representing the differential expression of 20 genes encoding exosome components in WT and LRRK2 G2019S astrocytes, across three independent biological replicates (labeled 1–3). The values represent the log10(1 + normalized sequencing counts) transformation as described in Materials and methods, log2FC represents LRRK2 G2019S vs. WT fold change in gene expression. Genes are sorted by their log2FC value in order of descending fold change. (D–H) Gene ontology analysis of non-isogenic and isogenic lines showing upregulated components identified by RNA sequencing, and Benjamini–Hochberg adjusted p-values were obtained from the Database for Annotation, Visualization and Integrated Discovery (DAVID) tool (D, F). Gene expression validation by qPCR of two exosome components, Rab27b (E, G) and CD82 (H) in non-isogenic and isogenic lines. Data are from three (B, C, D, F) four (G, H), or five (E) independent biological replicates; error bars represent mean + standard error of the mean (SEM). Statistical analysis was performed using two-tailed unpaired Student’s t-test with equal standard deviation (s.d.) (*p≤0.05, **p<0.01).
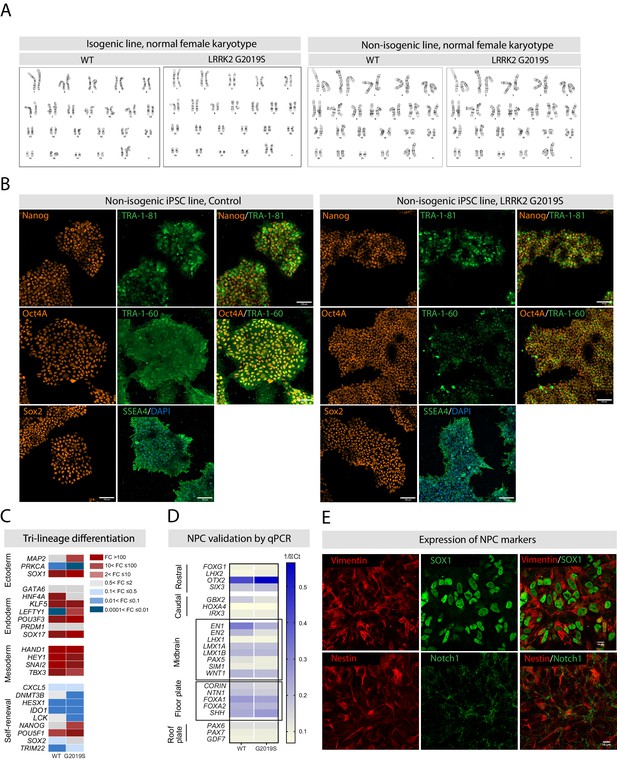
Quality control of induced pluripotent stem cells (iPSCs) and iPSC-derived neural progenitor cells (NPCs).
(A) Normal human female karyotype prepared from WT and LRRK2 G2019S iPSCs. Cytogenetic analysis was performed on 20G-banded metaphase cells for each genotype. (B) Representative confocal images of non-isogenic iPSCs reprogrammed from dermal fibroblasts collected from a healthy donor and a LRRK2 G2019S patient. Immunostaining shows expression of the pluripotency markers Nanog (red) and TRA-1-81 (green), Oct4 (red) and TRA-1-60 (green), Sox 2 (red), and SSEA4 (green). Nuclei are stained with DAPI. (C) Heatmap showing trilineage differentiation potential of the newly generated non-isogenic iPSCs via embryoid body formation and subsequent spontaneous differentiation. Data show RT-qPCR quantification of gene expression for known markers of the ectoderm, endoderm, and mesoderm lineages, as well as self-renewal genes, and are normalized to iPSC reference standards. (D) Representative heatmap showing the gene expression profile of NPCs patterned towards a midbrain fate. The left side of the heatmap identifies gene markers for specific brain regions (Kirkeby et al., 2012). Successfully differentiated NPCs should show higher expression levels of genes shown in the two black boxes (midbrain and floor plate) compared to other genes (Kirkeby et al., 2012; Kriks et al., 2011). The scale is calculated based on the 1/Δ cycle threshold (Ct) values calculated from the Ct of the gene of interest compared to the Ct of the loading control β-actin. (E) Confocal images of immunostained NPCs show expression of NPC markers vimentin (red), SOX1 (green), and merged vimentin (red) and SOX1 (green). The lower panel shows the expression of the markers nestin (red), notch1 (green), and merged nestin (red) and notch1 (green).
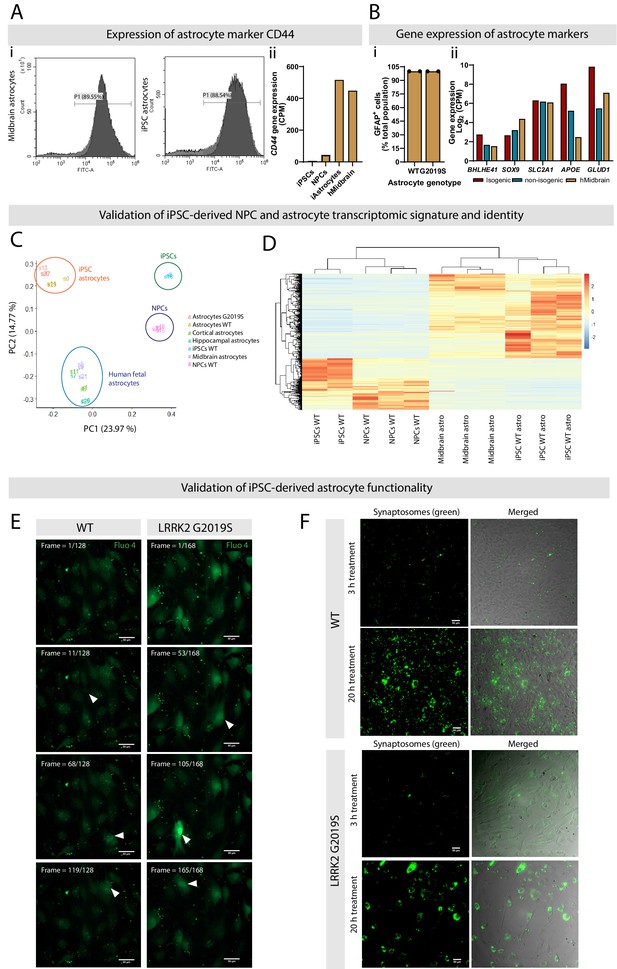
Quality control of induced pluripotent stem cell (iPSC)-derived astrocytes.
(A) Histogram representing the proportion of astrocytes expressing the marker CD44 by flow cytometry in cultures prepared from human fetal midbrain astrocytes (89.55% CD44+ cells) or iPSC-derived astrocytes (88.54% CD44+ cells). A total of 10,000 events were recorded for each experimental condition (Ai). CD44 gene expression in iPSCs, neural progenitor cells (NPCs), iPSC-derived astrocytes (iAstrocytes), or human fetal midbrain astrocytes (hMidbrain) collected by RNA-seq and expressed as CPM values (Aii). (B) Quantification of GFAP+ astrocytes in cultures differentiated from WT or LRRK2 G2019S iPSCs. Data are from two independent biological replicates, and at least 260 cells were counted per experimental condition (Bi). Gene expression of astrocyte markers in astrocytes derived from isogenic and non-isogenic iPSC lines, as well as human fetal midbrain astrocytes to use as a reference. Data were collected by RNA-seq and shown as the mean log2(CPM) values (Bii). (C, D) To confirm the successful differentiation of iPSCs into specific cell types, we performed RNA-seq of iPSCs, NPCs, iPSC-derived astrocytes, and human fetal astrocytes. RNA-seq data was analyzed by principal component analysis (PCA) (E) and a heatmap representing the unsupervised cluster analysis was generated (F); data are from two (iPSCs) or three (NPCs, iPSC-derived astrocytes and fetal astrocytes) independent biological replicates. (E, F) Representative wide-field images showing transient calcium signals using the green Fluo-4 indicator (E) and internalization of green-labeled synaptosomes (H) in WT and LRRK2 G2019S iPSC-derived astrocytes. Arrowheads in (E) show cells with transient increase in Fluo-4 signal indicative of calcium influx. Abbreviation: CPM, counts per million.
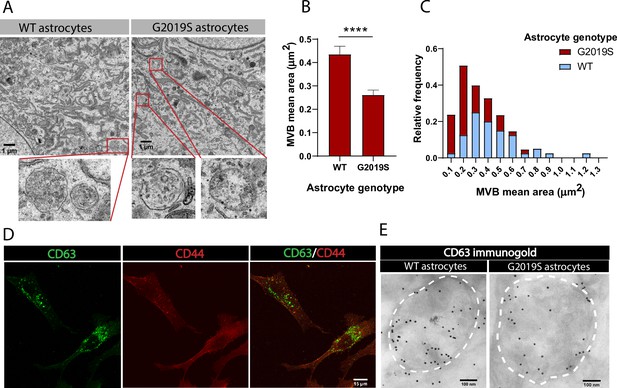
Multivesicular bodies (MVBs) in LRRK2 G2019S astrocytes are smaller than in WT astrocytes.
(A) Transmission electron microscopy (TEM) images of MVBs in WT and LRRK2 G2019S astrocytes. For illustration purposes, the red boxes in the top panel indicate MVBs in the cytoplasm of astrocytes and the lower panel shows a zoomed-in view of the MVBs. (B, C) Quantification of mean area (B) and size distribution (C) of MVBs identified in TEM images of WT and LRRK2 G2019S astrocytes. Data are sampled from at least 20 cells (≥40 MVBs) in each experimental condition; error bars represent mean + SEM for two independent biological samples (B). Statistical analysis was performed using two-tailed unpaired Student’s t-test with equal s.d. (****p<0.0001). (D) Representative confocal images of astrocytes labeled by immunofluorescence with the exosome marker CD63 (green) and the astrocyte marker CD44 (red). (E) Electron microscopy image showing immunogold labeling of CD63 (large gold) in astrocytes. Dashed lines delineate MVB membranes.
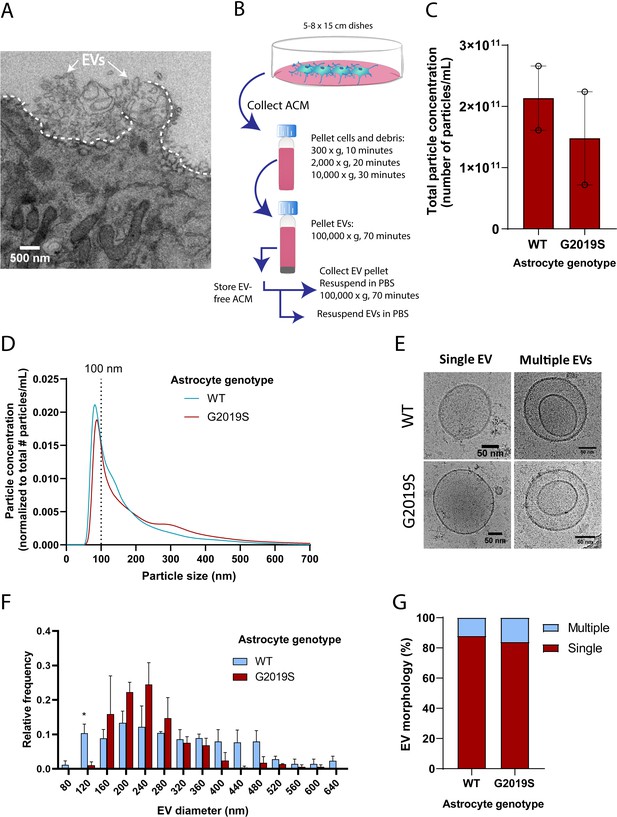
The size distribution of extracellular vesicles (EVs) secreted by astrocytes is altered in the LRRK2 G2019S mutant.
(A) Transmission electron microscopy (TEM) images demonstrate that induced pluripotent stem cell (iPSC)-derived astrocytes actively produce and secrete EVs by exocytosis. The dashed line delineates the plasma membrane. The white arrows indicate EVs. (B) Overview of the procedure to isolate EVs by ultracentrifugation. ACM: astrocyte conditioned medium; Cryo-EM: cryogenic electron microscopy. (C) Nanoparticle tracking analysis (NTA) quantification of the number of particles (i.e., EVs) isolated in WT or LRRK2 G2019S ACM by ultracentrifugation as described in (B). (D) Graph showing the distribution of isolated EVs by particle size. Data in (C, D) are from two independent biological replicates; error bars represent mean + SEM. Statistical analysis was performed using two-tailed unpaired Student’s t-test with equal s.d. (ns: not significant). (E–G) Secreted EVs were imaged by cryo-EM (E) and their diameter (F) and morphology (G) was analyzed. Data are from ≥177 EVs isolated from ACM taken from 30.4 × 106 plated astrocytes for each experimental condition; error bars are mean + SEM for three independent biological replicates. Statistical analysis was performed using two-tailed unpaired Student’s t-test with equal s.d. (*p≤0.05).
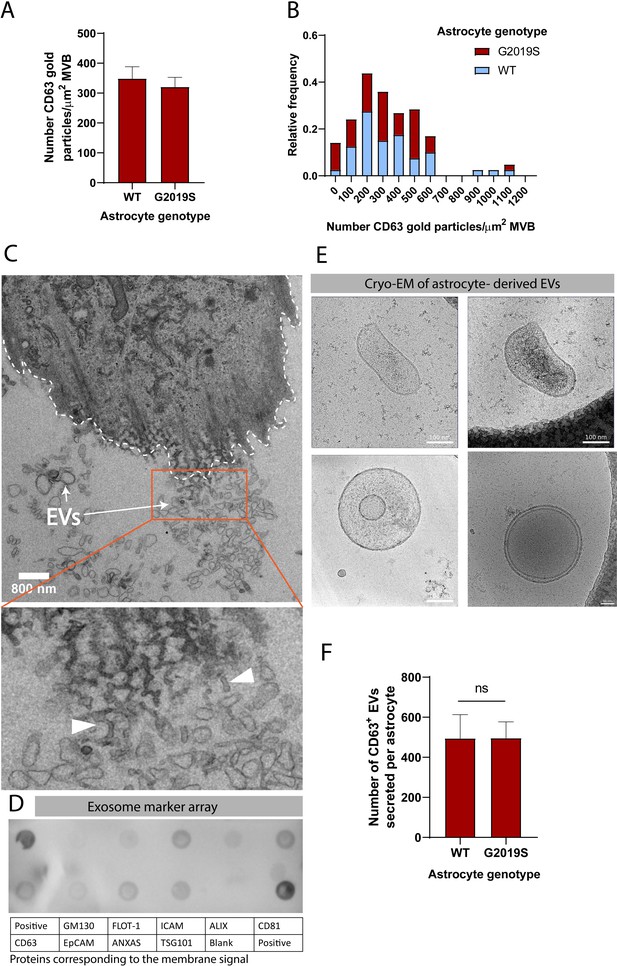
Analysis of astrocyte-secreted extracellular vesicles (EVs).
(A, B) Quantification of CD63 gold particles shown as the number of particles per µm2 multivesicular body (MVB) (A) and relative frequency (B) in WT vs. LRRK2 G2019S astrocytes. Error bars represent mean + SEM. (C) Transmission electron microscopy (TEM) image of an astrocyte and astrocyte-secreted EVs. The dashed line indicates the astrocyte cell membrane, and the arrows indicate EVs. The red box and the zoomed-in view show EVs that appear to bud from the astrocyte membrane (white arrowheads). (D) Identification of exosome markers in EV-enriched fractions obtained from astrocyte conditioned media (ACM) using an exosome antibody array. Each circle on the membrane represents a preprinted antibody spot marker of exosome or cellular contaminant, and the table details the name of each antibody marker spotted on the membrane. (E) Cryo-electron microscopy (cryo-EM) images of astrocyte-derived EVs that display an unusual morphology. (F) Quantification of the number of CD63+ EVs secreted in WT and LRRK2 G2019S ACM by ELISA using ELISA standards calibrated by nanoparticle tracking analysis (NTA) as discussed in Materials and methods. Data are from at least three independent biological replicates; error bars represent mean + SEM. Statistical analysis was performed using two-tailed unpaired Student’s t-test with equal s.d.
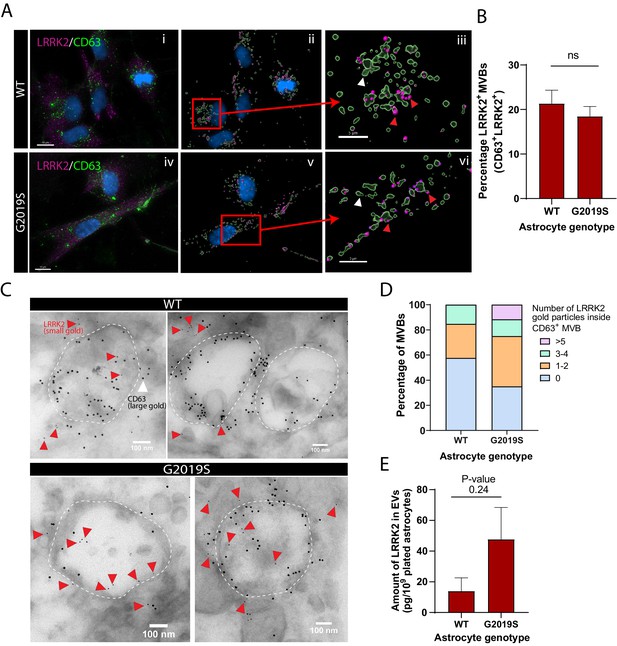
LRRK2 is associated with multivesicular bodies (MVBs) in astrocytes and accumulates in the MVBs of LRRK2 G2019S mutant.
(A) Representative images of WT and LRRK2 G2019S isogenic astrocytes labeled by immunofluorescence with CD63 (green), LRRK2 (purple), and DAPI nuclear stain (blue) (Ai, Aiv). The images were analyzed using Imaris software to identify CD63+ MVBs (green surfaces) colocalized with LRRK2 (purple dots) and show the localization of the nucleus (DAPI, blue) (Aii, Av). Zoomed-in images shows two populations of CD63+ surfaces: CD63+/LRRK2+ (red arrowhead), and CD63+/LRRK2- (white arrowhead) (Aiii, Avi). (B) Percentage of CD63-labeled surfaces that are also LRKK2 positive in WT and LRRK2 G2019S astrocytes, quantified with Imaris software using object-based colocalization. Data are from three independent biological replicates, and ≥40 astrocytes (>3000 MVBs) were analyzed per experimental condition; error bars represent mean + SEM. Statistical analysis was performed using two-tailed unpaired Student’s t-test with equal s.d. (ns: not significant). (C) Immunogold electron microscopy shows the presence of LRRK2 (small gold, red arrowheads) inside and in the vicinity of CD63+ MVBs (large gold) in WT and LRRK2 G2019S astrocytes. The dashed lines indicate the contour of MVBs. (D) Distribution of CD63+ MVBs according to their number of internal LRRK2 gold particles. Data are sampled from at least 20 astrocytes (≥59 MVBs) in each experimental condition. The distribution is significantly different in LRRK2 G2019S astrocytes compared to WT astrocytes (p-value = 0.0084, chi-square test). (E) Quantification of the amount of LRRK2 in WT or LRRK2 G2019S EV-enriched fractions by ELISA. Data are from at least three independent biological replicates; error bars represent mean + SEM; statistical analysis was performed using two-tailed unpaired Student’s t-test with equal s.d. (ns: not significant).
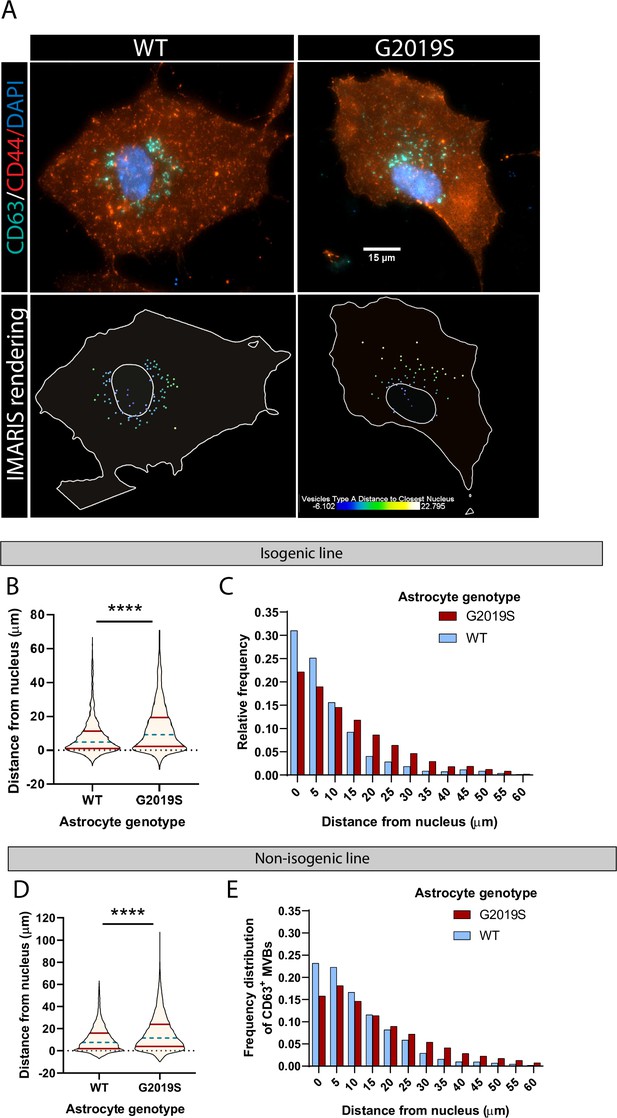
The spatial distribution of multivesicular bodies (MVBs) in LRRK2 G2019S astrocytes is altered.
(A) Representative immunofluorescence images of WT and LRRK2 G2019S astrocytes labeled with the exosome marker CD63 (green), the astrocyte marker CD44 (red), and the nuclear marker DAPI (dark blue). The bottom images show the corresponding Imaris software rendering of CD63+ MVBs, color-coded by distance to the nucleus, from blue (closest) to white (farthest). The plain white lines indicate the cell boundary (outer line) and nucleus (inner circle). (B–E) Quantification of the distance of CD63+ MVBs from the nuclear membrane in WT and LRRK2 G2019S isogenic (B, C) or non-isogenic (D, E) astrocytes using Imaris software ‘vesicles distance to closest nucleus’ calculation. The violin plot shows the median (blue dashed line) and interquartile range (red solid line) (B, D). Data are from three independent biological replicates, 40–70 astrocytes (>3300 MVBs) were analyzed for each experimental condition. Statistical analysis was performed using a Mann–Whitney test (****p<0.0001).
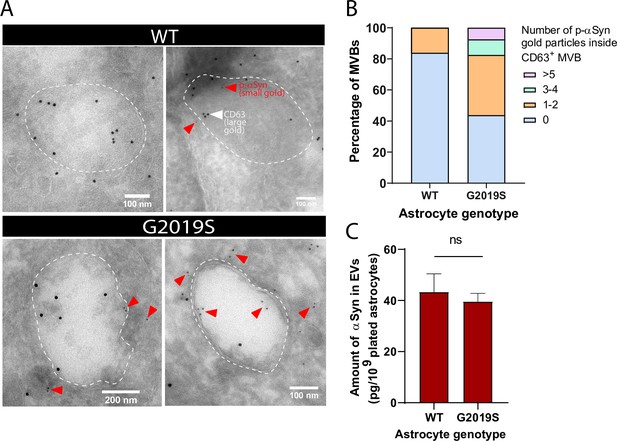
Alpha-synuclein and phospho-S129 alpha-synuclein are associated with multivesicular bodies (MVBs) in astrocytes and accumulate in the MVBs of LRRK2 G2019S mutant.
(A) Immunogold labeling of phospho-S129 alpha-synuclein (p-αSyn, small gold, red arrowheads) and CD63 (large gold) shows localization of p-αSyn inside and in the vicinity of MVBs in astrocytes. The low abundance of small gold particles in the control sample is consistent with the observation that healthy brain tissues contain low levels of p-αSyn (Fujiwara et al., 2002). The dashed lines indicate the boundary of the MVBs. (B) Distribution of CD63+ MVBs according to their number of internal p-αSyn gold particles, in WT and LRRK2 G2019S astrocytes. Data are sampled from at least 20 astrocytes (30–79 MVBs) for each experimental condition. The distribution is significantly different in LRRK2 G2019S astrocytes compared to WT astrocytes (p-value=0.0014, chi-square test). (C) Quantification of the amount of αSyn in WT and LRRK2 G2019S extracellular vesicle (EV)-enriched fractions by ELISA. Data are from seven independent biological replicates; error bars represent mean + SEM; statistical analysis was performed using two-tailed unpaired Student’s t-test with equal s.d. (ns: not significant).
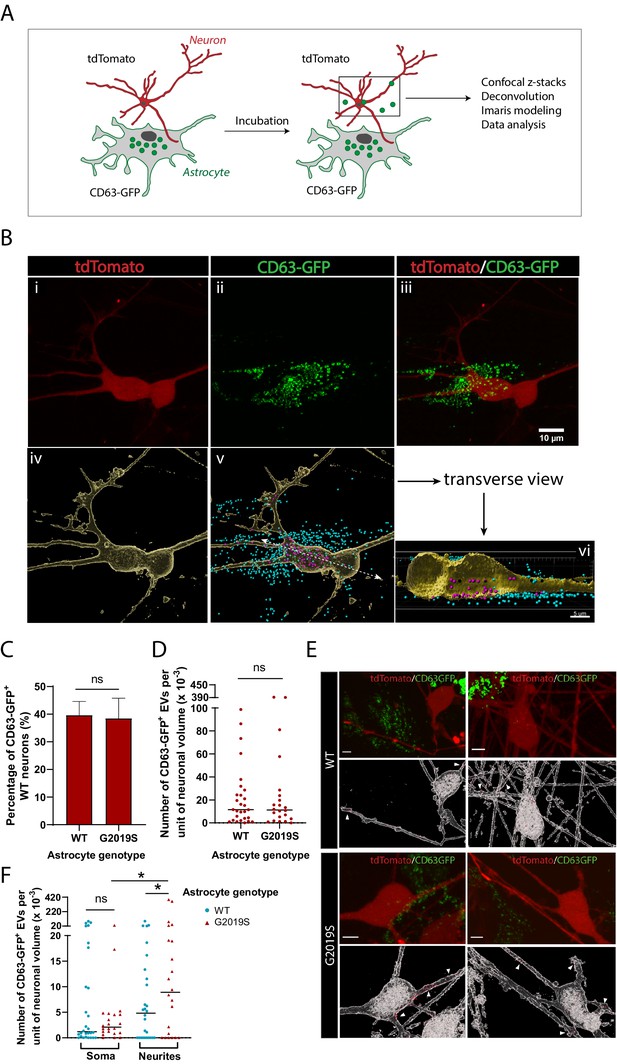
Dopaminergic neurons internalize exosomes secreted by WT and LRRK2 G2019S astrocytes.
(A) WT dopaminergic neurons were transduced with rh10-CAG-tdTomato (red), and WT or LRRK2 G2019S astrocytes were transduced with lenti-CD63-GFP (green) to produce green-labeled exosomes. Neurons and astrocytes were co-cultured, and uptake of CD63-GFP exosomes by neurons was monitored by live-cell confocal microscopy, followed by deconvolution and Imaris modeling. (B) Confocal images of tdTomato neurons (Bi), CD63-GFP astrocytes (Bii), and the merged image (Biii). The corresponding Imaris software rendering represents tdTomato neurons in yellow (Biv), and the CD63-GFP exosomes in blue (outside the neurons) or purple (inside the neurons) (Bv). A transverse view of a neuron shows purple-labeled exosomes inside the somas and neurites (Bvi). (C) Quantification of the percentage of neurons with internalized WT or LRRK2 G2019S CD63-GFP exosomes at the time of live-cell imaging. (D) Quantification of the number of CD63-GFP exosomes per unit of neuronal volume. (E) Confocal images of tdTomato neurons co-cultured with WT or G2019S CD63-GFP isogenic astrocytes, and the corresponding Imaris software rendering representing neurons in white and CD63-GFP exosomes in red. White arrowheads show exosomes inside neurites. Scale bar: 5 µm. (F) Quantification of the number of CD63-GFP exosomes per unit of soma or neurite volume. For all datasets: data are from three independent biological replicates, ≥80 neurons were analyzed for each experimental condition. The scatter plot shows the median value. Statistical analysis was performed using two-tailed unpaired Student’s t-test with equal s.d. (C, D), or one-way ANOVA with Newman–Keuls multiple comparisons (E) (ns: not significant, *p≤0.05).
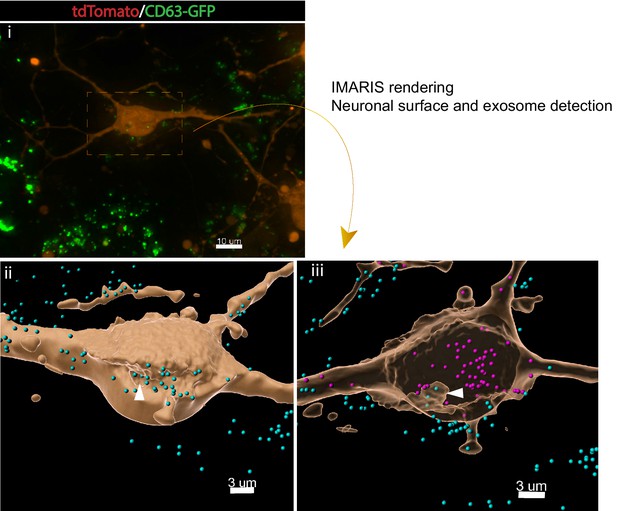
Dopaminergic neurons internalize astrocyte-derived extracellular vesicles (EVs) by endocytosis.
Widefield image of co-cultured tdTomato neurons (red) and CD63-GFP astrocytes (green) (i). The dashed red box shows the neuronal soma, and the corresponding Imaris software rendering represents the tdTomato neuron in yellow (ii, iii), and the CD63-GFP EVs in blue (outside the neurons) or purple (inside the neurons). The white arrowhead shows the internal budding of the neuronal plasma membrane and endocytosis of extracellular CD63+ exosomes.
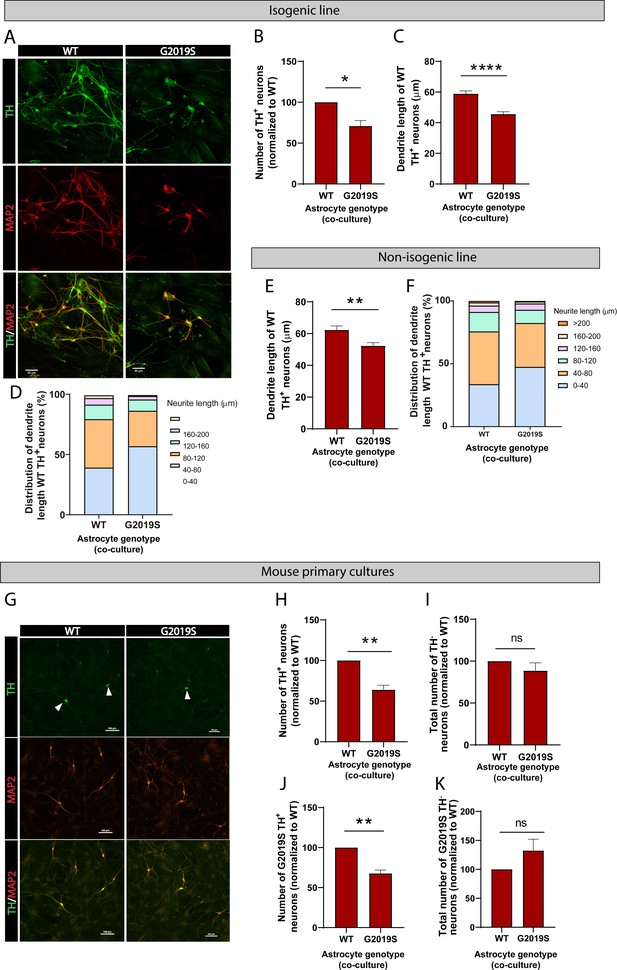
LRRK2 G2019S astrocytes affect the viability and morphology of dopaminergic neurons.
(A) Confocal images showing neurons labeled by immunofluorescence with the pan-neuronal and dendrite marker MAP2 (red), the marker for dopaminergic neurons TH (green), and the merged images (MAP2, red, and TH, green). MAP2: microtubule-associated protein 2; TH: tyrosine hydroxylase. (B–F) Quantification of neuron viability (number of dopaminergic neurons remaining in culture) (B), average dendrite length (C, E), and dendrite length distribution (D, F) after 14 days in culture with WT or LRRK2 G2019S isogenic (B–D) or non-isogenic (E, F) astrocytes. Viability data are from five (B, D) independent biological replicates, and at least 500 neurons were counted per experimental condition and biological replicate. WT and 530 LRRK2 G2019S neurons were counted (B). Dendrite length data are from three independent biological replicates, and more than 300 (E, F) or 500 (C, D) neurites were measured for each experimental condition. (G) Confocal images showing WT mouse primary neurons co-cultured with WT or LRRK2 G2019S mouse primary astrocytes. Neurons are labeled by immunofluorescence and images show MAP2 (red), TH (green), and the merged images (MAP2, red, and TH, green). White arrowheads mark TH+/MAP2+ dopaminergic neurons. (H, I) The cells were scored for TH+/MAP2+ dopaminergic (H) or TH-/MAP2+ non-dopaminergic neuron survival (I) after 14 days co-culture with WT or LRRK2 G2019S astrocytes. (J, K) LRRK2 G2019S mouse primary neurons were co-cultured with mouse WT or LRRK2 G2019S astrocytes as described in (H, I) and scored for relative viability of TH+/MAP2+ dopaminergic (J) or TH-/MAP2+ non-dopaminergic neurons (K). Viability data for all mouse primary culture experiments are from three independent biological replicates, and at least 200 neurons were counted for each experimental condition and biological replicate. For all datasets, error bars represent mean + SEM; statistical analysis was performed using two-tailed unpaired Student’s t-test with equal s.d. (*p≤0.05, **p<0.01, ****p<0.0001). Results shown in panel (B) support similar observations recently documented in a non-isogenic induced pluripotent stem cell (iPSC)-based model system (di Domenico et al., 2019).
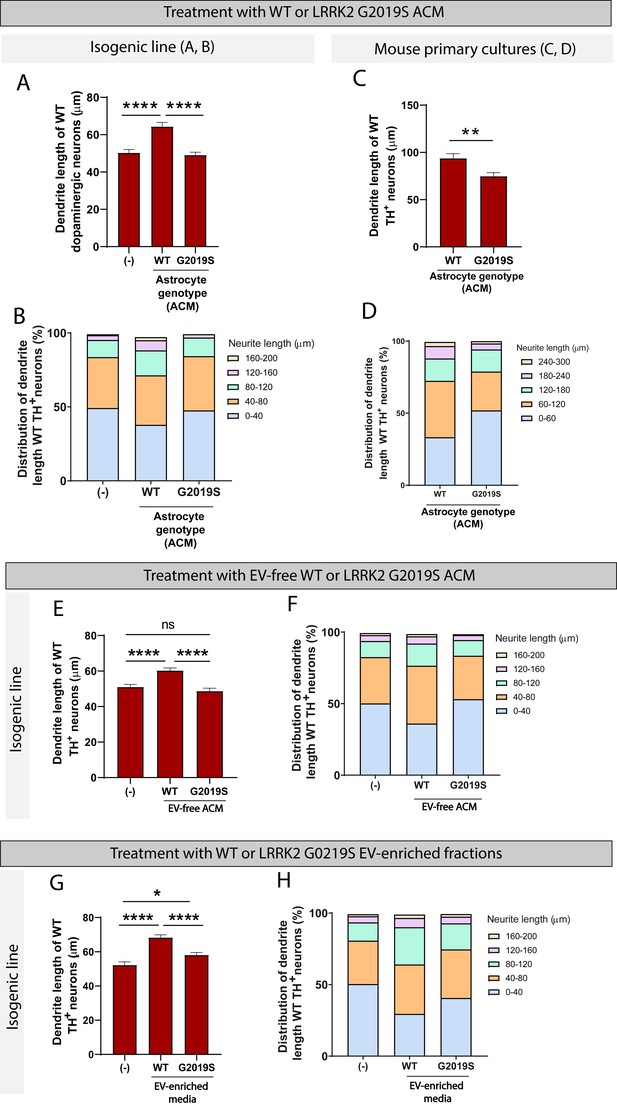
WT but not LRRK2 G2019S astrocytes release neurotrophic factors via two distinct secretory pathways to support the growth of dopaminergic neurons.
(A–D) WT dopaminergic neurons were cultured for 14 days with basal medium (-), WT or LRRK2 G2019S astrocytes conditioned media (ACM), and the resulting dendrite length average (A, C) and distribution (B, D) were quantified. Data in (A, B) were collected using the isogenic induced pluripotent stem cell (iPSC)-based model, data in (C, D) used the primary mouse culture system. The data are from three independent biological replicates, and ≥450 (A, B) or 170 (C, D) neurites were measured per experimental condition. (E, F) Quantification of the average (E) and distribution (F) of dendrite lengths of WT dopaminergic neurons cultured for 14 days with basal medium (-), or WT or LRRK2 G2019S extracellular vesicle (EV)-free ACM. Data are from four independent biological replicates, and ≥650 neurites were measured for each experimental condition. (G, H) Quantification of the average (G) and distribution (H) of dendrite lengths of WT dopaminergic neurons cultured for 14 days with basal medium (-), or WT or LRRK2 G2019S EV-enriched fractions. Data are from three independent biological replicates, and ≥450 dendrite were measured for each experimental condition. For all datasets, error bars represent mean + SEM, and statistical analysis was performed using two-tailed unpaired Student’s t-test with equal s.d. (C) or one-way ANOVA with Tukey’s multiple comparisons correction (A, E, G) (ns: not significant, *p≤0.05, ****p<0.0001).
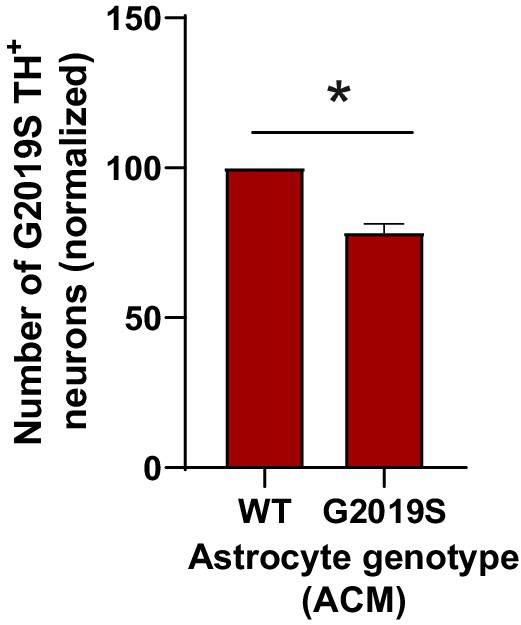
LRRK2 G2019S dopaminergic neurons are sensitive to factors secreted by LRRK2 G2019S astrocytes in a mouse primary culture model.
Quantification of mouse primary neuron viability (number of dopaminergic neurons remaining in culture) after 14 days treatment with astrocyte conditioned media (ACM) prepared from WT or mutant primary astrocytes. Data are from two independent biological replicates; error bars represent mean + SEM; statistical analysis was performed using two-tailed unpaired Student’s t-test with equal s.d.; *p≤0.05.
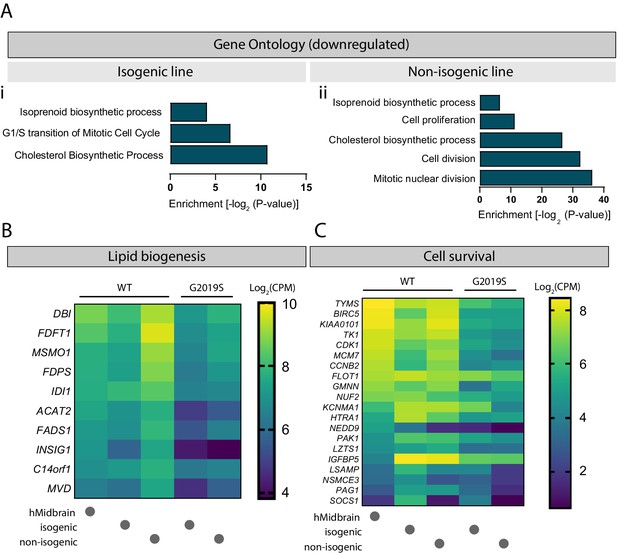
Cholesterol biogenesis is impaired in LRRK2 G2019S astrocytes.
Gene ontology analysis of isogenic (Ai) and non-isogenic (Aii) LRRK2 G2019S vs. WT induced pluripotent stem cell (iPSC)-derived astrocytes showing downregulated components identified by RNA sequencing. Benjamini–Hochberg adjusted p-values were obtained from the Database for Annotation, Visualization and Integrated Discovery (DAVID) tool. (B, C) Heatmaps showing top significantly downregulated genes associated with lipid biogenesis (B) and cell survival (C) in LRRK2 G2019S vs. WT astrocytes using a 0.7-fold threshold and a false discovery rate of 0.05. Data shows log2(CPM) values calculated for WT and LRRK2 G2019S astrocytes prepared using the isogenic or non-isogenic lines, as well as human midbrain fetal astrocytes to use as a reference. Abbreviation: CPM, counts per million.
Tables
Reagent type (species) or resource | Designation | Source or reference | Identifiers | Additional information |
---|---|---|---|---|
Antibody | Alexa Fluor-conjugated secondary antibodies | Thermo Fisher Scientific | Multiple | IF (1:1000) |
Antibody | Anti-alpha-synuclein (rabbit monoclonal) | Abcam | ab138501 | IF (1:200) |
Antibody | Anti-APC magnetic beads | Miltenyi | 130-090-855 | MACS (1:5) |
Antibody | Anti-CD133, APC-conjugated (mouse monoclonal) | BD Biosciences | 566596 | MACS (1:20) |
Antibody | Anti-CD44 (rat monoclonal) | BD Biosciences | 550538 | IF (1:500) |
Antibody | Anti-CD63 (mouse monoclonal) | Thermo Fisher Scientific | MA1-19281 | IF (1:500).IEM: (1:40) |
Antibody | Anti-GFAP (mouse monoclonal) | BD Biosciences | 556328 | IF (1:500) |
Antibody | Anti-LRRK2 (rabbit monoclonal) | Abcam | ab133474 | IF (1:200).IEM (1:20) |
Antibody | Anti-MAP2 (chicken polyclonal) | Thermo Fisher Scientific | PA1-10005 | IF (1:3000) |
Antibody | Anti-mouse IgG conjugated to 12 nm gold particles (goat polyclonal) | Jackson ImmunoResearch | 115-205-146 | IEM (1:20) |
Antibody | Anti-mouse IgG conjugated to 6 nm gold particles (goat polyclonal) | Jackson ImmunoResearch | 115-195-146 | IEM (1:40) |
Antibody | Anti-phospho alpha-synuclein (S129) (rabbit monoclonal) | Abcam | ab51253 | IEM (1:20) |
Antibody | Anti-rabbit IgG conjugated to 6 nm gold particles (goat polyclonal) | Jackson ImmunoResearch | 111-195-144 | IEM (1:40) |
Antibody | Anti-TH (rabbit polyclonal) | PhosphoSolutions | 2025-THRAB | IF (1:500) |
Antibody | Anti-vimentin (rat monoclonal) | R&D | MAB2105 | IF (1:100) |
Cell line (Homo sapiens) | Human midbrain astrocytes | ScienCell | 1850 | |
Cell line (Homo sapiens) | Isogenic control iPSCs | Reinhardt et al., 2013 | IM2-GC | Prof. Dr. Thomas Gasser (Universitätsklinikum Tübingen) and Prof. Dr. Hans R. Schöler (Max-Planck Institute) |
Cell line (Homo sapiens) | LRRK2 G2019S iPSCs | Reinhardt et al., 2013 | IM2 | Prof. Dr. Thomas Gasser (Universitätsklinikum Tübingen) and Prof. Dr. Hans R. Schöler (Max-Planck Institute) |
Cell line (Homo sapiens) | Healthy control iPSCs | RUCDR Infinite Biologics | RUID: NN0004085, line ID ND36091 | Dr. Randall T. Moon (Howard Hughes Medical Institute/University of Washington) |
Cell line (Homo sapiens) | LRRK2 G2019S iPSCs | RUCDR Infinite Biologics | RUID: NN0004061, line ID ND33879 | Dr. Randall T. Moon (Howard Hughes Medical Institute/University of Washington) |
Strain, strain background(Mus musculus) | WT mouse | Taconic | C57BL/6NTac | |
Strain, strain background(Mus musculus) | LRRK2 G2019S constitutive knock-in mouse | Taconic | C57BL/6-Lrrk2tm4.1Arte | |
Chemical compound, drug | Ascorbic acid | Sigma | A92902 | |
Chemical compound, drug | CHIR99021 | STEMCELL Technologies | 72054 | |
Chemical compound, drug | Cytosine β-D-arabinofuranoside (AraC) | Sigma | C1768 | |
Chemical compound, drug | DAPT | Tocris Bioscience | 2634 | |
Chemical compound, drug | Dibutyryl cAMP | Millipore Sigma | 28745 | |
Chemical compound, drug | LDN193189 hydrochloride | Sigma | SML0559 | |
Chemical compound, drug | Purmorphamine | Millipore Sigma | 540223 | |
Chemical compound, drug | ROCK inhibitor (Y-27632) | STEMCELL Technologies | 72304 | |
Chemical compound, drug | SB431542 | STEMCELL Technologies | 72234 | |
Commercial assay or kit | Control library phiX | Illumina | FC-110-3001 | |
Commercial assay or kit | ExoELISA-ULTRA Complete Kit (CD63 Detection) | System Biosciences | EXEL-ULTRA-CD63-1 | |
Commercial assay or kit | ExoGlow -Protein EV Labeling Kit (Red) | System Biosciences | EXOGP100A-1 | |
Commercial assay or kit | KAPA library quantification kit | Roche | 7960298001 | |
Commercial assay or kit | KAPA SYBR FAST qPCR kit | Roche | 7959397001 | |
Commercial assay or kit | Nextera XT DNA library preparation kit | Illumina | FC-131-1024 | |
Commercial assay or kit | RevertAid First Strand cDNA Synthesis Kit | Thermo Fisher Scientific | K1622 | |
Commercial assay or kit | RNA purification kit | Qiagen | 74104 | |
Commercial assay or kit | TaqMan LRRK2 SNP genotyping assay | Thermo Fisher Scientific | 4351379 | |
Commercial assay or kit | TaqMan hPSC Scorecard Panel | Thermo Fisher Scientific | A15871 | |
Other | 96-well plates with optically clear bottom | Ibidi | 89626 | |
Other | LD columns | Miltenyi Biotech | 130-042-901 | |
Other | Polycarbonate aluminum bottles with cap assembly | Beckman Coulter | 355618 | |
Other | Prolong Diamond with DAPI | Thermo Fisher Scientific | P36962 | |
Peptide, recombinant protein | BDNF (recombinant human) | PeproTech | 450-02 | |
Peptide, recombinant protein | EGF (recombinant human) | Thermo Fisher Scientific | PHG0311 | |
Peptide, recombinant protein | FGF basic (146 aa) (recombinant human) | R&D Systems | 233-FB-025 | |
Peptide, recombinant protein | GDNF (recombinant human) | PeproTech | 450-10 | |
Peptide, recombinant protein | SHH(C25II) (recombinant mouse) | R&D Systems | 464-SH-200 | |
Peptide, recombinant protein | TGF-β3 (recombinant human) | R&D Systems | 8420-B3-025 | |
Recombinant DNA reagent | pCT-CD63-GFP | System Biosciences | CYTO120-PA-1 | |
Sequence-based reagent | Human CD82_F | This paper | qPCR primer | 5’GGTTTCGTGGAAGGAAGC3’ |
Sequence-based reagent | Human CD82_R | This paper | qPCR primer | 5’AAGATCAAGTTGAAGAGGAAGAG 3’ |
Sequence-based reagent | Human Rab27b_F | This paper | qPCR primer | 5’AACTGGATGAGCCAACTG3’ |
Sequence-based reagent | Human Rab27b_R | This paper | qPCR primer | 5’CTTGCCGTTCATTGACTTC3’ |
Software, algorithm | Beacon Designer Lite 8.16 | Premier Biosoft (San Francisco, CA) | N/A | |
Software, algorithm | Database for Annotation, Visualization and Integrated Discovery (DAVID) v6.8 | N/A | https://david.ncifcrf.gov | |
Software, algorithm | EBseq v3.8 | Leng et al., 2013 | http://www.bioconductor.org/packages/devel/bioc/html/EBSeq.html | |
Software, algorithm | ComplexHeatmap v3.11 | Gu et al., 2016 | http://bioconductor.org/packages/release/bioc/html/ComplexHeatmap.html | |
Software, algorithm | Imaris 9.3 | Bitplane (Belfast, UK) | N/A | |
Software, algorithm | STAR v.2.7.3a | Dobin et al., 2013 | https://github.com/alexdobin/STAR/releases, Alexander, 2021 | |
Software, algorithm | Starcode v1.1 | Zorita et al., 2015 | https://github.com/gui11aume/starcode, Guillaume, 2021 |
Additional files
-
Supplementary file 1
List of exosome-related genes upregulated in the LRRK2 G2019S astrocytes compared to isogenic controls and identified by RNA-sequencing.
- https://cdn.elifesciences.org/articles/73062/elife-73062-supp1-v2.xlsx
-
Supplementary file 2
List of exosome-related genes upregulated in the LRRK2 G2019S astrocytes compared to non-isogenic controls and identified by RNA-sequencing.
- https://cdn.elifesciences.org/articles/73062/elife-73062-supp2-v2.xlsx
-
Transparent reporting form
- https://cdn.elifesciences.org/articles/73062/elife-73062-transrepform1-v2.docx